Abstract
Multiplex reverse transcription polymerase chain reaction (mRT-PCR) has recently emerged as an alternative to cytogenetics. We designed and used simplified mRT-PCR system as a molecular screen for acute leukemia. Fifteen fusion transcripts were included: BCR-ABL1, PML-RARA, ZBTB16-RARA, RUNX1-RUNX1T1, CBFB-MYH11, DEK-NUP214, TCF3-PBX1, ETV6-RUNX1, MLL-AFF1, MLL-MLLT4, MLL-MLLT3, MLL-MLLT10, MLL-ELL, MLL-MLLT1, and MLL-MLLT6. A total of 121 diagnostic acute leukemia specimens were studied, comparing the mRT-PCR system with standard cytogenetics. Fifty-six cases (46.3%) had fusion transcripts revealed by our mRT-PCR assay. The concordance rate between mRT-PCR and cytogenetics was 91.7%. However, false negative results were found in three cases who have inv(16), t(4;11) or t(11;19)(q23;p13.1), respectively. Seven cryptic translocations including ETV6-RUNX1, MLL-MLLT3, MLL-MLLT4, and PML-RARA were detected. This mRT-PCR assay is a useful screening tool in acute leukemia because it provides rapid and reliable detection of clinically important chimeric transcripts. In addition, cryptic translocations provide additional genetic information that could be clinically useful.
Conventional cytogenetic findings have repeatedly been shown to be the most powerful prognostic factors for both acute myeloid leukemia (AML) and acute lymphoblastic leukemia (ALL) (1). However, detection of prognostically important balanced translocations is now universally accepted in routine clinical practice as a useful tool in the diagnosis and risk stratification of acute leukemias. In addition, detection of these translocations is valuable for the choice of different treatment approaches (2-6). Balanced translocations can be assayed by a variety of methods. The standard method is the classical G-banding technique, but it is labor-intensive, operator-dependent, time-consuming, and sample quality-dependent. Furthermore, cryptic translocations, which cannot be detected by conventional G-banding technique may occur even when material and techniques are optimal (7-16). Therefore, molecular techniques such as polymerase chain reaction (PCR) and fluorescence in situ hybridization (FISH) have emerged as alternative methods for evaluating prognostically important translocations.
The presence of a large number of clinically relevant translocations in acute leukemia has led to the development of the multiplex reverse transcription-PCR (mRT-PCR) assay, which is capable of simultaneously detecting fusion transcripts. An mRT-PCR kit that enables the detection of 28 different translocations simultaneously has recently become commercially available (HemaVision, Bio-Rad Laboratories, Hercules, CA, USA), thereby rapidly providing a broad information on patients with acute leukemia (17-23). In our view, however, there are two drawbacks to this method. First, too many fusion transcripts are included and some transcripts occur very rarely in clinical specimens. Although this feature guarantees comprehensive screening of fusion transcripts, it may limit the clinical usefulness of the method. Second, because a nested PCR technique is used, two sequential PCR steps are required for each parallel reaction. Moreover, if a band is present, split-out PCR is needed to confirm the specificity of the band. For the above reasons, we sought to apply a more simplified method, both in terms of the number of fusion transcripts and the test procedure, for the detection of balanced translocations in acute leukemia. In the present study, our main aim was to design and use a simplified mRT-PCR assay for detecting prognostically important fusion transcripts in patients with acute leukemia. The second aim was to compare the results of our mRT-PCR assay with those obtained using conventional cytogenetics.
This study included a total of 121 patients who were diagnosed with acute leukemia from November 2006 to July 2007 at the Asan Medical Center. The surplus bone marrow aspirates stored at -70℃ immediately after collection were used in this study. Peripheral blood was used in a subset of patients who had a very high number of leukocytes with predominant blasts. Bone marrow or blood samples were collected into EDTA tubes.
Total RNA was extracted from all samples using a High Pure RNA Isolation kit (Roche, Mannheim, Germany) according to the manufacturer's instructions. The quality and quantity of extracted RNA was measured using a Nanodrop spectrophotometer (Nanodrop Technologies, Wilmington, DE, USA). Complementary DNA (cDNA) was synthesized from 1 µg of total RNA using oligo dT primers and a RevertAid first strand cDNA synthesis kit (Fermentas, Burlington, Canada). Three microliters of synthesized cDNA was used as a template for PCR amplification.
