Abstract
Duchenne and Becker muscular dystrophy (DMD/BMD) are X-linked recessive disorders caused by mutation in dystrophin gene. We analyzed the results of a genetic test in 29 DMD/BMD patients, their six female relatives, and two myopathic female patients in Korea. As the methods developed, we applied different procedures for dystrophin gene analysis; initially, multiplex polymerase chain reaction was used, followed by multiplex ligation-dependent probe amplification (MLPA). Additionally, we used direct DNA sequencing for some patients who had negative results using the above methods. The overall mutation detection rate was 72.4% (21/29) in DMD/BMD patients, identifying deletions in 58.6% (17/29). Most of the deletions were confined to the central hot spot region between exons 44 and 55 (52.9%, 7/19). The percentage of deletions and duplications revealed by MLPA was 45.5% (5/11) and 27.2% (3/11), respectively. Using the MLPA method, we detected mutations confirming their carrier status in all female relatives and symptomatic female patients. In one patient in whom MLPA revealed a single exon deletion of the dystrophin gene, subsequent DNA sequencing analysis identified a novel nonsense mutation (c.4558G > T; Gln1520X). The MLPA assay is a useful quantitative method for detecting mutation in asymptomatic or symptomatic carriers as well as DMD/BMD patients.
Duchenne muscular dystrophy (DMD; MIM 310200) and Becker muscular dystrophy (BMD; MIM 300376) are allelic X-linked recessive disorders that are caused by mutation of the DMD gene located at Xp21 (1). DMD is the most common muscle disease in children, with a reported incidence of 1:3,500 live born males (2).
The dystrophin gene is the largest of the identified human genes, spanning 2.4 Mb of a genomic sequence and corresponding to about 0.1% of the total human genome (1, 3). The gene contains 79 exons encoding a 14,000 bp messenger RNA transcript that is translated into the protein dystrophin (1, 3). The majority of identified mutations are deletions, accounting for approximately 60%-65% of DMD and 85% of BMD mutations, and duplications have been observed in 5%-15% (1, 3, 4). The remaining cases may be caused by small mutations such as microdeletions, microinsertions, point mutations, or splicing mutations (1, 5).
There are many methods for the detection of dystrophin gene mutations including multiplex polymerase chain reaction (PCR), Southern blotting, quantitative real time PCR, fluorescence in situ hybridization (FISH), and sequencing for point mutations (3, 5-7). Recently, multiplex ligation-dependent probe amplification (MLPA) was developed for quantitative genomic screening of copy numbers variations at specific target sequences (8, 9). However, there have been only a few reports of genetic analysis of the dystrophin gene in Korea.
In this study, we report the genetic analysis of the dystrophin gene and delineate genetic characteristics in DMD/BMD patients, their female carriers, and symptomatic female patients in Korea.
We performed mutation analysis in the dystrophin gene using multiplex PCR or MLPA at Samsung Seoul Hospital (Seoul, Korea) between January 2003 and November 2010. This study included 29 DMD/BMD patients, six asymptomatic female relatives, and two symptomatic female patients. All DMD/BMD patients were younger than 15 yr at the time of diagnosis and were clinically diagnosed based on typical clinical presentation, elevated creatine kinase, and family history. Among the 29 male patients, four patients were clinically diagnosed with BMD who are ambulatory beyond age 12, and the remaining were diagnosed with DMD based on clinical severity and immunohistochemical staining. A retrospective analysis of the medical records was conducted to determine the clinical and laboratory findings.
We applied multiplex PCR to 20 DMD/BMD patients between January 2003 and May 2006 and adopted the MLPA technique for 11 DMD/BMD patients, their six female relatives, and two symptomatic female patients between June 2006 and November 2010.
Genomic DNA was isolated from peripheral blood leukocytes using the Wizard genomic DNA purification kit according to the manufacturer's instructions (Promega, Madison, WI, USA). Multiplex DNA amplification of the dystrophin gene was carried out according to the techniques of Chamberlain et al. (10) and Beggs et al. (11) using two multiplex PCR assays for the amplification of ten primer pairs (exons 3, 4, 6, 8, 12, 13, 17, 19, 43-52, 60, and promoter). The PCR products were separated on 3% agarose gel or 2% nusieve + 1% agarose gel.
