Abstract
Recently, subpopulations of regulatory T (Treg) cells, resting Treg (rTreg) and activated Treg (aTreg), have been discovered. The authors investigated the relationship between the change of Treg, aTreg and rTreg and autoimmune diseases. Treg cells and those subpopulations were analyzed by using the human regulatory T cell staining kit and CD45RA surface marker for 42 rheumatoid arthritis (RA), 13 systemic lupus sclerosis (SLE), 7 Behcet's disease (BD), and 22 healthy controls. The proportion of Treg cells was significantly lower in RA (3.8% ± 1.0%) (P < 0.001) and BD (3.3% ± 0.5%) (P < 0.01) compared to healthy controls (5.0% ± 1.3%). The proportion of aTreg cells was also significantly lower in RA (0.4% ± 0.2%) (P = 0.008) and BD (0.3% ± 0.1%) (P = 0.013) compared to healthy controls (0.6% ± 0.3%). The rTreg cells showed no significant differences. The ratio of aTreg to rTreg was lower in RA patients (0.4% ± 0.2%) than that in healthy controls (0.7% ± 0.4%) (P = 0.002). This study suggests that the decrement of aTreg not rTreg cells contributes the decrement of total Treg cells in peripheral blood of RA and BD autoimmune diseases. Detailed analysis of Treg subpopulations would be more informative than total Treg cells in investigating mechanism of autoimmune disease.
Since human regulatory T (Treg) cells were first identified from the peripheral blood, there have been considerable advances in identifying and typing human Treg cells. Human Treg cells were initially characterized relying on the expression of CD4+CD25high cell surface markers based on the finding that murine CD4+CD25+ T cells are highly suppressive (1). After the forkhead box P3 (FoxP3) was discovered as a "master control gene" for Treg cell development and function, Treg cells were better characterized by the expression of intracellular FoxP3 as well as CD25 (2).
Treg cells play an important role in the maintenance of peripheral immunological self-tolerance through suppression of the activation and/or proliferation of self-reactive T cells (3). Therefore, changes of the proportion of Treg cells in peripheral blood or defect of suppressive function in vitro have been reported in several autoimmune diseases such as multiple sclerosis (MS), type I diabetes (T1D), psoriasis, autoimmune myasthenia gravis, systemic lupus sclerosis (SLE), Behcet's disease (BD), and rheumatoid arthritis (RA) (4-15). Although most of those studies demonstrated significant decrement of Treg cells in autoimmune diseases, their results have been controversial.
Recently, human Treg cells were divided into more detailed subpopulations functionally and/or phenotypically (4, 16). In one study, FoxP3 positive T cells were fractionized into CD4+CD25++CD45RA+ Treg, CD4+CD25+++CD45RA- Treg and cytokine-secreting CD4+CD25++CD45RA- non-suppressive T cells (16). The former two T cell populations were suppressive in vitro, then regarded as two Treg cell subpopulations: resting Treg (rTreg) and activated Treg (aTreg) cells. Indeed, the proportion of these subpopulations showed disease-specific changes. In active SLE, the proportion of aTreg cells was decreased and the proportions of rTreg and non-suppressive Treg cells were relatively increased. In contrast, sarcoidosis patients showed increased aTreg subpopulation (16). However, studies for Treg subpopulations in autoimmune diseases are at an early stage and little data have been reported.
The purpose of this study was first, to investigate whether proportion of Treg cells and its subpopulations, aTreg and rTreg, have changes in SLE, RA, and BD patients compared to healthy controls, and secondly to evaluate whether proportional change of Treg cell subpopulations have informative roles in investigating the autoimmune disease mechanism.
Peripheral blood samples were obtained from 42 RA (31 males and 11 females, age 56.8 ± 12.5 yr), 13 SLE (13 females, age 42.0 ± 16.1 yr) and 7 BD (3 male and 4 female, age 45.6 ± 9.7 yr) and 22 healthy controls (16 male and 6 female, age 53.7 ± 3.4 yr). Each patient was diagnosed according to the 2010 American College of Rheumatology criteria for RA, the 1997 revised American College of Rheumatology criteria for SLE and International Study Group Criteria for BD (17-19).
