Abstract
The influence of spinal cord injury (SCI) on protein expression in the rat urinary bladder was assessed by proteomic analysis at different time intervals post-injury. After contusion SCI between T9 and T10, bladder tissues were processed by 2-DE and MALDI-TOF/MS at 6 hr to 28 days after SCI to identify proteins involved in the healing process of SCI-induced neurogenic bladder. Approximately 1,000 spots from the bladder of SCI and sham groups were visualized and identified. At one day after SCI, the expression levels of three protein were increased, and seven spots were down-regulated, including heat shock protein 27 (Hsp27) and heat shock protein 20 (Hsp20). Fifteen spots such as S100-A11 were differentially expressed seven days post-injury, and seven proteins including transgelin had altered expression patterns 28 days after injury. Of the proteins with altered expression levels, transgelin, S100-A11, Hsp27 and Hsp20 were continuously and variably expressed throughout the entire post-SCI recovery of the bladder. The identified proteins at each time point belong to eight functional categories. The altered expression patterns identified by 2-DE of transgelin and S100-A11 were verified by Western blot. Transgelin and protein S100-A11 may be candidates for protein biomarkers in the bladder healing process after SCI.
Acute traumatic spinal cord injury (SCI) is a destructive neurological disorder that causes defects in sensory and autonomic functions as well as motor paralysis distal to the lesion (1). Sensory and autonomic dysfunctions often lead to urinary bladder impairment, which can be a major cause of morbidity for those with SCI (2). The molecules involved in the impairment of affected spinal cord or neural tissue itself have been relatively well-studied (3-5), but little has been reported about the expression and changes in relevant proteins in sensory and autonomic dysfunctions induced by SCI such as neurogenic bladder.
Neurogenic bladder is any dysfunctional condition of the urinary bladder caused by SCI or other lesions of the central nervous system (CNS). Cortical control over the sacral reflex voiding center is impaired after suprasacral SCI, and subsequent neurogenic detrusor overactivity and/or detrusor-sphincter dyssynergia leads to functional urethral obstruction, urinary retention, and bladder hypertrophy (6). Microarray analysis of gene expression in the bladder after SCI revealed that mRNA levels of molecules involved in tissue remodeling were up-regulated in the post-SCI bladder (7).
In this study, we used a proteomic analysis to identify differentially expressed proteins in the bladder after SCI. The proteins from bladder tissues in spinal cord injured rat and sham control were prepared and processed for 2-DE and MALDI-TOF/MS. To find new biomarkers for the healing process in the post-SCI bladder, dynamic changes in protein profiles identified by 2-DE were analyzed at different time points. Our results may provide insight into the novel candidate target proteins involved in the healing stages of the bladder after SCI and underlying pathophysiological mechanisms between SCI and neurogenic bladder.
The female Sprague-Dawley rats (250-300 g, 12 weeks old, n = 57) were purchased from Koatech Co. (Seoul, Korea). Animal experiments were performed according to the Gyeongsang National University Animal Care and Use Committee (GLA-090701-R0051).
Female rats have relatively short urethra compared to males and were preferentially used for SCI animals suffering from neurogenic voiding dysfunction. For contusion SCI (n = 40), the ninth thoracic cord segment (T9) of rats was exposed under anesthesia and injury was produced by dropping a 2.0 mm diameter rod with 10 g weight from 25 mm height onto the exposed spinal cord using the New York University (NYU) Impactor (New York, NY, USA). Sham-operated rats (n = 17) experienced the same operation as the spinal cord injured rats without contusion injury, and animal care after SCI was performed, as previously described (8). The bladder post-injury was manually voided twice daily until voluntary voiding occurred. The operated rats were randomized and divided into separate groups: 1) early phase of recovery, 6 hr (n = 5), 1 day (n = 6) and 2 days (n = 6) after SCI; 2) intermediate recovery phase, 7 days (n = 6) and 9 days (n = 6) after SCI; 3) late phase of recovery, 21 days (n = 5) and 28 days (n = 6). The rats in the control group were sacrificed at the same time intervals as the SCI groups.
The Basso, Beattie, and Bresnahan (BBB) test (9), a behavioral test for the assessment of hindlimb locomotion after SCI, was performed to evaluate the recovery of locomotor function post-contusion injury with scores from zero (no observable movement) to a maximum of 21 (normal locomotion). The test was performed on immediately after SCI and then continued for four weeks at 6 hr, 1 day, 2, 7, 9, 21, and 28 days post-SCI by two blinded observers. The data were presented as the mean ± SD (sham group [n = 17] and contusion group [n = 40]). Data were evaluated using a one-way ANOVA with a paired t-test using GraphPad Prism 4 for Windows, GraphPad Software, Inc. (La Jolla, CA; www.graphpad.com). Statistical significance was set at P < 0.05.