The MLPA reaction was performed to screen exons of the dystrophin gene using the SALSA MLPA probe sets P034 and P035 (MRC-Holland, Amsterdam, The Netherlands) according to the manufacturer's instructions (12). The MLPA samples consisted of approximately 200 ng of genomic DNA. Ligation and amplification were carried out on an ABI 9600 Thermal Cycler. The PCR conditions included 33 cycles at 94℃ for 30 sec, 60℃ for 30 sec, and 72℃ for 60 sec, followed by a final extension at 72℃ for 20 min to allow adequate probe hybridization with SALSA probe mix P034 (dystrophin gene exons 1-10, 21-30, 41-50, and 61-70) and P035 (dystrophin gene exons 11-20, 31-40, 51-60, and 71-79). All amplified fragments were separated using capillary electrophoresis on an ABI PRISM 3130 Genetic Analyzer. The area under the peak for each amplified fragment was measured and normalized in comparison with the peak areas of normal control individuals using GeneMarker software v.1.8 (SoftGenetics, State College, PA, USA). As the dystrophin gene is located on the X chromosome, deletions of one or more exons result in total absence of the corresponding MLPA amplification product in male patients and a 30%-55% reduction in female carriers (13). Duplications give an almost two-fold greater relative peak area in male patients and a 30%-55% increase in female carriers.
The MLPA results from male patients were initially assessed visually for the detection of deletion, which was represented as absence of the DMD specific peak. Absence of DMD peaks corresponding to two or more contagious exons was regarded as reliable, and no further investigation were performed. The absence of only one DMD peak in males was further examined by uniplex and multiplex PCR. If a deletion could not be confirmed by PCR, direct sequencing was performed to identify point mutations using a BigDye Terminator Cycle Sequencing Ready Reaction kit (Applied Biosystems) on the ABI 3100 Genetic analyzer (Applied Biosystems).
The mean age of 25 DMD patients at the time of genetic testing was 5.1 ± 2.2 yr (10 months-7.7 yr), and the mean age of symptoms onset was 3.5 ± 2.2 yr (5 months-7.2 yr). The most common presenting symptom was muscle weakness, which was seen in 13 patients (52.0%), followed by incidentally detected elevated hepatic enzymes in nine patients (36.0%). The remaining patients presented with developmental delay (n = 2) and abnormal gait (n = 1). Nine of 25 patients (31.0%) had a family history of muscular dystrophy. Thirteen patients (52.0%) had gross motor developmental delay at the time of diagnosis, and six patients (24.0%) had a language delay. On physical examination, pseudohypertrophy of calf muscles was present in 21 patients (85.0%), and Gowers' sign was observed in 17 patients (68.0%). The mean level of serum creatine kinase (CK) was 14,048 ± 8,925 IU/L (4,605-39,318 IU/L), and the mean levels of liver enzymes aspartate transaminase (AST) and alanine transaminase (ALT) were 298 ± 177 IU/L (98-876 IU/L) and 411 ± 293 IU/L (84-1437 IU/L), respectively. Nine patients underwent a muscle biopsy. Eight patients showed a complete absence of dystrophin on immunohistochemical analysis, while a small amount of the protein was detected in one patient (case 13). The clinical severity of the patient was the same as the other patients; the patient was wheelchair-bound at the age of 10 yr.
In cases of BMD, four patients came to medical attention at a mean age of 9.6 ± 3.0 yr. Mean age at diagnosis was 11.0 ± 3.7 yr. At that time, two patients complained of myalgia and muscle cramps, while the other two patients showed asymptomatic elevated hepatic enzymes. Only one patient had a family of dystrophinoapthy. All patients maintained independent ambulation. Gowers' sign was observed in one patient (case 9). CK levels were lower than in the DMD patients, with average values at diagnosis of 5,132 ± 4,284 IU/L (2,666-11,529 IU/L). The mean levels of AST and ALT were also lower in BMD patients at values of 107 ± 63 IU/L (69-200 IU/L) and 153 ± 34 IU/L (128-203 IU/L), respectively.