Peripheral blood samples were obtained freshly in sterile EDTA tubes before analysis. The total white blood cell (WBC) and lymphocyte counts, as well as lymphocyte fraction were detected using an ADVIA2120 automatic blood cell counter (Siemens Healthcare, NY, USA). Mononuclear cells (MNCs) were separated by density gradient centrifugation using Ficoll-Paque (GE Healthcare Life Sciences, Milan, Italy). The isolated MNCs were resuspended in phosphate buffered saline (PBS) at the concentration of 1 × 106 cells/mL. For the analysis of the lymphocyte subset, fluorescein isothiocyanate (FITC) CD3 and phycoerythrin (PE) CD4 (Beckman-Coulter, Marseille, France) were used. For the detection of Treg cells, the Human Regulatory T cell Staining kit (eBioscience, San Diego, CA, USA), including FITC CD4, PE CD25, and phycoerythrin-cyanin 5 (PE-Cy5) FoxP3 (forkhead box P3) were used. The detection method was followed by manufacturer's guide. After gating the lymphocyte population, the CD4+CD25highFoxP3+ population was sequentially gated and analyzed as Treg cells. For the analysis of Treg cell subpopulations, PE anti-hCD45RA, PE-Cy5 CD25 and FITC CD4 (Beckman-Coulter) cell surface markers were used. The CD4+CD25++CD45RA+ and CD4+CD25+++CD45RA- cell populations were gated as rTreg and aTreg cells, respectively (Fig. 1).
Values are presented as mean ± standard deviation (SD). All results were analyzed using SPSS v 19.0 (SPSS, Chicago, IL, USA). Differences in means between each disease group and healthy control or between disease groups were analyzed by independent T-test. A P value < 0.05 was considered statistically significant.
The WBC count was generally higher in the disease groups (6,883.4 ± 2,540.9/µL) than the healthy controls (5,416.8 ± 1,084.8/µL) (P < 0.001). Especially, the WBC count in the RA group (7,126.9 ± 2,653.4/µL) was significantly higher than healthy controls (P < 0.001). The lymphocyte fraction in WBC was significantly lower in the SLE group (22.5% ± 9.9%) than in the healthy controls (32.8% ± 6.8%) (P = 0.001). There was no significant difference between the RA or BD groups and healthy controls. The proportion of CD3+ T cells was similar between disease groups and healthy controls. However the proportion of CD4+ in CD3+ cells (helper T cell fraction) was different. The proportion of CD4+ was lower in the SLE group (48.5% ± 8.5%) than in RA (67.3% ± 10.5%) (P < 0.001), BD (58.2% ± 10.5%) (P = 0.036) or healthy controls (65.9% ± 9.1%) (P < 0.001). When we calculated the absolute count of the CD4+ T cells, the SLE group (466.9 ± 356.9/µL) also showed a lower count than the RA (914.2 ± 458.9/µL) (P = 0.002) or healthy controls (742.6 ± 208.1/µL) (P = 0.007) (Fig. 2).
The proportion of all CD25+ cells in the CD4+ cell populations was similar among the disease groups or healthy controls. However, there was a clear difference in the relative proportion of CD4+CD25highFoxP3+ Treg cells. The proportion of CD4+CD25high FoxP3+ was significantly lower in RA patients (3.8% ± 1.0%) (P < 0.001) and BD patients (3.3% ± 0.5%) (P < 0.001), compared to healthy controls (5.0% ± 1.3%). SLE patients also showed lower proportion of CD4+CD25highFoxP3+ cells (4.6% ± 1.3%) than controls, but it was not statistically significant (Fig. 3A).
