Abstract
Brief episodes of cerebral hypoxia-ischemia cause transient ischemic tolerance to subsequent ischemic events that are otherwise lethal. This study was conducted to evaluate the protective effect of hypoxic preconditioning on hypoxic-ischemic injury in the neonatal rat and the persistence of a protective window after hypoxic preconditioning. The rats were preconditioned with hypoxia (8% oxygen, 92% nitrogen) for three hours, subjected to ischemia using ligation of the right common carotid artery, and then exposed to another three hours of hypoxia. Using proton magnetic resonance spectroscopy, terminal deoxynucleotidyl transferase-mediated dUTP-biotin nick end-labeling (TUNEL) staining, and morphologic scores, this study shows that hypoxic preconditioning 6-hr to 1-day before hypoxic-ischemic injury increases survival rates and has neuroprotective effects against subsequent hypoxic-ischemic injury. The mechanism of the protective effects of hypoxic preconditioning in the newborn rat brain may involve downregulation of apoptotic cell death.
Hypoxic-ischemic encephalopathy due to birth asphyxia is the major factor that induces neuronal cell injury before, during, and after birth. Although there has been marked development in the fields of obstetrics and neonatology, the incidence of and mortality caused by hypoxic-ischemic encephalopathy remain elevated (1, 2).
Low birth weight infants and infants with intrauterine growth retardation, who have already experienced intrauterine repetitive hypoxic distress, seem to have milder hypoxic-ischemic brain damage than full-term newborns who are exposed to a lethal ischemic insult in the perinatal period. Therefore, some have suggested the possibility that sublethal hypoxic episodes afford a protective effect against further hypoxic exposure (3, 4).
Hypoxic preconditioning has been described in the brain, heart, retina, and other tissues. Gidday et al. (5) first showed that exposure of neonatal rat pups to hypoxia alone (8% oxygen for 3 hr) protected these animals 1 day later from a stroke induced by combined hypoxia/ischemia.
Although the mechanisms of preconditioning are still undetermined, it appears to require synthesis of new RNA and proteins during the reoxygenation period before permanent ligation (6, 7). In addition, Miller et al. (8) explained that the difference in the degree of hypoxia and the delay between preconditioning and ischemia determines the efficacy of preconditioning.
This study was conducted to evaluate the protective effect of hypoxic preconditioning on hypoxic-ischemic injury in the neonatal rat and the persistence of a protective window after hypoxic preconditioning. Furthermore, this study investigated the relationship between the downregulation of apoptosis and its possible role in the protective effect of hypoxic preconditioning. The survival rates, as well as ratios of lipid to N-acetyl aspartate (NAA) and lipid to creatine (Cr), were investigated in positive cells, and morphologic changes were investigated with proton (1H) magnetic resonance spectroscopy (MRS) and terminal deoxynucleotidyl transferase-mediated dUTP-biotin nick end-labeling (TUNEL) staining.
A total of 231 seven-day-old Sprague-Dawley newborn rats weighing 12-18 grams were divided into six groups: preconditioned 6 hr before hypoxic-ischemic injury (Pre-6 hr, n = 30), 12 hr before (Pre-12 hr, n = 32), 1 day before (Pre-1 day, n = 34), 3 days before (Pre-3 day, n = 41), 6 days before (Pre-6 day, n = 43), and control without preconditioning (n = 51). All experimental animal protocols were approved by the University of Ulsan College of Medicine IACUC Committee (2008-12-011).
The rats were preconditioned by hypoxic exposure (8% oxygen/92% nitrogen) for three hours at 37℃. Exhaled carbon dioxide was removed through a one-way valve in the lower part of a hypoxic chamber. Rats were returned to their home cages at room temperature immediately after the hypoxic preconditioning. Control group rats were isolated during preconditioning, and then they were also returned to their home cages.
Using ligation of the right common carotid artery, 6 hr, 12 hr, 1, 3, and 6 days after receiving three hours of hypoxic preconditioning, all rats, including the control group, were subjected to ischemia. The right common carotid artery was dissected and ligated using silk (No. 5) under isoflurane anesthesia, and the rats were submitted to hypoxia (8% oxygen, 92% nitrogen) for 3 hr under the same conditions as in the preconditioning procedure. After the hypoxic-ischemic insult, the specimens were returned to their home cages at room temperature. The schematic diagram in Fig. 1 illustrates our hypoxic-ischemic animal model.