Sixteen pairs of double-priming oligonucleotide (DPO) primers were designed to amplify the unique DNA sequences of leukemia translocation genes. The DPO system tends to prevent formation of secondary structure and effectively eliminates non-specific priming (24). The human hypoxanthine phosphoribosyltransferase 1 (HPRT1) gene was employed as an internal control. The following target genes (and accession numbers) were amplified: BCR-ABL1 (NM_021574/NM_005157), RUNX1-RUNX1T1 (NM_001001890/NM_175636), PML-RARA (XM_001132060/NM_000964), ZBTB16-RARA (NM_00600/NM_000964), CBFB-MYH11 (NM_001755/NM_002474), DEK-NUP214 (NM_003472/NM_005085), TCF3-PBX1 (NM_003200/NM_002585), ETV6-RUNX1 (NM_001987/NM_001754), MLL-AFF1 (NM_005933/NM_005935), MLL-MLLT4 (NM_005933/XM_001132155), MLL-MLLT3 (NM_005933/NM_004529), MLL-MLLT10 (NM_005933/NM_001009569), MLL-MLLT6 (NM_005933/NM_005937), MLL-MLLT1 (NM_005933/NM_005932), MLL-ELL (NM_005933/NM_006532), and HPRT1 (NM_000194). All primers were synthesized by the same company (Bioneer Corp., Daejeon, Korea).
The optimized multiplex PCR was performed in 20-µL reaction mixtures containing cDNA template, 5 × primer mixture containing internal control primer (final concentration, 5 pM of each primer), 2 × master mix (SolGent Co., Daejeon, Korea), and 30 µg/mL of 8-methoxypsoralen (Sigma-Aldrich, St. Louis, MO, USA). 8-Methoxypsoralen was added to reduce the chances of carry-over contamination that often occurs during the PCR process. The amplifications were carried out in an Applied Biosystems 9700 thermal cycler (Perkin-Elmer, Boston, MA, USA) with the following parameters: an initial denaturation step of 94℃ for 15 min; 37 cycles of 94℃ for 0.5 min (denaturation), 63℃ for 1.5 min (annealing), and 72℃ for 1.5 min (extension); and a final extension step of 72℃ for 10 min. As a negative control, sterile deionized water was used as the PCR template instead of nucleic acid. PCR products were detected by electrophoresis of 5 µL of each amplification mixture in 2% agarose gels containing ethidium bromide in 0.5 × Tris-Acetate-EDTA buffers. After PCR, reactants were exposed to 320-400 nm ultraviolet light for 20-30 min to prevent PCR amplicons from being reused as PCR templates.
Detection of 11 different fusion transcripts (RUNX1-RUNX1T1, BCR-ABL1, PML-RARA, ZBTB16-RARA, CBFB-MYH11, DEK-NUP214, MLL-MLLT4, MLL-MLLT3, MLL-MLLT10, MLL-MLLT6, and MLL-ELL) was tested in AML patients, whereas detection of 5 different transcripts (BCR-ABL1, TCF3-PBX1, ETV6-RUNX1, MLL-AFF1, and MLL-MLLT1) was tested in ALL patients. Five reaction tubes were used in AML patients: tube 1, t(8;21) and t(9;22); tube 2, t(15;17); tube 3, various 11q23 rearrangements; tube 4, t(6;9) and t(11;17); and tube 5, inv(16) or t(16;16). Three reaction tubes were used in ALL patients: tube 1, t(9;22) and t(1;19); tube 2, t(11;19) and t(12;21); and tube 3, t(4;11). All AML tubes and ALL tube 3 were used for mixed phenotype acute leukemia (MPAL) patients. Flowchart showing the different steps involved in the detection of fusion transcripts is shown in Fig. 1. The specificity of product was determined from the specific band size. For example, the band size of major BCR-ABL1 fusion gene is 476 bp (b3a2 isoform) or 401 bp (b2a2). On the other hand, the band size of minor BCR-ABL1 e1a2 is 308 bp (Fig. 2).