The proportion of rTreg was similar among RA, SLE and BD patients and healthy controls (Fig. 3B). However, the proportion of aTreg cells was significantly lower in RA patients (0.4% ± 0.2%) (P = 0.008) and BD patients (0.3% ± 0.1%) (P = 0.013) compared to healthy controls (0.6% ± 0.3%) (Fig. 3C). Comparison of the ratio of aTreg to rTreg cells revealed a significantly decreased ratio in RA patients (0.4% ± 0.2%) (P < 0.002) compared to healthy controls (0.7% ± 0.4%). SLE and BD patients did not show statistically significant changes of the ratio of aTreg to rTreg cells compared to controls (Fig. 3D).
The present study revealed that the proportion of total Treg and aTreg cells is significantly decreased in peripheral blood of RA and BD patients compared to healthy controls. Moreover, the decrement of the relative ratio of aTreg to rTreg was found in RA patient compared to healthy controls.
The Treg cells have been known to play a critical role in preventing autoimmune disease by suppressing self-reactive T cells, then the decrement of Treg proportion or decreased suppressive function has been investigated in several reports for autoimmune patients. However, while many of the previous studies demonstrated a significantly decreased Treg cell population and its negative correlation with clinical activity (5-10), other studies demonstrated either no change or a significant increase of Treg cell proportion or normal suppressive capacity of Treg cells in autoimmune patients (11-15). These conflicting results may be due to differences in detection markers, gating strategies or parameters, methodologically. Even the healthy control's Treg cells showed wide range (0.5%-12%) among previous studies (20). Variable disease activity status also could be another cause, because decrement of Treg cells was more distinct in active disease state than remission state (6, 8). Despite of these variabilities, the proportional decrement or decreased suppressive function of Treg cells in autoimmune disease are generally accepted, recently. We measured Treg cells according to expression of CD4+CD25highFoxP3+ which is the most widely used parameters for detection Treg cells recently. Our data for the proportion of Treg cells was 3.8% ± 1.0% in RA, 4.6% ± 1.3% in SLE, 3.3% ± 0.5% in BD, and 5.0% ± 1.3% in healthy controls. In spite of the small number of patients, RA and BD patients showed significantly low proportion of Treg cells than the healthy controls. However, we could not find significant changes in SLE, and this may be due to the limitation of a small number of SLE patients or variable disease status of activity at study point in our study.
In one recent study, functionally and phenotypically different subpopulations of Treg cells were delineated: CD45RA+ CD25++, CD45RA- CD25++ and CD45RA- CD25+++ populations (16). CD45RA+CD25++ and CD45RA- CD25+++ cells possessed a fully functional FOXP3 gene, hardly secreted cytokines and potently suppressed proliferation; these were designated as rTreg and aTreg cells, respectively. In contrast CD45RA-CD25++ cells were shown to be non-proliferative, capable of secreting cytokines and unable to suppress proliferation in vitro, and were designated as secreting non-suppressive T cells. Interestingly, rTreg cells were able to proliferate upon T cell receptor (TCR) stimulation and acquire aTreg cell phenotype. Then, most of the aTreg cells in peripheral blood were revealed to be derived from recently activated proliferating rTreg cells.
Based on these findings, the decreased proportion of aTreg cells in active SLE, but not in the inactive or remission states, was found (16). Unfortunately, we could not find the significant changes of the proportion of aTreg or rTreg in SLE patients, and this result is supposed to be caused by inclusion of the variable disease states, both active and inactive state. However, there were significant decrements in the proportion of aTreg cells in RA and BD patients accompanying decrement of total Treg cells. Regarding the aTreg to rTreg ratio, RA patients displayed a significantly decreased aTreg to rTreg ratio. Although BD and SLE patients showed no statistically significant differences in aTreg to rTreg ratio, the mean values were also lower than healthy controls in the aTreg to rTreg ratio. These data imply that the decrement of aTreg cells contributes the decrement of Treg cells in peripheral blood of RA and BD. Furthermore, the decreased conversion from rTreg or non-Treg cells to aTreg cells is assumed to contribute the total Treg cell decrement. Considering these findings, more detailed fractionation and analysis of subpopulations of Treg cells would be more informative than analysis of total Treg cells in investigating autoimmune diseases, as mentioned in a previous study (16).