Bladder tissues of spinal cord injured rats were homogenized in suspension buffer (0.1 g tissue/mL buffer, 50 mM Tris-HCl, pH 7.2, 50 mM EDTA and protease inhibitor cocktail) and processed by centrifugation at 100,000 × g for 10 min at 4℃. The supernatant was precipitated with trichloroacetic acid, and the resulting pellet was dried and dissolved in lysis buffer (8 M urea, 4% CHAPS, 100 mM DTT and 2% ampholytes pH 3-10) with sonication. After centrifugation at 15,000 × g for 15 min at 4℃, the final supernatant was used as a 2-DE sample. Protein concentration was measured with the Bradford reagent (Bio-Rad, Hercules, CA, USA) using bovine serum albumin as a standard.
Samples containing 50 µg (for analytical gel) or up to 600 µg (for micropreparative gel) proteins were diluted with rehydration solution (7 M urea, 2 M thiourea, 2% CHAPS, 100 mM DTT, 0.5% IPG buffer [pH 4-7] and bromophenol blue), and applied to IPG gel strips (Bio-Rad) at 50 V for 14 hr. Isoelectric focusing (IEF) in the first dimension was performed with 18 cm IPG strips (pH 4-7) using Protean IEF Cell (Bio-Rad). After completion of IEF, the strips were placed in an equilibration solution (50 mM Tris-HCl, pH 8.8, 6 M urea, 2% SDS, 30% glycerol, and bromophenol blue) containing 1% DTT for 15 min, and transferred to the equilibration solution containing 2.5% iodoacetamide before placing them on a SDS gel (7.5%-17.5%, 185 × 200 × 1 mm). Separation in the second dimension was performed in Tris-glycine buffer containing 0.1% SDS, and the separated gel was visualized with silver staining for analytical gel and/or Coomassie blue staining for micropreparative gel, as previously described (10).
Protein patterns in the gel were recorded as digitalized image using a high-resolution scanner (GS-710 Calibrated Imaging Densitometer, Bio-Rad) and matching of the gel image was performed with PDQuest software (version 6.2.1, Bio-Rad). For each sample, four gels were run. Differentially expressed proteins in separate gels (P < 0.05 by Student's t-test) were identified for each bladder sample at different healing stages of the spinal cord injured rat compared to the sham control sample. For all spot intensity calculations, normalized values were used.
For protein identification, the desired gel pieces were excised after staining, and processed for tryptic digestion, as previously described (10). Target preparation was performed by solution-phase nitrocellulose method (11). We used a Voyager-DE™ (delayed extraction) STR biospectrometry workstation (Applied Biosystems, Foster City, CA, USA) for MALDI-TOF/MS. Proteins were identified by peptide mass fingerprinting with the search program MS-FIT (http://prospector.ucsf.edu/prospector).
Total proteins (20 µg) from urinary bladder in spinal cord injured rat were separated on a 12% SDS-gel electrophoresis and subsequently transferred to nitrocellulose membrane by a Tank transfer system (Bio-Rad) for 1 hr at 340 mA. After blocking in 5% skim milk/TBST (50 mM Tris-HCl, pH 7.4, 0.1 M NaCl and 0.1% Tween 20) for overnight at 4℃, the membrane was incubated with rabbit polyclonal antibody against transgelin (Santa Cruz Biotechnology, Santa Cruz, CA, USA) or S100 alpha (Abcam, Cambridge, MA, USA) for 1 hr at room temperature and washed three times in TBST for 10 min each. Then the membrane was incubated with goat anti-rabbit IgG HRP (Santa Cruz Biotechnology) for 1 hr and the unbound antibodies were washed with TBST solution thrice for 10 min. Proteins were visualized with an ECL detection system (Pierce Chemical, Rockford, IL, USA).
To assess behavioral outcome of rats with or without contusion SCI, 57 female rats were used to validate the BBB score (9). Fig. 1A shows a picture of spinal cord injured rat with sutured muscles and skin overlying in the back. The hindlimbs of rat post-injury were observed as drooped and paralyzed state. The BBB score of rats in SCI groups and sham control was estimated at different time points (Fig. 1B). The rats in sham group sustained normal hindlimb locomotion and the score maintained almost constant level around from 19 to 21 throughout the experimental period (open circle). However, the BBB score of rats at 1 day after contusion injury appeared near zero, and the score subsequently started to improve from 2 days after SCI and achieved 50% recovery between 7 and 9 days post-injury (P < 0.05). The score of rats with the contusion injury continued to recover to the 80% values of sham control group until 28 days post-injury (closed circle).