Six female relatives of the four DMD patients were included in this study; four relatives were the mothers of affected patients, one was the sister of case 25, and one was the aunt of case 28. Additionally, this study included two symptomatic female carriers (case 34 and 37), aged 6.5 and 7.5 yr at the time of diagnosis. One patient (case 34) had strabismus and mild motor weakness and was scheduled to undergo surgery at an ophthalmology clinic. During the pre-surgical evaluation, an increase in hepatic enzymes (AST/ALT) was found incidentally in that patient, and her serum CK level was 1,471 IU/L (reference: 24-204 IU/L). The muscle biopsy revealed myopathic features, and MLPA showed a duplication of exons 61-77. The other symptomatic carrier (case 37) also had elevated hepatic enzymes and intermittent leg pain. She was found to have pseudohypertrophy of the calf muscle upon physical examination. Her serum CK level was 3,854 IU/L, and the MLPA revealed a deletion of exon 68.
Analysis of mutation in the dystrophin gene was performed with three different methods: multiplex PCR, MLPA, and direct DNA sequencing. In 15 patients (cases 1-20), multiplex PCR was performed to screen for deletions. Twelve patients (cases 1-12) had deletions of the dystrophin gene. Eight patients (cases 13-20) showed a normal pattern in the multiplex PCR. Full direct DNA sequencing of the dystrophin gene was performed for case 17, but the result was normal. MLPA analysis was performed in 11 patients (cases 19-29), including two patients with a normal multiplex PCR pattern (cases 19, 20). Six patients (cases 20-22, 24-26) had deletions of the dystrophin gene, and duplications were detected in three patients (cases 23, 28, 29, Table 1, Fig. 1). Seven patients (cases 13-19) who did not show any mutation on genetic studies were diagnosed by muscle biopsy and immunohistochemical analysis.
Two brothers (cases 11, 21) and their mother (case 30) were included in this study. While the elder brother (case 11) had deletions of exons 32-44 according to multiplex PCR, the younger brother (case 21) and their mother (case 30) had deletions of exons 18-44, shown with the MLPA method (Fig. 2). In the other five female relatives (cases 31-33, 35, 36), the deletions or duplications were identical to that previously detected in the related male (Table 2). MLPA analysis was also able to detect deletions or duplications in two female myopathic patients (Table 2).
MLPA analysis revealed a single exon deletion of the dystrophin gene in one patient (case 20), in whom conventional multiplex PCR analysis did not detect any deletions. We noted the absence of a peak corresponding to exon 33 and performed PCR amplification of the exon with a primer set designed for sequencing in order to confirm the deletion of the exon. PCR amplification revealed a product of the correct size, indicating that the exon was present. However, sequencing analysis revealed a nonsense mutation (c.4559G > T;Glu1520X) in the gene corresponding to the last 3' nucleotide of the left MLPA probe (Fig. 3, 4). The altered nucleotide was located precisely at the ligation site of the half-probes, sufficiently disrupting the ligation to appear as a deletion.
In this study, we used multiplex PCR from January 2003 to May 2006 in 20 DMD/BMD patients and the MLPA technique from June 2006 to November 2010 in 11 patients, their six female relatives, and two female myopathic patients to identify exonic rearrangements in the dystrophin gene. Twenty deletions and six duplications were identified (Tables 1, 2).
Deletions in male patients can be easily detected using multiplex PCR, because two multiplex sets covering mutation hotspot regions could detect about 98% of all deletions (10, 11). However, the MLPA assay analyzes all 79 exons, thus detecting all exon scale deletions and larger size of deletions than those previously determined by multiplex PCR (13). Interestingly, we found different sizes of deletions in one family. The multiplex PCR revealed deletions of exons 32-44 in the older brother before the utilization of MLPA. The MLPA method revealed that the younger brother and the mother (cases 21, 30) had a longer deletion (exons 18-44) after three years. Hwa et al. (14) reported that none of the deletions found by multiplex PCR was missed by MLPA, and MLPA increased the deletion detection rate by 5.7%. Although this is not a direct comparison, the MLPA may allow for more accurate determination of deletion size.