Amplified PCR products for each specific type were purified using a GEL-SV DNA Purification System (General Biosystem, Seoul, Korea), according to the manufacturer's instructions, and eluted with 20 µL of distilled water. The purified products were inserted into the pCR2.1-TOPO plasmid vector (Invitrogen, Carlsbad, CA, USA) as described by the manufacturer. Plasmid DNA was extracted using an AccuPrep Plasmid Extraction Kit (Bioneer Corp.). The standard control plasmid was confirmed by sequencing. Ten-fold serial dilutions of the recombinant plasmid (1011-103 copies/mL) were used to establish the assay's sensitivity. Each 20-µL reaction mixture contained 10 µL of 2 × PCR master mix (SolGent Co.), 1 µL of DNA sample, 3 µL of 8-methoxypsoralen solution (0.2 mg/mL), 2 µL of deionized water, and 4 µL of primer mixture. PCR was performed using the conditions described above, and amplification was assessed by electrophoresis on 2% agarose gels and ethidium bromide staining. The lowest template copy number amplified was considered the assay's sensitivity.
Bone marrow specimens were cultured and harvested for cytogenetic analysis according to established methods. Chromosome slides were analyzed by G-banding. Chromosomal aberrations are described according to the guidelines of the International System for Human Cytogenetic Nomenclature. Karyotype results served to validate the accuracy of our mRT-PCR system and rule out false positives.
The study group included 66 males and 55 females; 32 were children (median age, 9 yr; range 1-16 yr) and 89 were adults (median age, 47 yr; range 18-79). Of the 121 patients, 77 were diagnosed with AML, 37 with ALL, and 7 with MPAL. A total of 117 patients were diagnosed as de novo and four were diagnosed as secondary AML. The preceding diseases of secondary AML patients were myelodysplastic syndrome (n=3) and chronic myeloid leukemia (n=1). According to the French-American-British classification, 42.9% (33/77) of AML patients were in M2; 14.3% (11/77) each were in M1 and M4; 10.4% (8/77) were in M3; 6.5% (5/77) were in M5; and 11.7% (9/77) were in other types. Among ALL patients, L1 and L2 frequencies were 70.3% (26/37) and 29.7% (11/37), respectively.
Sensitivity assays were performed for CBFB-MYH11, MLL-AFF1, MLL-MLLT4, MLL-MLLT3, MLL-ELL, and TCF3-PBX1. The sensitivity was 1×10-2 copies/mL for all transcripts tested (data not shown).
The mRT-PCR analysis detected a fusion transcript in a total of 56 of 121 (46.3%) patients with acute leukemia (Table 1 and Fig. 2). Among AML patients, the frequency of positive transcripts was 44.2% (34/77). RUNX1-RUNX1T1 was detected in fourteen patients, PML-RARA in eight, MLL-MLLT4 in four, CBFB-MYH11 and MLL-MLLT3 in three each, and DEK-NUP214, BCR-ABL1, and MLL-ELL in one patient each. One patient had both PML-RARA and BCR-ABL1. Forty-three AML patients were negative for fusion transcripts. Among ALL patients, the frequency of positive transcripts was 45.9% (17/37). BCR-ABL1 was detected in seven patients, ETV6-RUNX1 in four, MLL-AFF1 in three, TCF3-PBX1 in two, and MLL-MLLT3 in a patient. Because MLL-MLLT3 was not included in reactions for ALL, the presence of MLL-MLLT3 was confirmed using reaction tube 3 for AML after t(9;11) was unexpectedly identified in conventional cytogenetics. We found that 20 ALL patients were negative for fusion transcripts. Among patients with MPAL, the frequency of positive transcripts was 71.4% (5/7), all of which were BCR-ABL1.
We compared the molecular screening results in 120 patients for whom cytogenetic data were available (Table 2). In one patient with AML, metaphase cells were inadequate for analysis. The overall concordance between conventional karyotyping and mRT-PCR assay was 91.7% (110/130).
The two analyses yielded the same results in 69 of 76 AML patients, for a concordance rate of 90.8%. The mRT-PCR assay revealed specific aberrations in seven patients with normal karyotypes or other chromosomal abnormalities not included in the mRT-PCR. These aberrations included two cases of MLL-MLLT3, one case each of MLL-MLLT4 and PML-RARA. On the other hand, mRT-PCR did not detect chromosomal abnormalities in a small subset of patients. One AML patient each with inv(16), t(4;11), and t(11;19)(q23;p13.1) was not detected by mRT-PCR assay, despite apparent cytogenetic evidence for the corresponding abnormalities. Molecular alterations in these three patients were proved by FISH analyses using dual color, break apart rearrangement probe. Fig. 3 illustrated an example of the FISH analysis performed on one of the three cases. Among patients with ALL, the two analyses yielded matched results in 34 of 37 patients (concordance rate, 92.0%). The mRT-PCR revealed specific aberrations in three patients with normal karyotypes or other chromosomal abnormalities not included in the mRT-PCR assay. These alterations included three cases of ETV6-RUNX1. There were no cases in which mRT-PCR failed to detect the corresponding cytogenetic aberrations in ALL patients. The concordance among patients with MPAL was 100%. Overall, the frequency of cryptic translocations was 5.8% (7/120). Cryptic translocations in this study included t(9;11), t(6;11), t(15;17), and t(12;21) (Table 2). The overall false-negative rate of mRT-PCR was 2.5% (3/120).