To investigate the differentially expressed proteins at healing stages of urinary bladder after SCI, proteomic analysis was performed in the bladder tissues of SCI-affected and sham control rats. All operated animals were evaluated hindlimb locomotor recovery by BBB test (9). The sacrificed rats owing to unsuitable urinary evacuation in the course of recovery process were excluded for the study.
Two-dimensional electrophoretic analysis of the bladder tissue proteins in rat after contusion SCI was carried out at the indicated time points post-injury (6 hr, 1, 2, 7, 9, 21, and 28 days) ranging from five to six animals in each experimental group, and the expression patterns of spots were compared using PDQuest image analysis software. The individual scanned images were matched to form a composite image of the sham and affected tissues. Approximately 1,000 protein spots (616-1,069 spots from each time point) were visualized in a gel (pH range of 4-7 and molecular mass ranges of 10-100 kDa) (Fig. 2). The overall protein expression patterns in the SCI-affected urinary bladder (neurogenic bladder) were quite dissimilar depending on the healing stages after injury, showing significant loss of protein spots in the early phase of recovery (1 and 2 days post-injury), considerable recovery of the spots in the intermediate of mid-phase (7 and 9 days post-injury), and almost complete rescue of the protein spots comparable to the unaffected sham control tissues in the late phase of recovery (21 and 28 days) (A-H in Fig. 2). Spots representing proteins that were up- or down-regulated with more than two-fold difference in 2-DE gels at indicated time points were selected, and further analyzed by comparing their expression patterns. Individual protein spots were picked out, digested with trypsin, and identified by MALDI-TOF/mass spectrometry (Table 1). The criteria used to accept identifications included the extent of sequence coverage, the number of peptides matched, the probabilistic score and also the corresponding values of pI and Mr. The mean intensity of each spot was calculated by its silver stain intensity and the relative intensities between urinary bladder tissues after SCI and sham control were compared. All of these proteins were found to have a statistical significance.
At 6 hr post-injury, of the beginning stage in the healing process of urinary bladder after SCI, 78 kDa glucose regulated protein (GRP) was only identified as a decreasingly expressed protein spot (Table 1). Of 81 up-regulated and 87 down-regulated protein spots in the SCI bladder tissues at 1 day post-injury ten spots were identified including up-regulated 14-3-3 zeta/delta, and down-regulated heat shock protein 27 (Hsp27), heat shock protein 20 (Hsp20), myosin light polypeptide 6 and myosin regulatory light chain 2 (Table 1). At 2 days after injury, 15 spots from 166 differentially expressed spots (108 increased and 58 decreased spots) in the SCI bladder were identified and revealed such as up-regulated apolipoprotein A-I and hemopexin, and down-regulated annexin A5, superoxide dismutase and aldose reductase (Table 1). At 7 days of the intermediate stage of recovery in the bladder after injury, ten proteins showed increased expressions, that is, three isoforms of 14-3-3 protein (epsilon, zeta/delta, beta/alpha) and rho GDP dissociation inhibitor (GDI), and five proteins such as aldehyde dehydrogenase and selenium binding protein were decreasingly expressed (Table 1). At 9 days after injury, three spots from 86 differentially expressed spots in the bladder post-SCI were identified as up-regulated 14-3-3 zeta/delta, myosin regulatory light chain 2 and 14-3-3 beta/alpha (Table 1). At 21 days of the late healing stage in the bladder post-SCI, two repressed proteins including annexin A5 and tubulin beta-5 chain were identified from 30 differentially expressed protein spots, whereas three increased proteins (14-3-3 protein zeta/delta, myosin regulatory light chain 2, and transgelin) and four repressed proteins (78 kDa GRP, apolipoprotein E, haptoglobin, and fetuin B) were revealed after 28 days of injury from 38 up-regulated and 15 down-regulated protein spots, respectively (Table 1).
Of these identified proteins with altered expression level 14-3-3 zeta/delta, myosin regulatory light chain 2, Hsp27, Hsp20, transgelin, and protein S100-A11 were continuously expressed with some fluctuation in the course of healing process of urinary bladder after SCI. Especially, the expression of protein S100-A11 showed peak around 9 days post-injury, the mid-phase of recovery, while the level of transgelin, Hsp27, and Hsp20 increased continuously up to the late phase of recovery in the bladder post-injury (Fig. 3). These data suggest that the recovery status of urinary bladder after SCI is often associated with stress response proteins in signal transduction such as molecular chaperone, inducing metabolic and biochemical alterations (Table 1). The expression level of each protein in both injury-affected urinary bladder and sham control tissues was indicated by estimating of the density values (Fig. 2). Their predicted values of isoelectric point (pI) and molecular weight (MW) are summarized in Table 1, and the values were compatible to those of acquired from the gels. The sequence coverage of proteins isolated from the peptide mass matching in a program was acceptable (20%-40% ranges).