1H MRS was performed 24 hr after hypoxic-ischemic insult, and the results were examined using Bruker Biospec 4.7 Tesla MRI/MRS system (Bruker, Fallanden, Switzerland). The voxel for MRS was located in the parietotemporal area, and the volume of a single voxel was 3 × 3 × 4 µL. The spin echo signal was detected by TR = 3,000 msec, TE = 30 msec, and NS = 128, and the H2O signal was inhibited by a chemical shift selective (CHESS) sequence. Spectra were analyzed semi-quantitively using an Aspect 3000 computer and Tomikon software (Bruker, Fallanden, Switzerland). In order to measure the ratios of lipid to NAA and lipid to Cr as early predictors of apoptosis, the 1H spectra of NAA, creatine, choline, and lipids were assessed at 2.02, 3.03, 3.24, and 1.3 ppm, respectively. To differentiate between lipids and lactate at 1.3 ppm, the presence of the peak at TE = 135 msec was analyzed.
One day after the hypoxic-ischemic injury, the rats were anesthetized with an intra-abdominal ketamine (2 mg/kg) injection following 1H MRS examination. The rats were then perfused through the left ventricle of the heart with heparinized saline (2 unit heparin/1 mL saline, 2.5 mL/g) followed by buffered paraformaldehyde (4% in phosphate buffer, pH 7.4). The brains were extracted, frozen, and enveloped in paraffin.
Two rats in each group were sacrificed one day after hypoxic-ischemic injury, and these brains were examined for histologic study using TUNEL staining. TUNEL staining for the detection of DNA fragmentation produced during cell apoptosis began with the digestion of deparaffinized sections using proteinase K, blocking solution, and permeabilization solution. These sections were then stained with the TUNEL reaction mixture (50 µL) of an in situ cell death detection kit (Boehringer-Mannheim, Germany). Tissue sections were evaluated using immunofluorescence microscopy.
The remaining rats were morphologically evaluated on the fourteenth day after hypoxic-ischemic injury and scored according to the following scale of modified Palmer's classification (9): 0, no difference between the two hemispheres in shape and size; 1, mild reduction of the right hemisphere volume only; 2, volume reduction and atrophic changes with small cysts in the right hemisphere; 3, moderate atrophic changes of the right hemisphere; and 4, severe atrophic changes of the right hemisphere.
The results were expressed as mean ± standard deviation, and the statistics were calculated using an unpaired t-test, one way ANOVA, Tukey's test, Dunn's method, Mann-Whitney U test, and Pearson's correlation with the threshold of significance at 0.05.
Here we confirmed the preconditioning groups, followed by 8% oxygen hypoxia, reduced brain injury in newborn rats. The Pre-6 hr, Pre-12 hr, and Pre-1 day hypoxic preconditioning groups had significantly higher survival rates when evaluating 152 rats among 231: control (25 rats, 47%); Pre-6 hr (26 rats, 86%); Pre-12 hr (32 rats, 100%); Pre-1 day (32 rats, 94%); Pre-3 day (19 rats, 45%); and Pre-6 day (18 rats, 42%) (Fig. 2).
We performed 1H MRS study after hypoxic-ischemic insult; and then lipid/NAA (N-acetyl aspartate) and lipid/Cr (creatine) ratios were measured as early predictors of apoptosis. The Pre-6 hr, Pre-12 hr, and Pre-1 day hypoxic preconditioning groups also had lower lipid/NAA and lipid/Cr ratios on 1H MRS in comparison with the Pre-3 day and Pre-6 day (P < 0.05, Fig. 3). The lipid/NAA ratios in control, Pre-6 hr, Pre-12 hr, Pre-1 day, Pre-3 day and Pre-6 day ratios were 8.05 ± 2.95, 4.64 ± 2.78, 5.25 ± 2.58, 5.05 ± 1.86, 7.09 ± 2.87, and 6.19 ± 2.62, respectively.
Results of the histologic evaluation demonstrated that the hypoxic preconditioning attenuated hypoxic-ischemic induced apoptosis in newborn brain. On day 1, the groups that received hypoxic preconditioning 6 hr, 12 hr, and 1 day prior to hypoxic-ischemic injury had fewer TUNEL-positive apoptotic cells present than in the control group, which received no preconditioning. The apoptotic cells were found predominantly in cortex and hippocampus. In addition, the 1H MRS characteristics are shown and lower lipid peaks are observed in the preconditioning groups in Fig. 4.