For a comparative analysis, a part of patient samples were also tested on the HemaVision kit. Twenty-seven samples included 12 cases with fusion gene positivity (2 with RUNX1-RUNX1T1, 2 with ETV6-RUNX1, 2 with MLL-AFF1, and one patient each with BCR-ABL1 b2a2, BCR-ABL1 e1a2, CBFB-MYH11, MLL-ELL, PML-RARA bcr3 and PML-RARA bcr1), and 15 cases with fusion gene negativity. All the HemaVision results were in agreement with those of our mRT-PCR assay.
The mRT-PCR method used in this study was simplified from the commercially available HemaVision assay in two ways. First, the number of fusion transcripts examined was reduced. Previous studies applying the HemaVision kit have shown that some transcripts, notably MLL-AFX, MLL-EPS15, MLL-MLLT6, ETV6-ABL1, ETV6-PDGFRB, ETV6-MN1, NPM1-RARA, and NPM1-MLF1, have been never detected in clinical samples, whereas transcripts, such as MLL-MLLT11, TCF3-HLF, RUNX1-EVI1 and FUS-ERG, are detected very rarely (17-23). In contrast to the HemaVision kit, which covers 28 different translocations, the mRT-PCR assay kit we used included 15 different translocations that are relatively common and prognostically relevant. Thus, we argue that this simplification did not significantly impair the clinical usefulness of the assay in terms of diagnosis or risk stratification of acute leukemia. Second, the processing method was further simplified by avoiding nested PCR and split-out PCR steps. In addition to shortening the turn-around time of the assay, this simplification eliminated the manipulation of post-amplification products and reduced the risk of serious contamination. In our view, this is a prime advantage of the simplified mRT PCR assay compared with the HemaVision kit.
Investigations of patients with acute leukemia using HemaVision have reported that the frequency of genetic alterations is 15.9%-37.8% (19, 21-23). The mRT-PCR assay used in this study detected fusion transcript in 46.3% of patients, which is a remarkably high frequency compared to those of previous studies. Considering that the full HemaVision kit can detect more chromosomal alterations, it seems contradictory that our assay detected a higher frequency of abnormalities. The most likely reason for this is the high incidence of RUNX1-RUNX1T1 and MLL rearrangement in AML patients. The incidences of these rearrangements in our study (18.2% and 10.4%, respectively) are definitely higher than previously reported frequencies (4%-8% and 4%-7%, respectively) (1). The frequency of the RUNX1-RUNX1T1 transcript recorded in our study is very similar to that reported in previous Korean studies (18.1%-22.5%) (25, 26). Geographic differences could help account for the higher frequency of RUNX1-RUNX1T1 in our study. According to previous studies using HemaVision, the incidence of MLL rearrangement was 6.2% (7/113) in Korean AML patients (23), but only 2.6% (6/233) among Danish AML patients (19). These observations are consistent with the known higher incidence of t(8;21) (the cytogenetic presentation of the RUNX1-RUNX1T1 fusion transcript) among Asians compared to Western populations (27). The incidence of MLL rearrangement in this study is high. Cryptic translocation involving 11q23 can also partly explain the high incidence of MLL rearrangement in our study, because it is a representative difficult-to-detect translocation using conventional cytogenetics.