Of four proteins exhibited in Fig. 3, transgelin, which is expressed below half amount of sham control at 1 day post-injury, showed continuously elevated protein expression in bladder up to 28 days after SCI. While protein S100-A11, which is also expressed below half amount of sham control at 1 day post-SCI, displayed peak expression at 9 days post-injury, which is the mid-point of recovery phase and the initial point of spontaneous voiding after SCI, but the expression was declined thereafter. Based on the 2-DE results, transgelin and S100-A11 were further confirmed their expressions in the bladder after SCI by Western blot analysis. The expression level of transgelin and S100-A11 was well agreement with the protein intensities shown in 2-DE gel (Figs. 3, 4). Beta-actin was used as a loading control for the Western blot.
The 54 proteins identified from the bladder tissue after SCI were annotated to achieve biological and functional information of the changed proteins. Fig. 5 shows the alteration of protein expressions in a certain category during recovery of the bladder tissue after SCI (A, 1 day post-SCI; B, 2 days post-SCI; C, 9 days post-SCI; D, 28 days post-SCI). At 2 days post-SCI, the proteins for signal transduction, cytoskeleton and metabolism were quite decreased with more than three-fold, but cell adhesion and migration related proteins were considerably increased compared to the bladder tissues at 1 day post-injury. However, the protein expression patterns at 9 days post-injury, the mid-point of recovery in the bladder post-SCI, turned to comprise most altered proteins involved in signal transduction and cytoskeleton as seen in Fig. 5C. The increased proteins for the active recovery at 9 days post-SCI seemed to significantly decreased thereafter, and proteins related with angiogenesis/circulatory system, carrier, and metabolism were fairly returned and positioned in the rest of protein category at 28 days post-injury (Fig. 5D).
Spinal cord injury relevant to thoracic region from contusion to complete transection initially affects the lower urinary tract function (12). The loss of normal descending control inhibits normal micturition, requiring manual bladder evacuation. The bladder responds by enlarging, and in transection models this is known to initiate the afferent plasticity and subsequent interneuronal alterations resulting in reflex bladder development (13, 14). The same mechanisms occurred after incomplete contusion injury in the first week after SCI (15). The bladder pathologies after SCI comprised not only changes in mechanical properties but also changes in tissue morphology such as hypertrophy and fibrosis (16). In addition, functional deficiencies including bladder areflexia, hyperreflexia, and detrusor-sphincter dyssynergia could be induced in the SCI bladder. However, the molecular mechanisms underlying the observed changes in the bladder following SCI have not yet been elucidated.
In the present study, differentially expressed proteins in the rat bladder after SCI compared with normal bladder were identified at different time points from 6 hr to 28 days post-injury. For this purpose, proteomic analysis using 2-DE, MALDI-TOF/mass spectrometry and peptide mass fingerprinting were utilized.
The protein expression in the SCI-affected urinary bladder showed different profile according to the recovery phase after injury. In the early acute phase (6 hr, 1, and 2 days post-injury), 2-DE gel images of injury-affected bladder tissue exhibited significant loss of protein spots compared to sham control except those of 6 hr (Fig. 2A to D). At this stage, cytoskeletal proteins such as myosin light polypeptide 6, myosin regulatory light chain 2, vinculin, and destrin were remarkably decreased, suggesting SCI as a significant stress to smooth muscle function of urinary bladder. The expression level of Hsp20 and Hsp27 involved in the signal transduction and molecular chaperone machinery was also decreased at 1 day post-injury but gradually recovered their own expression same as sham control up to 28 days after SCI (Fig. 3). On the contrary, the expression of two types of serine protease inhibitor (A3K and A3L) and apolipoprotein (A-I and E) was up-regulated at this stage.