Among all 29 DMD/BMD patients, 17 (58.6%) were found to have exon deletions. With MLPA, the rate of deletions was 45.5% (5/11), which is lower than that estimated in previous reports, based mainly on European or American data (1, 3, 9, 15). In addition, the duplication rate was relatively higher (27.3%, 3/11). The deletion rate and the duplication rate were reported as 36.0% and 24.7%, respectively, in Taiwanese patients (14). Another study revealed a similar deletions rate of 34.3% in local Chinese patients (16). Those studies hypothesized that the discrepancies of frequencies of deletions and duplications compared to previous reports may have been due to the ethnic or regional difference (14-16). Although our result is similar to those results, it is limited for generalization by small sample size and should be further confirmed in larger studies.
Deletions and duplications can happen almost anywhere in the dystrophin gene. However, one location towards the central part of the gene (exons 44-55) and the other site towards the 5' end (exons 2-20) have been previously reported as the two hotspot regions (1, 3, 11). The 200-kb region covering intron 44, exon 45, and intron 45 is known as the major deletion breakpoint region of the gene (3). In this study, most of deletions were confined to the central hotspot region between exons 44 and 55 (n = 9, 52.9% of deletions), followed by exons 2-20 (n = 3, 17.6% of deletions). Those data are in agreement with other studies (1, 3, 15). In particular, in our BMD patients, deletions were arranged between exons 45 and 52, and three patients had deletions between exons 45-48. According to previous reports, distal rod deletions involving exons 45 to 48 result in a relatively high level of dystrophin and a mild BMD phenotype, while proximal deletions tend to associate with a low level of dystrophin and a more severe phenotype (17, 18). In this study, two patients had only mild myalgia, while the other one patient was asymptomatic, with increased CK levels. All three patients maintained independent ambulation ability and normal muscle strength beyond age 13.
Since adopting the MLPA technique, we could identify mutation of the dystrophin gene in six female relatives and two female myopathic patients. The previous performed multiplex PCR approach is not useful for detecting female carriers, because the deletions of dystrophin gene are masked by the amplification of the normal X chromosome. However, MLPA, a new method involving quantitative analysis of all exons, allows for carrier detection and can provide valuable information for genetic counseling and prenatal diagnosis. In all female relatives, MLPA provided evidence of the same dystrophin gene mutations as those observed in the affected, related males. For one female carrier (case 36), we carried out prenatal diagnosis using amniotic fluid cells and confirmed that the fetus did not have any deletions or duplications in the dystrophin gene. The female carrier confidently continued with her pregnancy. Recently, Wu et al. (19) reported that MLPA is a very promising prenatal diagnosis technique for congenital genetic diseases combined with short tandem repeat analysis (STR) genotyping and linkage analysis. Using STR genotyping, the genomic DNA of the fetus was identified not to be contaminated by his mother's DNA, because amniotic fluid cells are easily contaminated by blood cells from the mother. In addition, X-linkage analysis showed that only the X chromosome of the fetus comes from his mother. MLPA analysis disclosed mutations in dystrophin genes in our two myopathic female patients. Two-thirds of the mothers of affected males are thought to be female carriers, and approximately 8% of female carriers suffer from some degree of muscle weakness (8, 20, 21). Serum CK levels are elevated in approximately 45%-70% of carriers, including those in our study, and the measurement of serum CK is the most commonly used initial screening method for detecting carriers (21, 22). Although muscular weakness was not prominent in our cases, one female (case 37) presented with intermittent myalgia, predominantly in the leg, and pseudohypertrophy of the calf muscle. Using MLPA analysis, we could confidently identify carrier individuals in female myopathic patients who had no family history of dystrophinopathies. However, familial genetic analysis will provide the exact genetic information for other family members and for genetic counseling.