In addition, the decreased proportion as well as the absolute count of CD4+ T helper (Th) cells was found in SLE patients. These findings are compatible with previous reports that the proportion of CD4+ cells and CD16/56+ NK cells are significantly lower in SLE patients compared to healthy controls (21).
In conclusion, although there is a limitation for small number subjects, our data demonstrate that the decrement of Treg cell population in RA and BD patients results from the decrement of aTreg cell fraction. It is suggested that the distinction and evaluation of Treg subpulations, especially aTreg cells would be more informative in assessing the dynamics of Treg cells as well as understanding the disease mechanism in autoimmune diseases. Further investigations including a larger number of patients will be needed.
Figures and Tables
Fig. 1
Detection of Treg cells and subpopulations by flowcytometry. (A) CD25 and FoxP3 expression in CD4+ cells. The gated (a) population was regarded as CD25highFoxP3+ Treg cells. (B) CD25 and CD45RA expression in CD4+ cells. The gated (a) population was regarded as CD4+CD25+++CD45RA- activated Treg (aTreg) cells, the (b) population as CD4+CD25++CD45RA+ resting Treg (rTreg) cells and (c) population was regarded as CD4+CD25++CD45RA- non-suppressive T cells. Treg, regulatory T cells; FoxP3, forkhead box P3; PE-Cy5, phycoerythrin-cyanin 5; PE, phycoerythrin.
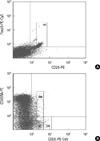
Fig. 2
The proportion of CD3+CD4+ cells and the absolute CD4+ T cell count. (A) The proportion of CD4+ in CD3+ T cell was significantly lower in SLE group than RA, BD, or healthy controls. (B) The absolute count of the CD4+ T cells was significantly lower in SLE group than RA or healthy controls. RA, rheumatoid arthritis; BD, Behcet's disease; SLE, systemic lupus erythematosus; HC, healthy controls; Treg, regulatory T cells; aTreg, activated Treg cells; rTreg, resting Treg cells.
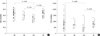
Fig. 3
Comparison of proportions of total Treg cells, aTreg and rTreg subpopulations. (A) The proportion of Treg cells was significantly lower in RA and BD than healthy controls. (B) The proportion of aTreg cells was significantly lower in RA and BD compared to healthy controls. (C) The proportions of rTreg were not different between disease groups and healthy controls. (D) RA patients showed a significantly decreased ratio of aTreg to rTreg. RA, rheumatoid arthritis; BD, Behcet's disease; SLE, systemic lupus erythematosus; HC, healthy controls; Treg, regulatory T cells; aTreg, activated Treg cells; rTreg, resting Treg cells.

References
1. Baecher-Allan C, Brown JA, Freeman GJ, Hafler DA. CD4+CD25high regulatory cells in human peripheral blood. J Immunol. 2001. 167:1245–1253.
2. Yagi H, Nomura T, Nakamura K, Yamazaki S, Kitawaki T, Hori S, Maeda M, Onodera M, Uchiyama T, Fujii S, et al. Crucial role of FOXP3 in the development and function of human CD25+CD4+ regulatory T cells. Int Immunol. 2004. 16:1643–1656.
3. Costantino CM, Baecher-Allan CM, Hafler DA. Human regulatory T cells and autoimmunity. Eur J Immunol. 2008. 38:921–924.
4. Cvetanovich GL, Hafler DA. Human regulatory T cells in autoimmune diseases. Curr Opin Immunol. 2010. 22:753–760.
5. Kuhn A, Beissert S, Krammer PH. CD4(+)CD25 (+) regulatory T cells in human lupus erythematosus. Arch Dermatol Res. 2009. 301:71–81.
6. Zhang B, Zhang X, Tang F, Zhu L, Liu Y. Reduction of forkhead box P3 levels in CD4+CD25high T cells in patients with new-onset systemic lupus erythematosus. Clin Exp Immunol. 2008. 153:182–187.