The characteristics of the intermediate phase of recovery (7 and 9 days post-injury) were manifested as up-regulated cytoskeletal proteins and adaptor signaling molecules, and down-regulated metabolic enzymes. Protein S100-A11 (calcium binding protein), tropomyosin alpha-3 chain playing roles in actin filament binding and regulation of smooth muscle contraction, and myosin regulatory light chain 2 (smooth muscle isoform) involved in the cytoskeletal system are increasingly expressed, implicating of the initiation of spontaneous voiding at this mid-stage (Table 1). One of the adaptor molecules, 14-3-3 protein family, has seven isoforms in mammalian. The mammalian 14-3-3 proteins are involved in many intracellular processes including cell cycle regulation, metabolism control, apoptosis, and control of gene transcription (17). Pull-down assays of the 14-3-3 proteins demonstrated the association with phosphopeptide analogs of Hsp20. The binding of 14-3-3 protein to phosphopeptide analogs of Hsp20 prevented the association of cofilin with 14-3-3, suggesting Hsp20 as a modulator of actin cytoskeletal dynamics by competing with the actin depolymerizing protein cofilin for binding to the scaffolding protein 14-3-3. Especially, this association could be modulated by phosphopeptide motifs only within Hsp20 molecule. These data also suggest the possibility that cyclic nucleotide dependent relaxation of smooth muscle might be mediated by a thin filament (actin) regulatory process (18). Three isoforms of 14-3-3 zeta/delta, epsilon, and beta/alpha was continuously increased in expression at mid-stage of the recovery (Table 1) and possible association of each 14-3-3 molecule with Hsp20 should be studied for the future project. Metabolic enzymes at this stage are generally down-regulated, suggesting the priority of the recovery of the smooth muscle contractility in the affected bladder due to SCI.
In the late phase of recovery (21 and 28 days) post-SCI, the protein spots found in unaffected sham control tissues were considerably recovered (A, G and H in Fig. 2). The iron saving molecules such as haptoglobin was no more urgently needed for the late stage of recovery. However, the expression of cytoskeletal proteins such as myosin regulatory light chain 2 and transgelin was considerably increased, and 14-3-3 zeta/delta protein was up-regulated (Table 1). Throughout recovery stages after SCI, four proteins such as transgelin, Hsp27, Hsp20, and protein S100-A11 were continuously expressed as shown in Fig. 3. Three proteins (transgelin, Hsp27 and Hsp20) exhibit similar expression patterns; marked decrease at day 1 after injury and gradual regaining of the protein amounts until late healing stages. On the other hands, protein S100-A11 decreased at 1 day post-injury and markedly increased protein expression at mid-point of recovery phase after injury. We discuss these four proteins for their biochemical and molecular properties and functional implications as below.
Transgelin, also called smooth muscle (SM)-specific SM22 protein, is 22 kDa cytoskeleton related protein. It binds to and co-localizes with F-actin, indicating that it may be involved in cell differentiation by stabilizing the cytoskeleton through actin binding (19, 20). SM22α plays a functional role in inducing F-actin bundling and forming strong stress fibers, which are essential structures for cell contraction (21). As shown in Figs. 3, 4, the level of the transgelin expression was diminished 1 day post-SCI compared to sham control, suggesting the close relationship of transgelin with the bladder smooth muscle contraction. This finding suggests decreased contractility of detrusor muscle due to SCI. Proteomic investigation on chronic irritation of the rat bladder such as interstitial cystitis revealed remarkable up-regulation of four nuclear proteins containing inflammation-associated calgranulin A and marked down-regulation of one nuclear protein (contractility-associated transgelin) compared with normal rat bladders.
Western blot analysis exhibited that time-course expression of transgelin showed maximal decrease of expression at 4 days after brief (90 sec) intravesical HCl irritation and returned to normal expression at 28 days after the treatment (22). The decreased expression of transgelin and restoring to control level at 4 weeks post-injury are validated not only in chronic bladder irritation in rat by HCl but also in the rat bladder after contusion SCI. Down-regulated transgelin expression was also observed by proteomic investigation after partial bladder outlet obstruction (23). On the other hands, an interesting study about the effects of SCI on alterations in gene expression and respective protein products using human skeletal muscle was reported 2 days and 5 days post-SCI. These data suggest that the primary signaling networks activated in the days post-SCI are those involved in protein degradation (ubiquitin proteasome pathway), and those which may function to protect skeletal muscle from rapid degradation (metallothioneins) (16). Hence, it is suggested that there can be some different protein expression patterns in the recovery after SCI between skeletal muscle and smooth muscle.