In the present study, we found a deletion of a single exon caused by point mutations in the oligo-binding domains. False-positive MLPA results may be due to the presence of polymorphisms obstructing probe annealing, or they can occur if a sequence variation coincides with the probe binding regions and ligation site (13, 23, 24). Therefore, a confirmatory test by PCR or direct sequencing is necessary for cases with a single exon deletion detected by MLPA (13, 23). In the present study, we confirmed that the apparent exon 33 deletion observed in one patient (case 20) was caused by a novel nonsense mutation (c.4558G > T;Gln1520X) in the oligonucleotide hybridization region through direct DNA sequencing analysis.
For those patients with no exon deletions or duplications, full sequence analysis is the robust method for detecting small mutations (25). However, in our study, one patient (case 17) showed no mutations using direct DNA sequencing of the dystrophin gene and was diagnosed via muscle biopsy. Some mutations remain unidentified, and complex rearrangements, which are thought to be pure intronic rearrangements or changes in the regulatory regions, are impossible to precisely define using routine molecular diagnostic techniques (3, 26).
Recently, a novel, custom high-density comparative genomic hybridization array (CGH), which analyzes copy number variation across the entire dystrophin gene, was designed (26-28). That method has the added advantages of detecting complex rearrangements and large scale intronic alterations, thereby offering a slightly higher mutation detection rate than MLPA and other exon-based tests. However, it is apparent from the majority of genetic studies that all mutations cannot be identified with standard molecular analysis. In those cases, a muscle biopsy may be helpful for protein studies and muscle RNA analysis to establish an accurate diagnosis.
In this study, the overall mutation detection rate was 72.4% in DMD/BMD patients. The percentage of deletions in the dystrophin gene was lower, while the duplication rate was higher than data for a Caucasian population. However, our study should be further confirmed in future studies due to the small sample size. Since the time MLPA was introduced, it is possible to identify female carriers as well as deletions or duplications in the dystrophin gene, as we have demonstrated the mutations in six female relatives and two female myopathic patients. Our recommendation is that genetic study for dystrophin gene analysis should begin with quantitative screening via MLPA, followed by full sequence analysis from genomic DNA. The MLPA-based array analysis system, recently developed as a simple, rapid and automated system, provides high-resolution and speed (29, 30). Therefore, we expect MLPA analysis to be enhanced by future technological improvements to further increase mutation detection and reduce cost.
Figures and Tables
Fig. 1
Duplications of exons 3-7 of the dystrophin gene in one affected patient (case 23). (A) Electrophoregrams after MLPA in a control patient, (B) Electrophoregrams after MLPA in case 23.
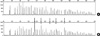
Fig. 2
Deletions of exons 18-44 of the dystrophin gene in one affected patient (case 21) and his mother (case 30). These electrophoregrams show the MLPA sample after hybridization with SALSA probe mix P034 (dystrophin gene exons 1-10, 21-30, 41-50, and 61-70). The deletions of exons 18-20 and 31-40 were detected after probe hybridization with P035 (dystrophin gene exons 11-20, 31-40, 51-60, and 71-79), which is not shown. (A) Control, (B) Female carrier, (C) Case 21.
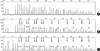
Fig. 3
The results of single exon PCR (A) and electrophoregrams after MLPA analysis (B) of exon 33 in the dystrophin gene of case 20. (A) Single exon PCR result. Lane 1 is from a control sample, and the band of lane 2 corresponds with exon 33 of case 20. (B) The arrow indicates absence of a peak corresponding to exon 33 in MLPA analysis.
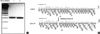
Fig. 4
Direct DNA sequencing of exon 33. The arrow indicates the hemizygous G to T transversion at nucleotide 4558 in exon 33 (c.4558G > T;Glu1520X) of the dystrophin gene of case 20. (A) Control, (B) Case 20.
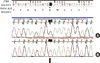
Table 1
Exon deletions and duplications detected by multiplex PCR and MLPA assay in DMD/BMD patients
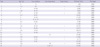
*Case 11 and case 21 are brothers; †Means the age of symptom onset; ‡The remark of + means that the patient has a family history for DMD or BMD; §Plasma creatine kinase (CK) levels were measured in all patients at onset. CK, creatine kinase; BMD, Becker muscular dystrophy; DMD, Duchenne muscular dystrophy.
