Abstract
This study was conducted to investigate the effects of erythropoietin (Epo) on both acute and chronic limb ischemia (ALI and CLI) and to evaluate the differences in mechanisms according to the method of Epo administration. Hindlimb ischemia was made in BALB/c mice with femoral artery ligation. The mice were divided into four groups: Group 1 (control, no treatment), Group 2 (ALI, early multiple doses), Group 3 (ALI, early single high dose), Group 4 (CLI, late multiple doses). Blood flow ratio significantly increased in Group 2 in 4 weeks. Expression of pAkt and Erythropoietin receptor were significantly higher in Group 2 on postoperative day (POD) 7. The number of CD31- and vascular endothelial growth factor-positive cells were significantly higher in Group 2 on POD 7 and 56. Group 3 and 4 showed a tendency of higher cell counts than the control. The early sustained Epo was effective in improving blood flow through angiogenesis. In chronic phase, weekly multiple dosing of Epo induced angiogenesis, however, the blood flow ratio did not increase significantly. The results of this study suggest that Epo administration during the acute phase followed by maintenance for several days may be important for increasing blood flow and angiogenesis.
Acute and chronic lower extremity ischemia (ALI and CLI) is one of the major diseases that affect quality of life. With increased life expectancy and prevalence of chronic diseases, the number of patients with such ischemia has increased. The mortality rate of acute limb ischemia requiring amputation has also increased (1). In chronic limb ischemia, pain at rest and ulcers increase as the disease progresses, and the annual mortality rate was reported to be up to 20% (2). However, bypass surgery can be performed on only 50% of the patients. The other patients are unable to undergo operation because of comorbidities or paucity of appropriate vessels to be revascularized (3). Unfortunately, currently, there is not any medication for patients who cannot undergo surgical treatment or endovascular intervention.
Erythropoietin (Epo) is a 165 amino-acid glycoprotein hormone with a molecular weight of 30 kDa, which is commonly used to treat anemia. The primary role of Epo is to stimulate the formation of red blood cells and to promote differentiation. Recently, it has been found that hypoxia induces the expression of the Epo gene (4). This phenomenon has led us to believe that Epo is related to induce angiogenesis, apoptosis inhibition and the direct or indirect protection of ischemic tissue.
In general, angiogenesis occurs through the formation of new blood vessels by endothelial progenitor cells (EPCs) and by sprouting from the existing vessels (5). Erythropoietin receptors (EpoRs) and EPCs are known as substances involved in the mechanism of angiogenesis (6, 7). However, the mechanisms have not yet been well elucidated. In particular, studies on EPCs have led to varying results. The mechanisms may vary depending on the administration methods (8).
This study was conducted to investigate the effects of Epo on both acute and chronic limb ischemia and to evaluate the differences in mechanisms according to the method of Epo administration.
We used 7-week-old BALB/c mice which were purchased from Central Lab (Seoul, Korea). In this research institute, the mice were bred for 7 weeks with no dietary restrictions for adaptation. They were all males, weighing 24-27 g. In weeks 1 and 8, they were sacrificed, and underwent Western blot analysis and flow cytometry and immunohistochemistry. All procedures were conducted after approval of the institutional animal care and use committee of Seoul National University Hospital (SNUHIACUC, No 09-0225).
For general anesthesia, Zoletil® (Virbac lab, Carros, France) 100 mL/kg and xylazine 25 mg/kg were administered intraperitoneally space. A 2-cm left vertical skin incision was then made. After the identification of the femoral vessels, the superficial femoral artery was ligated including the inferior epigastric artery, with the preservation of the femoral nerve. After the surgery, mice were randomly assigned to 4 groups: Group 1 (n = 9, controls, no treatment), Group 2 (n = 9, acute limb ischemia [ALI] model with injection of 1,000 U/kg Epo for 4 days after surgery), Group 3 (n = 9, ALI model with injection of 5,000 U/kg Epo once on the day of surgery), Group 4 (n = 6, chronic limb ischemia [CLI] model, injection of 5,000 U/kg Epo once a week for 4 weeks after postoperative day [POD] 28) (Fig. 1). Epo (Recormon®, 1,000 U/0.3 mL, JW Pharmaceuticals, Seoul, Korea) was injected into the ischemic thigh several times using a 0.1 mL syringe.