S100 proteins belong to the EF-hand calcium-binding protein family, which are expressed in cell- and tissue-specific manners, regulate a variety of cellular processes via interaction with different target proteins (24). S100-A11 (previously named S100C or calgizzarin) is a novel and relatively unknown member of the large family of S100 proteins, and exhibits several unique properties. S100-A11 was found to regulate the cytoskeletal function via calcium-dependent interaction with annexin I (25). S100-A11 expression was significantly increased in leiomyoma, human uterine smooth muscle tumors (26). Suppression of S100-A11 by small interfering RNA (siRNA) led to apoptosis, and the over-expression of S100-A11 inhibited apoptosis in human uterine smooth muscle tumor cells, suggesting S100-A11 has an anti-apoptotic function. Investigation of the molecules participating in the metabolism of calcium/calmodulin may provide new insights into the pathophysiology of various smooth muscle diseases as well as cardiac and skeletal muscle diseases (26). S100-A11 protein expressed in the bladder tissue after SCI is shown with peak increase in the mid-point of recovery phase post-injury and decreases thereafter, suggesting that it can be associated with signal transduction of calcium/calmodulin relevant muscle contraction and inhibition of apoptosis in the initiation stage of spontaneous voiding in the smooth muscle of bladder tissue after SCI (Figs. 3, 4).
In the past few years, many proteins have been found to be involved in the injured spinal cord tissue (3, 5). Of these proteins, Hsp27 belong to the small heat shock protein family is differentially expressed both in spinal cord tissue itself and SCI-affected bladder tissue (Fig. 3). Exercise-induced Hsp27 mRNAs expression in the soleus muscle is dependent on time after SCI in rats (27). The expression level of Hsp27 in the spinal cord after SCI varied with the injury type and duration of recovery (5, 16).
Another small heat shock protein, Hsp20, is expressed in nearly all mammalian tissues. Hsp20 expression in smooth muscles is variable. Low levels of Hsp20 expression in the normal bladder are converted to substantial expression when the bladder is obstructed (28). Low or no expression of Hsp20 is associated with diminished cyclic nucleotide-induced relaxation, suggesting phosphorylation of Hsp20 can be a dominant mechanism of smooth muscle relaxation (29). The expression of Hsp20 in smooth muscle of the affected bladder after SCI exhibited similar pattern shown in Hsp27 (Fig. 3).
In conclusion, this study displays a proteomic profiling of the affected bladder after contusion SCI at seven time points from 6 hr to 28 days. During entire recovery phase post-injury, 54 proteins related with SCI-affected bladder tissue are identified. Of four proteins which are continuously expressed with different patterns, two proteins including transgelin and S100-A11 are confirmed their expression level by Western blot analysis. The next goal for the future study will be focused on Hsp20 and 14-3-3, two intriguing proteins identified by this proteome research, elucidating biochemical and pathophysiological relationship between two molecules in the affected urinary bladder after SCI.
Figures and Tables
Fig. 1
Evaluation of spinal cord injury by locomotor behavior scoring. (A) A photograph of spinal cord injured rat. Sutured muscles and skin in the back after surgery are shown, and hindlimbs are drooped and paralyzed. (B) Basso Beattie Bresnahan (BBB) open field locomotor rating scale is scored in the course of healing process after contusion SCI. Adult rats (n = 5-6 animals per group) were contused at T-9 exposed spinal cord with a 250 gram weight drop device from a 25 mm height using the New York University (NYU) Impactor. Locomotor behavior was evaluated before injury and then for up to 28 days with different time intervals. The graph exhibits functional hindlimb recovery of rats in contusion group compared with those of sham control group. Data were presented as mean ± SD and P < 0.05 in both groups.
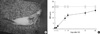
Fig. 2
Comparison of protein expression patterns of rat urinary bladder after contusion SCI at different time points. The bladder tissue proteins (50 µg) from each stage (sham control [A], 6 hr [B], 1 day [C], 2 days [D], 7 days [E], 9 days [F], 21 days [G], and 28 days [H] post-injury) were separated on pH 4?? IPG strips (17 cm, Bio-Rad) in the first dimension and on 7.5%-17.5% linear-gradient SDS-PAGE in the second dimension, and visualized by silver nitrate staining. Protein names specified by Arabic numerals (A and H) are listed in Table 1.
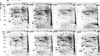
Fig. 3
Expression profiles of selected protein spots in the bladder post-injury at different time points. Representative spots specified at each stage (spot intensity from PDQuest software), identified as transgelin, protein S100-A11, Hsp27, and Hsp20 are shown in the healing course of rat bladder after contusion spinal cord injury. The bar graphs exhibited the expression level of each protein at indicated time points compared with the sham control. Data are presented mean ± SD (n = 5 per group), P < 0.05 (ANOVA).
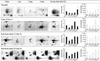
Fig. 4
Validation of differentially expressed proteins in the rat bladder after contusion injury by Western blot analysis. Selected proteins in rat bladder tissues after SCI and sham control were analyzed and confirmed by Western blot at each time points. The expression levels of transgelin and S100-A11 in the protein blot are well agreement with quantitative 2-DE results (Fig. 3), respectively. β-actin was used as a loading control.