7. Lyssuk EY, Torgashina AV, Soloviev SK, Nassonov EL, Bykovskaia SN. Reduced number and function of CD4+CD25highFoxP3+ regulatory T cells in patients with systemic lupus erythematosus. Adv Exp Med Biol. 2007. 601:113–119.
8. Kawashiri SY, Kawakami A, Okada A, Koga T, Tamai M, Yamasaki S, Nakamura H, Origuchi T, Ida H, Eguchi K. CD4+CD25highCD127low/-Treg cell frequency from peripheral blood correlates with disease activity in patients with rheumatoid arthritis. J Rheumatol. 2011. 38:2517–2521.
9. Sugita S, Yamada Y, Kaneko S, Horie S, Mochizuki M. Induction of regulatory T cells by infliximab in Behcet's disease. Invest Ophthalmol Vis Sci. 2011. 52:476–484.
10. Valencia X, Stephens G, Goldbach-Mansky R, Wilson M, Shevach EM, Lipsky PE. TNF downmodulates the function of human CD4+CD25hi T-regulatory cells. Blood. 2006. 108:253–261.
11. Yan B, Ye S, Chen G, Kuang M, Shen N, Chen S. Dysfunctional CD4+, CD25+ regulatory T cells in untreated active systemic lupus erythematosus secondary to interferon-alpha-producing antigen-presenting cells. Arthritis Rheum. 2008. 58:801–812.
12. Alvarado-Sánchez B, Hernández-Castro B, Portales-Pérez D, Baranda L, Layseca-Espinosa E, Abud-Mendoza C, Cubillas-Tejeda AC, González-Amaro R. Regulatory T cells in patients with systemic lupus erythematosus. J Autoimmun. 2006. 27:110–118.
13. van Amelsfort JM, Jacobs KM, Bijlsma JW, Lafeber FP, Taams LS. CD4(+)CD25(+) regulatory T cells in rheumatoid arthritis: differences in the presence, phenotype, and function between peripheral blood and synovial fluid. Arthritis Rheum. 2004. 50:2775–2785.
14. Valencia X, Lipsky PE. CD4+CD25+FoxP3+ regulatory T cells in autoimmune diseases. Nat Clin Pract Rheumatol. 2007. 3:619–626.
15. Flores-Borja F, Jury EC, Mauri C, Ehrenstein MR. Defects in CTLA-4 are associated with abnormal regulatory T cell function in rheumatoid arthritis. Proc Natl Acad Sci U S A. 2008. 105:19396–19401.
16. Miyara M, Yoshioka Y, Kitoh A, Shima T, Wing K, Niwa A, Parizot C, Taflin C, Heike T, Valeyre D, et al. Functional delineation and differentiation dynamics of human CD4+ T cells expressing the FoxP3 transcription factor. Immunity. 2009. 30:899–911.
17. Hochberg MC. Updating the American College of Rheumatology revised criteria for the classification of systemic lupus erythematosus. Arthritis Rheum. 1997. 40:1725.
18. International Study. Criteria for diagnosis of Behçet's disease. Lancet. 1990. 335:1078–1080.
19. Aletaha D, Neogi T, Silman AJ, Funovits J, Felson DT, Bingham CO 3rd, Birnbaum NS, Burmester GR, Bykerk VP, Cohen MD, et al. Rheumatoid arthritis classification criteria: an American College of Rheumatology/European League Against Rheumatism Collaborative Initiative. Arthritis Rheum. 2010. 62:2569–2581.
20. Moon HW, Kim BH, Park CM, Hur M, Yun YM, Kim SY, Lee MH. CD4+CD25highFoxP3+ regulatory T-cells in hematologic diseases. Korean J Lab Med. 2011. 31:231–237.
21. Wouters CH, Diegenant C, Ceuppens JL, Degreef H, Stevens EA. The circulating lymphocyte profiles in patients with discoid lupus erythematosus and systemic lupus erythematosus suggest a pathogenetic relationship. Br J Dermatol. 2004. 150:693–700.