References
1. Muntoni F, Torelli S, Ferlini A. Dystrophin and mutations: one gene, several proteins, multiple phenotypes. Lancet Neurol. 2003. 2:731–740.
2. Emery AE. Population frequencies of inherited neuromuscular diseases: a world survey. Neuromuscul Disord. 1991. 1:19–29.
3. Prior TW, Bridgeman SJ. Experience and strategy for the molecular testing of Duchenne muscular dystrophy. J Mol Diagn. 2005. 7:317–326.
4. Hu XY, Ray PN, Murphy EG, Thompson MW, Worton RG. Duplicational mutation at the Duchenne muscular dystrophy locus: its frequency, distribution, origin, and phenotypegenotype correlation. Am J Hum Genet. 1990. 46:682–695.
5. Roberts RG, Bobrow M, Bentley DR. Point mutations in the dystrophin gene. Proc Natl Acad Sci U S A. 1992. 89:2331–2335.
6. Traverso M, Malnati M, Minetti C, Regis S, Tedeschi S, Pedemonte M, Bruno C, Biassoni R, Zara F. Multiplex real-time PCR for detection of deletions and duplications in dystrophin gene. Biochem Biophys Res Commun. 2006. 339:145–150.
7. Yau SC, Bobrow M, Mathew CG, Abbs SJ. Accurate diagnosis of carriers of deletions and duplications in Duchenne/Becker muscular dystrophy by fluorescent dosage analysis. J Med Genet. 1996. 33:550–558.
8. Gatta V, Scarciolla O, Gaspari AR, Palka C, De Angelis MV, Di Muzio A, Guanciali-Franchi P, Calabrese G, Uncini A, Stuppia L. Identification of deletions and duplications of the DMD gene in affected males and carrier females by multiple ligation probe amplification (MLPA). Hum Genet. 2005. 117:92–98.
9. Lalic T, Vossen RH, Coffa J, Schouten JP, Guc-Scekic M, Radivojevic D, Djurisic M, Breuning MH, White SJ, den Dunnen JT. Deletion and duplication screening in the DMD gene using MLPA. Eur J Hum Genet. 2005. 13:1231–1234.
10. Chamberlain JS, Gibbs RA, Ranier JE, Nguyen PN, Caskey CT. Deletion screening of the Duchenne muscular dystrophy locus via multiplex DNA amplification. Nucleic Acids Res. 1988. 16:11141–11156.
11. Beggs AH, Koenig M, Boyce FM, Kunkel LM. Detection of 98% of DMD/BMD gene deletions by polymerase chain reaction. Hum Genet. 1990. 86:45–48.
12. Schouten JP, McElgunn CJ, Waaijer R, Zwijnenburg D, Diepvens F, Pals G. Relative quantification of 40 nucleic acid sequences by multiplex ligation-dependent probe amplification. Nucleic Acids Res. 2002. 30:e57.
13. Lai KK, Lo IF, Tong TM, Cheng LY, Lam ST. Detecting exon deletions and duplications of the DMD gene using Multiplex Ligation-dependent Probe Amplification (MLPA). Clin Biochem. 2006. 39:367–372.
14. Hwa HL, Chang YY, Chen CH, Kao YS, Jong YJ, Chao MC, Ko TM. Multiplex ligation-dependent probe amplification identification of deletions and duplications of the Duchenne muscular dystrophy gene in Taiwanese subjects. J Formos Med Assoc. 2007. 106:339–346.
15. Wang X, Wang Z, Yan M, Huang S, Chen TJ, Zhong N. Similarity of DMD gene deletion and duplication in the Chinese patients compared to global populations. Behav Brain Funct. 2008. 4:20.
16. Lo IF, Lai KK, Tong TM, Lam ST. A different spectrum of DMD gene mutations in local Chinese patients with Duchenne/Becker muscular dystrophy. Chin Med J (Engl). 2006. 119:1079–1087.
17. Beggs AH, Hoffman EP, Snyder JR, Arahata K, Specht L, Shapiro F, Angelini C, Sugita H, Kunkel LM. Exploring the molecular basis for variability among patients with Becker muscular dystrophy: dystrophin gene and protein studies. Am J Hum Genet. 1991. 49:54–67.