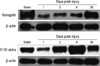
Fig. 5
Functional manifestations of differentially expressed proteins in rat bladder tissues after SCI. The expression profiles and functions of the proteins were categorized into eight different subgroups. Values exhibited the number of identified proteins in each category. (A) to (D) shows total proteins expressed at indicated time points: (A) 1 day post-injury, (B) 2 days post-injury, (C) 9 days post-injury, and (D) 28 days post-injury.
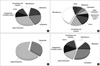
Table 1
Identification of differentially expressed spots by MALDI-TOF/MS in rat bladder post-SCI at indicated time points
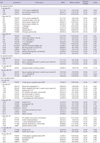
*Protein names and accession numbers are listed according to the SWISS-PROT database. SSP, standard spot numbers; SCI, spinal cord injury; MW, molecular weight; pI, isoelectrical point. Arabic numerals in parentheses specified identified proteins in Fig. 2.
AUTHOR SUMMARY
Spinal Cord Injury Markedly Altered Protein Expression Patterns in the Affected Rat Urinary Bladder during Healing Stages
Ji-Young Lee, Bong Jo Kim, Gyujin Sim, Gyu-Tae Kim, Dawon Kang, Jae Hun Jung, Jeong Seok Hwa, Yeon Ju Kwak, Yeon Jin Choi, Young Sook Park, Jaehee Han, Cheol Soon Lee and Kee Ryeon Kang
We investigated the influence of spinal cord injury (SCI) on the protein expressions in rat urinary bladder using proteomic analysis. The bladder tissues were processed for 2-DE and MALDI-TOF/MS from 6 hr to 28 days after the contusion SCI between T9 and T10 SCI. 54 proteins were found to show altered expression levels, and among them, transgelin, S100-A11, Hsp27, and Hsp20 were continuously expressed with variation in entire recovery period post-injury. The changes in transgelin and S100-A11 were confirmed by Western blot analysis. The results suggest that transgelin and protein S100-A11 might be involved in the healing process of bladder after SCI.
References
1. Saganová K, Orendácová J, Sulla I Jr, Filipcík P, Cízková D, Vanický I. Effects of long-term FK506 administration on functional and histopathological outcome after spinal cord injury in adult rat. Cell Mol Neurobiol. 2009. 29:1045–1051.
2. Nath M, Wheeler JS Jr, Walter JS. Urologic aspects of traumatic central cord syndrome. J Am Paraplegia Soc. 1993. 16:160–164.
3. Shen LF, Cheng H, Tsai MC, Kuo HS, Chak KF. PAL31 may play an important role as inflammatory modulator in the repair process of the spinal cord injury rat. J Neurochem. 2009. 108:1187–1197.
4. Komori N, Takemori N, Kim HK, Singh A, Hwang SH, Foreman RD, Chung K, Chung JM, Matsumoto H. Proteomics study of neuropathic and nonneuropathic dorsal root ganglia: altered protein regulation following segmental spinal nerve ligation injury. Physiol Genomics. 2007. 29:215–230.
5. Kang SK, So HH, Moon YS, Kim CH. Proteomic analysis of injured spinal cord tissue proteins using 2-DE and MALDI-TOF MS. Proteomics. 2006. 6:2797–2812.
6. de Groat WC, Yoshimura N. Changes in afferent activity after spinal cord injury. Neurourol Urodyn. 2010. 29:63–76.
7. Wognum S, Lagoa CE, Nagatomi J, Sacks MS, Vodovotz Y. An exploratory pathways analysis of temporal changes induced by spinal cord injury in the rat bladder wall: insights on remodeling and inflammation. PLoS One. 2009. 4:e5852.
8. Jang I, La JH, Kim GT, Lee JS, Kim EJ, Lee ES, Kim SJ, Seo JM, Ahn SH, Park JY, Hong SG, Kang D, Han J. Single-channel recording of TASK-3-like K channel and up-regulation of TASK-3 mRNA expression after spinal cord injury in rat dorsal root ganglion neurons. Korean J Physiol Pharmacol. 2008. 12:245–251.
9. Basso DM, Beattie MS, Bresnahan JC. A sensitive and reliable locomotor rating scale for open field testing in rats. J Neurotrauma. 1995. 12:1–21.
10. Hwa JS, Kim HJ, Goo BM, Park HJ, Kim CW, Chung KH, Park HC, Chang SH, Kim YW, Kim DR, Cho GJ, Choi WS, Kang KR. The expression of ketohexokinase is diminished in human clear cell type of renal cell carcinoma. Proteomics. 2006. 6:1077–1084.