To provide functional evidence for Epo in angiogenesis, blood flow was measured in both legs on POD 3 and once every week for 8 weeks using laser Doppler (Moor Instruments, Devon, UK). After each animal was anesthetized with ketamine, its fur was removed using a depilatory cream from the limb before imaging. Mice were placed on a heating plate to minimize temperature variation. Color coded images were recorded, and the average perfusions of the ischemic and nonischemic limb were calculated on the basis of colored histogram pixels (Laser Doppler imager windows software version 3.08, Devon, UK). The calculated perfusion was expressed as a ratio of ischemic to nonischemic limb.
Western blot was performed to elucidate the mechanisms of Epo. After the muscle was harvested from the ischemic thigh at weeks 1 and 8, it was stored at -80℃ using dry ice. Homogeneous tissue was extracted using a Radio-Immunoprecipitation Assay (RIPA) buffer. The protein from the ischemic leg was reacted with EpoR (1:1,000; Santa Cruz, Heidelberg, Germany), serine/threonine protein kinase (pAkt) (1:1,000; Cell Signaling Technology, Danvers, MA, USA) and eNOS (1:1,000; Cell Signaling Technology) antibodies. The difference between individual plates was corrected by using β-actin. Concentrations were quantified by using the Image J program (NIH, Bethesda, MD, USA).
The intensities of fluorescence-stainted CD34 and CD133 were measured 1 and 8 weeks after the surgery in order to evaluate the role of endothelial progenitor cells (EPCs). A blood sample was taken from the abdominal aorta and then stored in test tubes containing EDTA solution. After cells were separated, plasma was collected in 15 mL conical tubes. After dissolution and centrifugation of red blood cells the supernatant liquid was removed and phosphate buffer saline (PBS) washing was performed 2 or 3 times. CD34 antibody (BD pharmingen, San Diego, CA, USA) and CD133 antibody (eBioscience, San Diego, CA, USA) were added in each tube. The intensities of CD34 and CD133 were measured using a flow cytometer (Becton Dickinson, Mountain View, CA, USA). All processes were performed on the day of surgery.
The muscle taken from the ischemic thigh was kept formalinfixed and paraffin embedded. Each muscle specimen was cut into 4 µm sections and stained with CD31 (Abcam, Cambridge, UK) and vascular endothelial growth factor (VEGF) (AbDserotec, Kidlington, UK) in order to measure the density of blood vessels. A single pathologist counted stained cells under high power field (HPF) (× 400 magnification) at 20 randomly selected sites.
The results in each group are expressed as mean ± SD. The statistical significance of the results was analyzed using the Kruskal-Wallis test and Mann-Whitney test. Statistical significance was accepted if P value was less than 0.05. SPSS version 17.0 (SPSS Inc, Chicago, IL, USA) was used for all statistical analyses.
Immediately after surgery, ischemia was induced in the all mice without any significant difference in LDPI between the 4 groups (P = 0.207). All groups showed an increased ratio of LDPI for 4 weeks and thereafter remained constant. In Group 2, the ratio of LDPI was particularly increased from week 2 and showed a statistically significant increase in week 4 (P = 0.048) (Fig. 2). The LDPI ratio of Group 3 tended to become lower, though it seemed to increase at POD 21. Group 4 treated with Epo from week 4 showed no significant difference from Group 1 at the end of the study (P = 0.629).