We monitored the structural damage in rats of different groups on the fourteenth day after hypoxic-ischemic injury. The morphologic scores of the preconditioning groups were also significantly lower than those of the control group (P < 0.05, Fig. 5). The morphologic scores in the control, Pre-6 hr, Pre-12 hr, Pre-1 day, Pre-3 day, and Pre-6 day groups were 3.28 ± 0.84, 1.12 ± 1.21, 1.59 ± 1.46, 1.69 ± 1.15, 2.95 ± 1.08, and 2.61 ± 1.54, respectively, at day 14.
The high lipid/NAA and lipid/Cr ratios on 1H MRS were obtained on the first day after hypoxic-ischemic insult and were predictive of morphologic changes (Fig. 6).
However, the 1H MRS and morphologic scores of the Pre-3 day and Pre-6 day preconditioning groups did not show a protective effect on survival rates or on lipid/NAA and lipid/Cr ratios.
Both fetal and neonatal asphyxia can cause cerebral hypoxic-ischemic injury, resulting in severe neurologic sequelae and death. Survivors of perinatal asphyxia frequently have moderate to severe brain injury for which there is currently no promising therapy.
Reports published in the last decade have made increasing use of the term hypoxic or ischemic preconditioning to describe an adaptive response to subsequent lethal events (3). Focusing on protective strategies to prevent and reduce hypoxic-ischemic injury, sublethal stimuli, such as hypoxia (4), hyperthermia (10), hypothermia (11), glutamate and seizure (12), oxygen-glucose deprivation (13), receptor blockade/activation (14), and cytokine (15), have been reported.
The mechanism of the brain tolerance induced by hypoxic preconditioning has not been fully elucidated, but three major mechanisms have been proposed.
First, the formation of various types of prolonged neuronal adaptive responses is associated with the activation of the cell genome and protein synthesis. These include stress proteins (heat shock proteins [HSP]) (10); immediate early genes (c-fos, c-jun, krox-24 [NGFI-A]) (16); transcription factors (hypoxia-inducible factor-1α [HIF-1α]) (17); NFκB (nuclear factor κB) (18); cAMP response element-binding protein (CREB) (19); neuromodulatory peptides for survival, differentiation, and plasticity; growth factors (IGF-1, brain-derived neurotrophic factor [BDNF], NGF) (20); and anti-apoptotic genes (bcl-2, bcl-xl, bax) (3). In particular, an important role is played by immediate early gene superfamilies whose protein products are transcription factors that regulate the expression of phenotype-specific late genes.
A rather long time (24 hr or more) between mild preconditioning episodes and severe hypoxia is needed for the maximal protective effect of preconditioning to appear, which suggests the involvement of neuronal gene expression and de novo protein synthesis in the mechanisms of brain tolerance (7). For example, Kitagawa et al. (21) found that sublethal ischemia at 1 and 2 days prior to an ischemic insult that is normally lethal to neurons was neuroprotective in vivo.
Our data, however, showed significant neuroprotective effects in the Pre-6 hr hypoxic preconditioning group. This suggests that the factors mentioned above could be induced to influence the tolerance of the brain in as little as 6 hr, and the persistence of the protective effect lasted 3 days after preconditioning.
The second possibility involves the roles of signaling proteins (NO, ERK1/2, Akt) (22). NO, an important proximal component of the transduction pathway of adaptive genomic changes, was shown to induce diverse paracrine actions in the central nervous system. NO production and activity were critical to the induction of ischemic tolerance.
Third, the roles of molecules, including adenosine A1 receptors (23) and manganese superoxide dismutase (MnSOD) (24) have suggested a possible mechanism.
Finally, activation of N-methyl-D-aspartate (NMDA) receptors has been shown to be involved in the development of both adaptive and pathological brain reactions. Glutamate has long been known to kill neurons by NMDA receptor-mediated mechanisms. Paradoxically, subtoxic concentrations of NMDA protect neurons against glutamate-mediated excitotoxicity (1, 14). The major mechanism of NMDA receptor-medicated tolerance after preconditioning involves HSP induction; BCL-2 gene expression; activation of adenosine A1 receptors; release and synthesis of BDNF by activation of NFκB; TrkB receptor, Akt activation through phosphoinositide 3-kinase, and calmodulin-dependent protein kinase; and downregulation of the mixed-lineage kinase 3 (MLK3)-c-Jun N-terminal kinase (JNK) signaling pathway via Akt activation (22).