Our mRT-PCR system detected a few cryptic translocations, including t(12;21), t(15;17), t(9;11), and t(6;11). Their collective frequency was 5.8%, which is similar to that reported in previous studies (2.1%-15.3%) (17-22, 28). These cryptic translocations have been repeatedly reported in the literature, supporting the feasibility of our detection system. Among these abnormalities, cryptic t(15;17) is a noteworthy finding because the identification of t(15;17) remains a diagnostic hallmark of the disease. In rare cases of acute promyelocytic leukemia (APL), submicroscopic insertion of RARA into PML leads to the expression of the PML-RARA transcript. Depending on the size of the insertion, the target can be so small that it does not hybridize with the probe or may hybridize but fail to generate a fluorescent signal large enough to be visualized by FISH (16). No PML-RARA fusion signals were detected by FISH using a dual-color, dual-fusion probe in our patients, although RT-PCR revealed a single PML-RARA chimeric transcript, and morphological examination showed hypergranular characteristics typical of APL. Our finding supports the above-mentioned mechanism of cryptic t(15;17), which is present in 2% of cases (10).
This study has some limitations. First, we could not perform point by point comparison between our mRT-PCR assay and currently widely used HemaVision kit. Comparative analysis was done to only small numbers of patients. Although all of the results of our mRT-PCR assay were in accordance with those of HemaVision kit from a small comparison, there still remains the possibility of discrepant result between two tests. Second, our mRT-PCR assay did not detect each case of inv(16), t(4;11), and t(11;19)(q23;p13.1). Because of the diversity of partner genes and the variations in genetic structures at PCR primer-binding sites, a subset of genetic defects might remain undetected by mRT-PCR analysis, despite apparent cytogenetic presentation. In fact, false-negative results for genetic aberrations, including MLL rearrangements, PML-RARA and CBFB-MYH11, were also reported in previous studies using the HemaVision kit (17, 19, 20, 23). However, an effort for the technical modification will be mandatory to obtain continuous improvement in the diagnostic performance of this mRT-PCR assay.
In recent years, there has been a growing interest in moving beyond cytogenetics to a consideration of subsets of acute leukemia with molecular aberrations that can be particularly useful in further stratifying acute leukemia patients with normal cytogenetics. Whereas some of these genomic aberrations portend a poor prognosis (e.g., FLT3 ITD, overexpression of WT1, BAALC, ERG and EV11), others are associated with a favorable outcome (e.g., NPM1 and CEPBA). Therefore, mRT-PCR assays capable of detecting both molecular aberrations and classical balanced translocations should be a very powerful tool for risk stratification and treatment decisions in acute leukemia. Development and clinical application of these mRT-PCR assays deserve further study.
In conclusion, we believe that the mRT-PCR assay described in this study is a useful screening tool in acute leukemia because it provides rapid and reliable detection of clinically important oncogene chimeric transcripts. Cryptic translocations detected by mRT-PCR provided additional genetic information that could be clinically useful. Although mRT-PCR cannot totally replace conventional cytogenetics, it will serve not only as an auxiliary diagnostic test but also as a platform for risk stratification of acute leukemia. Further applications of simultaneous detection of balanced translocations and molecular aberrations using mRT-PCR assays can be expected in the near future.
Figures and Tables
Fig. 1
Flow diagram illustrating the multiplex RT-PCR assay used in this study. (A) Agarose gel with all negative PCR results. (B) Agarose gel with RUNX1-RUNX1T1-positive PCR (591-bp band) in lane 1. Note that there is no need for nested PCR or split-out PCR to determine the specificity of translocation. (C) Agarose gel with a positive band in lane 3. A single PCR analysis was performed using the individual primer sets specific for MLL-ELL (519-bp band) after identification of the positive band in AML reaction tube 3.
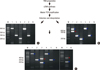
Fig. 2
Examples of fusion transcripts detected by multiplex RT-PCR. Round pointers indicated internal control bands and arrowheads indicated positive PCR bands. (A) BCR-ABL1 b2a2 (401-bp band in lane 1). (B) CBFB-MYH11 isoform A (223-bp band in lane 5). (C) DEK-NUP214 (547-bp band in lane 4). (D) BCR-ABL1 e1a2 (308-bp band in lane 1). (E) ETV6-RUNX1 1B (398-bp band in lane 2). M, 100-bp ladder; N, distilled water (negative control).
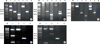
Fig. 3
Fluorescence in situ hybridization with dual color, break apart rearrangement probe on the interphase cells from the patient with inv(16)(p13.1q22) who did not show CBFB-MYH11 fusion gene in the mRT-PCR assay. The green and red signals are separated. It implies the CBFB gene rearrangement.