The thigh muscle was stained for CD31, a marker of arterioles, in week 1. Stained spots were randomly counted at 20 locations under HFP. CD31 was significantly higher in Groups 2 and 3 than in Group 1. In addition, VEGF staining in Groups 2 and 3 were also significantly higher than in Group 1 (P = 0.011) (Fig. 3). Additionally, both CD31 and VEGF were more significantly increased in Group 2 than in Group 3 (P = 0.018 and P = 0.02, respectively). This results showed fractionated Epo injection increased angiogenesis more effectively compared with high dose single administration.
In week 8, CD31 and VEGF stains were significantly more increased in Groups 2, 3 and 4 than in Group 1 (P = 0.007, P = 0.007, respectively).
Epo, as shown in Groups 2 and 3, increased the density of blood vessels, which lasted for 8 weeks. In the chronic phase, Epo was also effective in angiogenesis.
If there was a reaction with EPCs antibody CD34 or CD133 through flow cytometry, the reaction was considered positive. There were no significant differences between the four groups in week 1 (P = 0.654) (Fig. 4). Also, all of the groups, including Group 4 showed no significant difference in week 8. So this result means that EPCs are not likely to be involved in the angiogenesis by local injection of Epo regardless of acute or chronic limb ischemia.
Western blot was performed to determine whether pAkt and EpoR were expressed in weeks 1 and 8. In week 1, the expressions of pAkt and EpoR were significantly higher in Group 2 (P = 0.037, P = 0.017, respectively) (Fig. 5). The expression of pAkt and EpoR tended to be higher in Group 3 than in Group 1, but this difference was significance (P = 0.83). In week 8, Groups 3 and 4 showed an increasing tendency for the expressions of pAkt and EpoR, which was not statistically significant (P = 0.610, P = 0.70).
Since EpoR was found in the brain, retina, heart, kidney, smooth muscle, myoblasts and endothelial cells, which are non-erythroid cells, Epo has been expected to play a non-hematopoietic role in damaged tissues (9-15). Experiments on the non-hematopoietic effects of Epo are underway in the field of cardiology and neuroscience. Ralf Gäbel et al. (16) reported that administration of Epo (3,000 U/kg) decreased the infarction range by 45% and increased the capillary by 17% after the induction of myocardial infarction. In the field of neuroscience, Epo has been reported to significantly improve neurologic function by inhibiting the demyelination and infiltration of inflammatory cells (17, 18). While research on the non-hematopoietic functions of Epo is active in the cardiology and neuroscience fields, experiments concerning its effects in limb ischemia are limited. For this reason, a 5-fold or higher dose of Epo is needed to confirm the non-hematopoietic effect in an animal model of limb ischemia due to an affinity difference. The affinity of non-hematopoietic EpoR for Epo is 50-fold less than that of hematopoietic EpoR (19). In other words, the EpoR responsible for non-hematopoiesis has different structures from hematopoietic EpoR. Hematopoietic EpoR consists of a pair of the same receptors (EpoR2), whereas non-hematopoietic EpoR is composed of one EpoR and one beta-common receptor (βcR) stemming from interleukin-3 or -5 (20). Moreover, it is relatively difficult to exert angiogenetic and anti-inflammatory effects on skeletal muscles because of the wider area. These differences make it difficult to prove the effects of anti-inflammation and angiogenesis on limb ischemia. Therefore, it is important to determine the appropriate drug concentrations and administration times for inducing angiogenesis in ischemia.
In this experiment, 1,000 U/kg of Epo for 3 days increased the blood flow and reduced peripheral necrosis through increased Akt and EpoR. Whereas, a single administration of 5,000 U/kg tended to increase Akt and EpoR, but this did not reach statistical significance, suggesting that a single high-dose of Epo appears to be less effective. Based on these results, it is conceivable that a sustained concentration of Epo may be important as a non-hematopoietic factor in the acute ischemia phase than higher single concentration. This finding is in quite a contrast to the effect in anemia, where a single high-dose of Epo is effective (8).