According to data from the present study, the Pre-6 hr, Pre-12 hr, and Pre-1 day hypoxic preconditioning groups had significantly higher survival rates than those of the control group. These results suggest that preconditioning was effective in myocardial cells as well as cells in the brain. Mild, short ischemic events in myocytes contributed to the resistance to subsequent, lethal ischemic exposure, which reduced cardiac infarction and improved cardiac function during reperfusion after ischemic insult. The survival rates showed the possibility of cardiac tolerance through preconditioning.
It is well known that apoptosis plays an important role in hypoxic-ischemic brain injury and that the apoptotic cell counts are closely related to the degree of brain injury (25). Evidence of fragmented and condensed DNA is apparent using the TUNEL method of in situ DNA end labeling. Cantagrel et al. (3) found a decrease in the number of apoptotic cells 24 and 48 hr after the insult using a newborn rat model of hypoxic preconditioning (8% oxygen/92% nitrogen, 3 hr, 37℃) on day 6 followed by carotid ligation and hypoxic insult on day 7, suggesting that regulation of apoptotic cell death is one of the mechanisms involved in the tolerance to hypoxia-ischemia that is induced by hypoxic preconditioning. Using the same experimental model, we investigated the preconditioning effects in the present study.
MRS allows for the study of brain biochemistry and metabolism and for indirect characterization of the composition of brain tissue in vivo. MRS has become an important adjunct to diagnostic structural imaging that permits more accurate and earlier determination of prognosis after hypoxia-ischemia in the newborn (26-28). Furthermore, non-invasive in vivo 1H MRS of a hypoxic-ischemic brain might reveal apoptosis (29). In 1H MRS of hypoxic-ischemic newborn rat brains, the prominent lipid peaks at 1.3 ppm appear early, and the apoptotic cell counts might be correlated with the intensity of the lipid peak and the ratios of lipid/NAA and lipid/Cr. Therefore, the ratios of lipid/NAA and lipid/Cr could be used as an early indicator to diagnose hypoxic-ischemic injury by apoptosis and also to assess the effect of preconditioning on decreasing apoptosis.
We have used 1H MRS in vivo for the purpose of early detection in the hypoxic-ischemic brain injury model (30). Additionally, the results of the current study demonstrate that the increase of the lipid peak at 24 hr following hypoxic-ischemic injury is correlated with morphologic scores. We suggest a promising future for early in vivo detection of apoptosis using 1H MRS, which can be used to develop new therapeutic methods to attenuate apoptosis in the hypoxic-ischemic injured brain.
In conclusion, using 1H MRS, TUNEL staining, and morphologic scores, this study showes that hypoxic preconditioning 6 hr to 1 day before hypoxic-ischemic injury produces higher survival rates and the presence of neuroprotective effects against subsequent hypoxic-ischemic injury. However, hypoxic preconditioning in the Pre-3 day & Pre-6 day groups does not show these protective effects. The downregulation of apoptotic cell death may be a mechanism underlying the protective effects of hypoxic preconditioning in the newborn rat brain.
Figures and Tables
Fig. 1
Schematic diagram illustrating the hypoxic-ischemic animal model. One day after hypoxic-ischemic injury, the number of TUNEL-positive cells and the lipid peak on 1H MRS in the right brain markedly increase. Fourteen days after the insult, severe atrophy of the right hemisphere is observed.
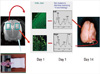
Fig. 2
The effect of hypoxic preconditioning on survival rates after hypoxic-ischemic brain injury in newborn rats. The survival rates are higher in the Pre-1 day, Pre-12 hr, and Pre-6 hr groups than in the control group. The values are expressed as mean ± standard deviation.
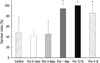
Fig. 3
The ratios of lipid/N-acetyl aspartate (Lip/NAA) and lipid/creatine (Lip/Cr) on 1H MRS in the control group and hypoxic preconditioned groups one day after hypoxic-ischemic brain injury in the newborn rat. The ratios of lipid/NAA and lipid/Cr at the hypoxic-ischemic injured right brain decrease significantly in the Pre-1 day, Pre-12 hr, and Pre-6 hr groups. The values are expressed as mean ± standard deviation. *P < 0.05.