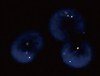
Table 1
Summary of aberrations in patients with acute leukemia detected by multiplex RT-PCR and conventional cytogenetics
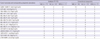
References
1. Mrozek K, Heerema NA, Bloomfield CD. Cytogenetics in acute leukemia. Blood Rev. 2004. 18:115–136.
2. Borkhardt A, Cazzaniga G, Viehmann S, Valsecchi MG, Ludwig WD, Burci L, Mangioni S, Schrapppe M, Riehm H, Lampert F, et al. Associazione Italiana Ematologia Oncologia Pediatrica and the Berlin-Frankfurt-Münster Study Group. Incidence and clinical relevance of TEL/AML1 fusion genes in children with acute lymphoblastic leukemia enrolled in the German and Italian multicenter therapy trials. Blood. 1997. 90:571–577.
3. Pieters R, Schrappe M, De Lorenzo P, Hann I, De Rossi G, Felice M, Hovi L, LeBlanc T, Szczepanski T, Ferster A, et al. A treatment protocol for infants younger than 1 year with acute lymphoblastic leukaemia (Interfant-99): an observational study and a multicentre randomised trial. Lancet. 2007. 370:240–250.
4. Dombret H, Preudhomme C, Boissel N. Core binding factor acute myeloid leukemia (CBF-AML): is high-dose Ara-C (HDAC) consolidation as effective as you think? Curr Opin Hematol. 2009. 16:92–97.
5. Yanada M, Ohno R, Naoe T. Recent advances in the treatment of Philadelphia chromosome-positive acute lymphoblastic leukemia. Int J Hematol. 2009. 89:3–13.
6. Thomas X, Raffoux E, Renneville A, Pautas C, de Botton S, Terre C, Gardin C, Hayette S, Preudhomme C, Dombret H. Which AML subsets benefit from leukemic cell priming during chemotherapy? Long-term analysis of the ALFA-9802 GM-CSF study. Cancer. 2010. 116:1725–1732.
7. Hunger SP, Devaraj PE, Foroni L, Secker-Walker LM, Cleary ML. Two types of genomic rearrangements create alternative E2A-HLF fusion proteins in t(17;19)-ALL. Blood. 1994. 83:2970–2977.
8. Uphoff CC, MacLeod RA, Denkmann SA, Golub TR, Borkhardt A, Janssen JW, Drexler HG. Occurrence of TEL-AML1 fusion resulting from (12;21) translocation in human early B-lineage leukemia cell lines. Leukemia. 1997. 11:441–447.
9. Uckun FM, Herman-Hatten K, Crotty ML, Sensel MG, Sather HN, Tuel-Ahlgren L, Sarquis MB, Bostrom B, Nachman JB, Steinherz PG, et al. Clinical significance of MLL-AF4 fusion transcript expression in the absence of a cytogenetically detectable t(4;11)(q21;q23) chromosomal translocation. Blood. 1998. 92:810–821.
10. Grimwade D, Biondi A, Mozziconacci MJ, Hagemeijer A, Berger R, Neat M, Howe K, Dastugue N, Jansen J, Radford-Weiss I, et al. Characterization of acute promyelocytic leukemia cases lacking the classic t(15;17): results of the European Working Party. Blood. 2000. 96:1297–1308.
11. O'Reilly J, Chipper L, Springall F, Herrmann R. A unique structural abnormality of chromosome 16 resulting in a CBF beta-MYH11 fusion transcript in a patient with acute myeloid leukemia, FAB M4. Cancer Genet Cytogenet. 2000. 121:52–55.
12. Rowe D, Cotterill SJ, Ross FM, Bunyan DJ, Vickers SJ, Byron J, McMullan DJ, Griffiths MJ, Reilly JT, Vandenberghe EA, et al. Cytogenetically cryptic AML1-ETO and CBF beta-MYH11 gene rearrangements: incidence in 412 cases of acute myeloid leukemia. Br J Haematol. 2000. 111:1051–1056.
13. von Bergh A, Gargallo P, De Prijck B, Vranckx H, Marschalek R, Larripa I, Kluin P, Schuuring E, Hagemeijer A. Cryptic t(4;11) encoding MLL-AF4 due to insertion of 5' MLL sequences in chromosome 4. Leukemia. 2001. 15:595–600.
14. Brown J, Jawad M, Twigg SR, Saracoglu K, Sauerbrey A, Thomas AE, Elis R, Harbott J, Kearney L. A cryptic t(5;11)(q35;p15.5) in 2 children with acute myeloid leukemia with apparently normal karyotypes, identified by a multiplex fluorescence in situ hybridization telomere assay. Blood. 2002. 99:2526–2531.