In general, improved blood flow is a result of angiogenesis by EPCs as well as arteriogenesis by expansion of small arteries (5). It has been reported that Epo induces the release of EPCs from bone marrow in cardiac ischemia. However, in our study, there were no significant differences in the number of EPCs at weeks 1 and 8. Therefore, the local injection of Epo into ischemic areas is thought to act locally, by increasing expression of EpoR and Akt rather than through systemic responses. This result is similar with experiment of Li et al. (21). In addition, several days of continuous treatment with Epo is important for the sufficient expression of Akt.
In chronic limb ischemia, Epo stimulates angiogenesis but does not increase blood flow. Unlike acute injury, antiapoptotic and anti-inflammatory reactions to Epo may be not play an important role in CLI.
This study has an important limitation. The results of initially divided doses for several days in chronic limb ischemia were missing. Further studies are needed to confirm our results.
In conclusion, the initial divided doses of Epo were effective in improving blood flow through angiogenesis and in reducing necrosis in the acute phase of ischemic injury. However, in the chronic phase, weekly injected Epo induced angiogenesis did not increase blood flow. The results of this study suggest that Epo administration during the acute phase followed by maintenance for several days may be important for increasing blood flow and angiogenesis.
Figures and Tables
Fig. 1
Study flowchart. The mice were divided into four groups: Group 1 (control, no treatment), Group 2 (ALI, early multiple doses of Epo), Group 3 (ALI, early single high dose of Epo), Group 4 (CLI, late multiple doses of Epo). Large arrows mean injection of 5,000 U/kg Epo and small arrows 1,000 U/kg Epo. Five mice died before the end of the experiment. Epo, erythropoietin; G1, Group 1; G2, Group 2; G3, Group 3; G4, Group 4; ALI, acute limb ischemia; CLI, chronic limb ischemia; POD, postoperative day.
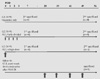
Fig. 2
Representative results of Doppler blood flowmetry. (A) Ischemic/non-ischemic limb blood flow ratio value in mice. Group 2 shows improvement on the blood flow rate than other groups (*P < 0.048) after POD 28. (B) Laser Doppler blood flow (LDBF) pseudocolor image POD 56. Group 2 picture shows intact toes.
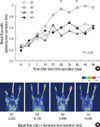
Fig. 3
Measurement of the CD31 and VEGF at POD 7 and POD 56. Number of the CD31 and VEGF positive cell count/HPF are significantly a higher in Group 2 than that of other groups at both POD 7 and POD 56. Group 3, 4 have a higher count of the CD31 and VEGF positive cell count than Group 1 at POD 56; *,†P < 0.05. VEGF, vascular endothelial growth factor; HPF, high power field.
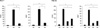
References
1. Campbell WB, Marriott S, Eve R, Mapson E, Sexton S, Thompson JF. Amputation for acute ischaemia is associated with increased comorbidity and higher amputation level. Cardiovasc Surg. 2003. 11:121–123.
2. Ouriel K. Peripheral arterial disease. Lancet. 2001. 358:1257–1264.
3. Adam DJ, Beard JD, Cleveland T, Bell J, Bradbury AW, Forbes JF, Fowkes FG, Gillepsie I, Ruckley CV, Raab G, et al. Bypass versus angioplasty in severe ischaemia of the leg (BASIL): multicentre, randomised controlled trial. Lancet. 2005. 366:1925–1934.
4. Wenger RH. Mammalian oxygen sensing, signalling and gene regulation. J Exp Biol. 2000. 203:1253–1263.
5. Carmeliet P. Angiogenesis in health and disease. Nat Med. 2003. 9:653–660.
6. Wright GL, Hanlon P, Amin K, Steenbergen C, Murphy E, Arcasoy MO. Erythropoietin receptor expression in adult rat cardiomyocytes is associated with an acute cardioprotective effect for recombinant erythropoietin during ischemia-reperfusion injury. FASEB J. 2004. 18:1031–1033.