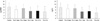
Fig. 4
Histologic findings of TUNEL staining in the newborn rat brain obtained on the first day after hypoxic-ischemic injury. Increased TUNEL-positive cells are noted in the right hemisphere in the control, Pre-6 day, and Pre-3 day groups. Increased 1H MRS lipid peaks were observed in the control, Pre-6 day, and Pre-3 day groups.
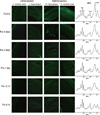
Fig. 5
The effect of hypoxic preconditioning on morphologic scores of the newborn rat brain fourteen days after hypoxic-ischemic brain injury. The morphologic scores are significantly lower in the Pre-1 day, Pre-12 hr, and Pre-6 hr groups than in the control group (*P < 0.05). The values are expressed as mean ± standard deviation.
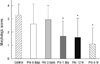
AUTHOR SUMMARY
Protective Effect of Hypoxic Preconditioning on Hypoxic-Ischemic Injured Newborn Rats
Hyun-Kyung Park, In-Joon Seol and Ki-Soo Kim
Brief episodes of hypoxia before subsequent lethal ischemic events could be helpful. The protective effect of hypoxic preconditioning on hypoxic-ischemic brain injury was investigated in the neonatal rat. Our study showed that hypoxic preconditioning 6-hr to 1-day before hypoxic-ischemic injury increased survival rates and had neuroprotective effects against subsequent hypoxic-ischemic injury.
References
1. Lee JM, Grabb MC, Zipfel GJ, Choi DW. Brain tissue responses to ischemia. J Clin Invest. 2000. 106:723–731.
2. Pin TW, Eldridge B, Galea MP. A review of developmental outcomes of term infants with post-asphyxia neonatal encephalopathy. Eur J Paediatr Neurol. 2009. 13:224–234.
3. Cantagrel S, Krier C, Ducrocq S, Bodard S, Payen V, Laugier J, Guilloteau D, Chalon S. Hypoxic preconditioning reduces apoptosis in a rat model of immature brain hypoxia-ischaemia. Neurosci Lett. 2003. 347:106–110.
4. Sanders RD, Manning HJ, Robertson NJ, Ma D, Edwards AD, Hagberg H, Maze M. Preconditioning and postinsult therapies for perinatal hypoxic-ischemic injury at term. Anesthesiology. 2010. 113:233–249.
5. Gidday JM, Fitzgibbons JC, Shah AR, Park TS. Neuroprotection from ischemic brain injury by hypoxic preconditioning in the neonatal rat. Neurosci Lett. 1994. 168:221–224.
6. Cadenas S, Aragonés J, Landázuri MO. Mitochondrial reprogramming through cardiac oxygen sensors in ischaemic heart disease. Cardiovasc Res. 2010. 88:219–228.
7. Wrang ML, Moller F, Alsbo CW, Diemer NH. Changes in gene expression following induction of ischemic tolerance in rat brain: detection and verification. J Neurosci Res. 2001. 65:54–58.
8. Miller BA, Perez RS, Shah AR, Gonzales ER, Park TS, Gidday JM. Cerebral protection by hypoxic preconditioning in a murine model of focal ischemia-reperfusion. Neuroreport. 2001. 12:1663–1669.
9. Palmer C, Vannucci RC, Towfighi J. Reduction of perinatal hypoxic-ischemic brain damage with allopurinol. Pediatr Res. 1990. 27:332–336.
10. Sivaswamy S, Neafsey EJ, Collins MA. Neuroprotective preconditioning of rat brain cultures with ethanol: potential transduction by PKC isoforms and focal adhesion kinase upstream of increases in effector heat shock proteins. Eur J Neurosci. 2010. 32:1800–1812.
11. Nishio S, Chen ZF, Yunoki M, Toyoda T, Anzivino M, Lee KS. Hypothermia-induced ischemic tolerance. Ann N Y Acad Sci. 1999. 890:26–41.
12. Schurr A, Payne RS, Tseng MT, Gozal E, Gozal D. Excitotoxic preconditioning elicited by both glutamate and hypoxia and abolished by lactate transport inhibition in rat hippocampal slices. Neurosci Lett. 2001. 307:151–154.
13. Khaspekov L, Shamloo M, Victorov I, Wieloch T. Sublethal in vitro glucose-oxygen deprivation protects cultured hippocampal neurons against a subsequent severe insult. Neuroreport. 1998. 9:1273–1276.