15. Helias C, Leymarie V, Entz-Werle N, Falkenrodt A, Eyer D, Costa JA, Cherif D, Lutz P, Lessard M. Translocation t(5;14)(q35;q32) in three cases of childhood T cell acute lymphoblastic leukemia: a new recurring and cryptic abnormality. Leukemia. 2002. 16:7–12.
16. Han JY, Kim KE, Kim KH, Park JI, Kim JS. Identification of PML-RARA rearrangement by RT-PCR and sequencing in an acute promyelocytic leukemia without t(15;17) on G-banding and FISH. Leuk Res. 2007. 31:239–243.
17. Strehl S, König M, Mann G, Haas OA. Multiplex reverse transcriptase-polymerase chain reaction screening in childhood acute myeloblastic leukemia. Blood. 2001. 97:805–808.
18. Elia L, Mancini M, Moleti L, Meloni G, Buffolino S, Krampera M, De Rossi G, Foa R, Cimino G. A multiplex reverse transcription-polymerase chain reaction strategy for the diagnostic molecular screening of chimeric genes: a clinical evaluation on 170 patients with acute lymphoblastic leukemia. Haematologica. 2003. 88:275–279.
19. Olesen LH, Clausen N, Dimitrijevic A, Kerndrup G, Kjeldsen E, Hokland P. Prospective application of a multiplex reverse transcription-polymerase chain reaction assay for the detection of balanced translocation in leukaemia: a single-laboratory study of 390 paediatric and adult patients. Br J Haematol. 2004. 127:59–66.
20. Hutchings Hoffmann M, Wirenfeldt Klausen T, Hasle H, Schmiegelow K, Brondum-Nielsen K, Johnsen HE. Multiplex reverse transcription polymerase chain reaction screening in acute myeloid leukemia detects cytogenetically unrevealed abnormalities of prognostic significance. Haematologica. 2005. 90:984–986.
21. Preiss BS, Kerndrup GB, Pedersen RK, Hasle H, Pallisgaard N. Contribution of multiparameter genetic analysis to the detection of genetic alterations in hematologic neoplasia. An evaluation of combining G-band analysis, spectral karyotyping, and multiplex reverse-transcription polymerase chain reaction (multiplex RT-PCR). Cancer Genet Cytogenet. 2006. 165:1–8.
22. Meyer-Monard S, Parlier V, Passweg J, Muhlematter D, Hess U, Bargetzi M, Kuhne T, Cabrol C, Gratwohl A, Jotterand M, et al. Combination of broad molecular screening and cytogenetic analysis for genetic risk assignment and diagnosis in patients with acute leukemia. Leukemia. 2006. 20:247–253.
23. Park JS, Yi JW, Jeong SH, Lee HW, Kang SY, Choi JH, Kim HC, Park JE, Kim E, Lim YA, et al. Comparison of multiplex reverse transcription polymerase chain reaction and conventional cytogenetics as a diagnostic strategy for acute leukemia. Int J Lab Hematol. 2008. 30:513–518.
24. Chun JY, Kim KJ, Hwang IT, Kim YJ, Lee DH, Lee IK, Kim JK. Dual priming oligonucleotide system for the multiplex detection of respiratory viruses and SNP genotyping of CYP2C19 gene. Nucleic Acids Res. 2007. 35:e40.
25. Kim YS, Kim GS, Lee CH, Choi SI, Rhang DW, Cho HC. The cytogenetic study of acute and chronic leukemic patients in Korea. Korean J Clin Pathol. 1997. 17:898–911.
26. Ha JS, Ryoo NH, Jeon DS, Kim JR, Kim YJ. The cytogenetic analysis of leukemic patients; a study of 515 cases. Korean J Hematol. 2003. 38:8–14.
27. Johansson B, Mertens F, Mitelman F. Geographic heterogeneity of neoplasia-associated chromosome aberrations. Genes Chromosomes Cancer. 1991. 3:1–7.
28. King RL, Naghashpour M, Watt CD, Morrissette JJ, Bagg A. A comparative analysis of molecular genetic and conventional cytogenetic detection of diagnostically important translocations in more than 400 cases of acute leukemia, highlighting the frequency of false-negative conventional cytogenetics. Am J Clin Pathol. 2011. 135:921–928.