11. Landry F, Lombardo CR, Smith JW. A method for application of samples to matrix-assisted laser desorption ionization time-of-flight targets that enhances peptide detection. Anal Biochem. 2000. 279:1–8.
12. Jamison J, Maguire S, McCann J. Catheter policies for management of long term voiding problems in adults with neurogenic bladder disorders. Cochrane Database Syst Rev. 2004. 2:CD004375.
13. Hansen J, Media S, Nøhr M, Biering-Sørensen F, Sinkjaer T, Rijkhoff NJ. Treatment of neurogenic detrusor overactivity in spinal cord injured patients by conditional electrical stimulation. J Urol. 2005. 173:2035–2039.
14. Mitsui T, Fischer I, Shumsky JS, Murray M. Transplants of fibroblasts expressing BDNF and NT-3 promote recovery of bladder and hindlimb function following spinal contusion injury in rats. Exp Neurol. 2005. 194:410–431.
15. Yan X, Liu J, Luo Z, Ding Q, Mao X, Yan M, Yang S, Hu X, Huang J, Luo Z. Proteomic profiling of proteins in rat spinal cord induced by contusion injury. Neurochem Int. 2010. 56:971–983.
16. Urso ML, Chen YW, Scrimgeour AG, Lee PC, Lee KF, Clarkson PM. Alterations in mRNA expression and protein products following spinal cord injury in humans. J Physiol. 2007. 579:877–892.
17. van Hemert MJ, Steensma HY, van Heusden GP. 14-3-3 proteins: key regulators of cell division, signalling and apoptosis. Bioessays. 2001. 23:936–946.
18. Dreiza CM, Brophy CM, Komalavilas P, Furnish EJ, Joshi L, Pallero MA, Murphy-Ullrich JE, von Rechenberg M, Ho YS, Richardson B, Xu N, Zhen Y, Peltier JM, Panitch A. Transducible heat shock protein 20 (HSP20) phosphopeptide alters cytoskeletal dynamics. FASEB J. 2005. 19:261–263.
19. Chiavegato A, Roelofs M, Franch R, Castellucci E, Sarinella F, Sartore S. Differential expression of SM22 isoforms in myofibroblasts and smooth muscle cells from rabbit bladder. J Muscle Res Cell Motil. 1999. 20:133–146.
20. Prasad PD, Stanton JA, Assinder SJ. Expression of the actin-associated protein transgelin (SM22) is decreased in prostate cancer. Cell Tissue Res. 2010. 339:337–347.
21. Han M, Dong LH, Zheng B, Shi JH, Wen JK, Cheng Y. Smooth muscle 22 alpha maintains the differentiated phenotype of vascular smooth muscle cells by inducing filamentous actin bundling. Life Sci. 2009. 84:394–401.
22. Tyagi P, Chen X, Hayashi Y, Yoshimura N, Chancellor MB, de Miguel F. Proteomic investigation on chronic bladder irritation in the rat. Urology. 2008. 71:536–540.
23. Kim HJ, Sohng I, Kim DH, Lee DC, Hwang CH, Park JY, Ryu JW. Investigation of early protein changes in the urinary bladder following partial bladder outlet obstruction by proteomic approach. J Korean Med Sci. 2005. 20:1000–1005.
24. Zimmer DB, Cornwall EH, Landar A, Song W. The S100 protein family: history, function, and expression. Brain Res Bull. 1995. 37:417–429.
25. Naka M, Qing ZX, Sasaki T, Kise H, Tawara I, Hamaguchi S, Tanaka T. Purification and characterization of a novel calcium-binding protein, S100C, from porcine heart. Biochim Biophys Acta. 1994. 1223:348–353.
26. Kanamori T, Takakura K, Mandai M, Kariya M, Fukuhara K, Sakaguchi M, Huh NH, Saito K, Sakurai T, Fujita J, Fujii S. Increased expression of calcium-binding protein S100 in human uterine smooth muscle tumours. Mol Hum Reprod. 2004. 10:735–742.
27. Dupont-Versteegden EE, Houlé JD, Dennis RA, Zhang J, Knox M, Wagoner G, Peterson CA. Exercise-induced gene expression in soleus muscle is dependent on time after spinal cord injury in rats. Muscle Nerve. 2004. 29:73–81.
28. Batts TW, Klausner AP, Jin Z, Meeks MK, Ripley ML, Yang SK, Tuttle JB, Steers WD, Rembold CM. Increased expression of heat shock protein 20 and decreased contractile stress in obstructed rat bladder. J Urol. 2006. 176:1679–1684.
29. Salinthone S, Tyagi M, Gerthoffer WT. Small heat shock proteins in smooth muscle. Pharmacol Ther. 2008. 119:44–54.