7. Lipsic E, Schoemaker RG, van der Meer P, Voors AA, van Veldhuisen DJ, van Gilst WH. Protective effects of erythropoietin in cardiac ischemia: from bench to bedside. J Am Coll Cardiol. 2006. 48:2161–2167.
8. Steensma DP, Molina R, Sloan JA, Nikcevich DA, Schaefer PL, Rowland KM Jr, Dentchev T, Novotny PJ, Tschetter LK, Alberts SR, et al. Phase III study of two different dosing schedules of erythropoietin in anemic patients with cancer. J Clin Oncol. 2006. 24:1079–1089.
9. Liu C, Shen K, Liu Z, Noguchi CT. Regulated human erythropoietin receptor expression in mouse brain. J Biol Chem. 1997. 272:32395–32400.
10. Grimm C, Wenzel A, Groszer M, Mayser H, Seeliger M, Samardzija M, Bauer C, Gassmann M, Reme CE. HIF-1-induced erythropoietin in the hypoxic retina protects against light-induced retinal degeneration. Nat Med. 2002. 8:718–724.
11. Wu H, Lee SH, Gao J, Liu X, Iruela-Arispe ML. Inactivation of erythropoietin leads to defects in cardiac morphogenesis. Development. 1999. 126:3597–3605.
12. Westenfelder C, Biddle DL, Baranowski RL. Human, rat, and mouse kidney cells express functional erythropoietin receptors. Kidney Int. 1999. 55:808–820.
13. Ammarguellat F, Gogusev J, Drueke TB. Direct effect of erythropoietin on rat vascular smooth-muscle cell via a putative erythropoietin receptor. Nephrol Dial Transplant. 1996. 11:687–692.
14. Ogilvie M, Yu X, Nicolas-Metral V, Pulido SM, Liu C, Ruegg UT, Noguchi CT. Erythropoietin stimulates proliferation and interferes with differentiation of myoblasts. J Biol Chem. 2000. 275:39754–39761.
15. Anagnostou A, Liu Z, Steiner M, Chin K, Lee ES, Kessimian N, Noguchi CT. Erythropoietin receptor mRNA expression in human endothelial cells. Proc Natl Acad Sci U S A. 1994. 91:3974–3978.
16. Gäbel R, Klopsch C, Furlani D, Yerebakan C, Li W, Ugurlucan M, Ma N, Steinhoff G. Single high-dose intramyocardial administration of erythropoietin promotes early intracardiac proliferation, proves safety and restores cardiac performance after myocardial infarction in rats. Interact Cardiovasc Thorac Surg. 2009. 9:20–25. discussion 5.
17. Agnello D, Bigini P, Villa P, Mennini T, Cerami A, Brines ML, Ghezzi P. Erythropoietin exerts an anti-inflammatory effect on the CNS in a model of experimental autoimmune encephalomyelitis. Brain Res. 2002. 952:128–134.
18. Zhang J, Li Y, Cui Y, Chen J, Lu M, Elias SB, Chopp M. Erythropoietin treatment improves neurological functional recovery in EAE mice. Brain Res. 2005. 1034:34–39.
19. Yamaji R, Okada T, Moriya M, Naito M, Tsuruo T, Miyatake K, Nakano Y. Brain capillary endothelial cells express two forms of erythropoietin receptor mRNA. Eur J Biochem. 1996. 239:494–500.
20. Bianchi R, Buyukakilli B, Brines M, Savino C, Cavaletti G, Oggioni N, Lauria G, Borgna M, Lombardi R, Cimen B, et al. Erythropoietin both protects from and reverses experimental diabetic neuropathy. Proc Natl Acad Sci U S A. 2004. 101:823–828.
21. Li L, Okada H, Takemura G, Esaki M, Kobayashi H, Kanamori H, Kawamura I, Maruyama R, Fujiwara T, Fujiwara H, et al. Sustained release of erythropoietin using biodegradable gelatin hydrogel microspheres persistently improves lower leg ischemia. J Am Coll Cardiol. 2009. 53:2378–2388.