14. Meloni BP, Majda BT, Knuckey NW. Evaluation of preconditioning treatments to protect near-pure cortical neuronal cultures from in vitro ischemia induced acute and delayed neuronal death. Brain Res. 2002. 928:69–75.
15. Liu J, Ginis I, Spatz M, Hallenbeck JM. Hypoxic preconditioning protects cultured neurons against hypoxic stress via TNF-alpha and ceramide. Am J Physiol Cell Physiol. 2000. 278:C144–C153.
16. Rybnikova E, Vataeva L, Tyulkova E, Gluschenko T, Otellin V, Pelto-Huikko M, Samoilov MO. Mild hypoxia preconditioning prevents impairment of passive avoidance learning and suppression of brain NGFI-A expression induced by severe hypoxia. Behav Brain Res. 2005. 160:107–114.
17. Hieber S, Huhn R, Hollmann MW, Weber NC, Preckel B. Hypoxia-inducible factor 1 and related gene products in anaesthetic-induced preconditioning. Eur J Anaesthesiol. 2009. 26:201–206.
18. Blondeau N, Widmann C, Lazdunski M, Heurteaux C. Activation of the nuclear factor-kappaB is a key event in brain tolerance. J Neurosci. 2001. 21:4668–4677.
19. Lee HT, Chang YC, Wang LY, Wang ST, Huang CC, Ho CJ. cAMP response element-binding protein activation in ligation preconditioning in neonatal brain. Ann Neurol. 2004. 56:611–623.
20. Wang X, Deng J, Boyle DW, Zhong J, Lee WH. Potential role of IGF-I in hypoxia tolerance using a rat hypoxic-ischemic model: activation of hypoxia-inducible factor 1alpha. Pediatr Res. 2004. 55:385–394.
21. Kitagawa K, Matsumoto M, Tagaya M, Hata R, Ueda H, Niinobe M, Handa N, Fukunaga R, Kimura K, Mikoshiba K, Kamada T. 'Ischemic tolerance' phenomenon found in the brain. Brain Res. 1990. 528:21–24.
22. Yin XH, Zhang QG, Miao B, Zhang GY. Neuroprotective effects of preconditioning ischaemia on ischaemic brain injury through inhibition of mixed-lineage kinase 3 via NMDA receptor-mediated Akt1 activation. J Neurochem. 2005. 93:1021–1029.
23. Pugliese AM, Latini S, Corradetti R, Pedata F. Brief, repeated, oxygen-glucose deprivation episodes protect neurotransmission from a longer ischemic episode in the in vitro hippocampus: role of adenosine receptors. Br J Pharmacol. 2003. 140:305–314.
24. Arthur PG, Lim SC, Meloni BP, Munns SE, Chan A, Knuckey NW. The protective effect of hypoxic preconditioning on cortical neuronal cultures is associated with increases in the activity of several antioxidant enzymes. Brain Res. 2004. 1017:146–154.
25. Nakajima W, Ishida A, Lange MS, Gabrielson KL, Wilson MA, Martin LJ, Blue ME, Johnston MV. Apoptosis has a prolonged role in the neurodegeneration after hypoxic ischemia in the newborn rat. J Neurosci. 2000. 20:7994–8004.
26. Li YK, Liu GR, Zhou XG, Cai AQ. Experimental hypoxic-ischemic encephalopathy: comparison of apparent diffusion coefficients and proton magnetic resonance spectroscopy. Magn Reson Imaging. 2010. 28:487–494.
27. Hüppi PS, Lazeyras F. Proton magnetic resonance spectroscopy ((1)HMRS) in neonatal brain injury. Pediatr Res. 2001. 49:317–320.
28. da Silva LF, Höefel Filho JR, Anés M, Nunes ML. Prognostic value of 1HMRS in neonatal encephalopathy. Pediatr Neurol. 2006. 34:360–366.
29. Blankenberg FG, Katsikis PD, Storrs RW, Beaulieu C, Spielman D, Chen JY, Naumovski L, Tait JF. Quantitative analysis of apoptotic cell death using proton nuclear magnetic resonance spectroscopy. Blood. 1997. 89:3778–3786.
30. Park SJ, Yoon HS, Lim KH, Lee JH, Kim KS, Pi SY. Early prediction of hypoxic-ischemic brain damage in newbon rats using proton magnetic resonance spectroscopy and neuroprotective effect of EGb 761. J Korean Soc Neonatol. 2001. 8:133–140.