18. Magri F, Govoni A, D'Angelo MG, Del Bo R, Ghezzi S, Sandra G, Turconi AC, Sciacco M, Ciscato P, Bordoni A, Tedeschi S, Fortunato F, Lucchini V, Bonato S, Lamperti C, Coviello D, Torrente Y, Corti S, Moggio M, Bresolin N, Comi GP. Genotype and phenotype characterization in a large dystrophinopathic cohort with extended follow-up. J Neurol. 2011. 258:1610–1623.
19. Wu Y, Yin G, Fu K, Wu D, Zhai Q, Du H, Huang Z, Niu Y. Gene diagnosis for nine Chinese patients with DMD/BMD by multiplex ligation-dependent probe amplification and prenatal diagnosis for one of them. J Clin Lab Anal. 2009. 23:380–386.
20. Hoffman EP, Arahata K, Minetti C, Bonilla E, Rowland LP. Dystrophinopathy in isolated cases of myopathy in females. Neurology. 1992. 42:967–975.
21. Song TJ, Lee KA, Kang SW, Cho H, Choi YC. Three cases of manifesting female carriers in patients with Duchenne muscular dystrophy. Yonsei Med J. 2011. 52:192–195.
22. Griggs RC, Mendell JR, Brooke MH, Fenichel GM, Miller JP, Province M, Moxley RT 3rd, Huntzinger D, Vaughn A, Cohen M. Clinical investigation in Duchenne dystrophy: V. use of creatine kinase and pyruvate kinase in carrier detection. Muscle Nerve. 1985. 8:60–67.
23. Janssen B, Hartmann C, Scholz V, Jauch A, Zschocke J. MLPA analysis for the detection of deletions, duplications and complex rearrangements in the dystrophin gene: potential and pitfalls. Neurogenetics. 2005. 6:29–35.
24. Sellner LN, Taylor GR. MLPA and MAPH: new techniques for detection of gene deletions. Hum Mutat. 2004. 23:413–419.
25. Hwa HL, Chang YY, Huang CH, Chen CH, Kao YS, Jong YJ, Chao MC, Ko TM. Small mutations of the DMD gene in Taiwanese families. J Formos Med Assoc. 2008. 107:463–469.
26. Bovolenta M, Neri M, Fini S, Fabris M, Trabanelli C, Venturoli A, Martoni E, Bassi E, Spitali P, Brioschi S, Falzarano MS, Rimessi P, Ciccone R, Ashton E, McCauley J, Yau S, Abbs S, Muntoni F, Merlini L, Gualandi F, Ferlini A. A novel custom high density-comparative genomic hybridization array detects common rearrangements as well as deep intronic mutations in dystrophinopathies. BMC Genomics. 2008. 9:572.
27. Laing NG, Davis MR, Bayley K, Fletcher S, Wilton SD. Molecular diagnosis of duchenne muscular dystrophy: past, present and future in relation to implementing therapies. Clin Biochem Rev. 2011. 32:129–134.
28. del Gaudio D, Yang Y, Boggs BA, Schmitt ES, Lee JA, Sahoo T, Pham HT, Wiszniewska J, Chinault AC, Beaudet AL, Eng CM. Molecular diagnosis of Duchenne/Becker muscular dystrophy: enhanced detection of dystrophin gene rearrangements by oligonucleotide array-comparative genomic hybridization. Hum Mutat. 2008. 29:1100–1107.
29. Hegde MR, Chin EL, Mulle JG, Okou DT, Warren ST, Zwick ME. Microarray-based mutation detection in the dystrophin gene. Hum Mutat. 2008. 29:1091–1099.
30. Zeng F, Ren ZR, Huang SZ, Kalf M, Mommersteeg M, Smit M, White S, Jin CL, Xu M, Zhou DW, Yan JB, Chen MJ, van Beuningen R, Huang SZ, den Dunnen J, Zeng YT, Wu Y. Array-MLPA: comprehensive detection of deletions and duplications and its application to DMD patients. Hum Mutat. 2008. 29:190–197.