Abstract
Cardiothoracic ratio (CTR), the ratio of cardiac diameter (CD) to thoracic diameter (TD), is a useful screening method to detect cardiomegaly, but is reliable only on posteroanterior chest radiography (chest PA). We performed this cross-sectional 3-phase study to establish reliable CTR from anteroposterior chest radiography (chest AP). First, CDChest PA/CDChest AP ratios were determined at different radiation distances by manipulating chest computed tomography to simulate chest PA and AP. CDChest PA was inferred from multiplying CDChest AP by this ratio. Incorporating this CD and substituting the most recent TDChest PA, we calculated the 'corrected' CTR and compared it with the conventional one in patients who took both the chest radiographies. Finally, its validity was investigated among the critically ill patients who performed portable chest AP. CDChest PA/CDChest AP ratio was {0.00099 × (radiation distance [cm])} + 0.79 (n = 61, r = 1.00, P < 0.001). The corrected CTR was highly correlated with the conventional one (n = 34, difference: 0.00016 ± 0.029; r = 0.92, P < 0.001). It was higher in congestive than non-congestive patients (0.53 ± 0.085; n = 38 vs 0.49 ± 0.061; n = 46, P = 0.006). Its sensitivity and specificity was 61% and 54%. In summary, reliable CTR can be calculated from chest AP with an available previous chest PA. This might help physicians detect congestive cardiomegaly for patients undergoing portable chest AP.
The cardiothoracic ratio (CTR) has been considered as a classic index of cardiac function (1). However, its value has been questioned because echocardiography, radionuclide imaging, angiography, computed tomography (CT), and magnetic resonance imaging can provide more precise information about cardiac function (2-4). Nevertheless, clinicians continue to use the CTR because a quick decision is required under urgent situations, especially in the emergency department (ED) or intensive care unit (ICU). Daily follow-up of chest radiography is still recommended in the ICU (5). New information favoring CTR also has been being reported (6-10).
CTR is calculated by dividing the cardiac diameter (CD) by the thoracic diameter (TD) as measured on posteroanterior chest radiography (chest PA) (1). Because of limited transport or position of patients, portable anteroposterior chest radiography (chest AP) is more frequently performed in the ED or ICU than chest PA. However, chest AP has 3 serious limitations preventing its use for the precise measurement of CTR: 1) radiation entering from the anterior makes the CD appear larger because the heart resides toward the anterior thorax; 2) the shorter distance between the radiation source and the imaging cassette results in a larger image and thus overestimates both the CD and TD; 3) the patients who cannot inhale to maximum or hold their breath because of dyspnea, pain, or unconsciousness make the TD unreliable (Fig. 1). If calculable, assessing the reliable CTR from chest AP would provide clinicians with more information about cardiac function under urgent situations. Thus, we designed a new method for calculating the CTR from chest AP, namely the 'corrected CTR', and evaluated its clinical usefulness.
The basic concept of this study arose from the following question: "Could we not predict the CD and TD on chest PA, although unchecked, with those available on chest AP?"
Because TD measured from chest AP could be overestimated, we substituted TD measured from chest PA, which was performed most recently within the prior 3 yr, to calculate the 'corrected' CTR. Lauder and Milne reported a maximum decrease of 12 mm over 15 yr from the age of 80 yr (11). For people aged 62 yr or older, TD decreased by 3.5 mm at most over 5 yr (12). Thus, we assumed that the TD would change negligibly, by less than 3 mm, over 3 yr.
Both the CDChest PA and CDChest AP depend on the distance between the radiation source and the imaging cassette. While the international standard distance for chest PA is 180 cm, the distance for chest AP is not standardized. In consequence of consultations with radiologists in several hospitals, it usually ranged from 110 to 150 cm. Therefore, using chest CT as a reference, we simulated three distances to perform chest AP (110, 130, and 150 cm) and calculated the CDChest PA/CDChest AP ratio at each. If the ratio is fixed at the given distance, we would be able to predict CDChest PA using CDChest AP and calculate the corrected CTR as follows:
Corrected CTR = [Expected CDChest PA]/[Expected TDChest PA] = [CDChest AP × (CDChest PA/CDChest AP ratio)]/[Most recent TDChest PA]
If this corrected CTR reflects the conventional CTR high enough, corrected CTR of ≥ 0.5 could be defined as cardiomegaly just as for conventional CTR.
A cross-sectional, 3-phase study was conducted retrospectively. In phase 1, by manipulating chest CT to simulate chest PA and AP at different radiation distances, we determined CDChest PA/CDChest AP ratios in terms of the radiation distance. If the ratio is fixed at a specific distance to perform chest AP, we would be able to infer CDChest PA by multiplying CDChest AP by this ratio. In phase 2, by incorporating the relevant CDChest PA/CDChest AP ratio to calculate CD and replacing TD with that on the most recent chest PA, we calculated the 'corrected' CTR and compared it with the conventional one to examine whether the former reflects the latter well. In phase 3, the validity of the corrected CTR was investigated with patients who performed chest AP in ED or ICU.
We included patients aged 25 yr or older to avoid bias caused by bony growth.
All patients who underwent chest AP and chest CT within 24 hr in ED or ICU between January 2005 and December 2009 and had undergone chest PA within the prior 3 yr were included. By manipulating chest CT of these patients to simulate chest PA and AP, we measured CDChest PA and CDChest AP, and determined the CDChest PA/CDChest AP ratio in terms of the radiation distance to perform chest AP. We excluded patients who met the following criteria: thoracic wall or spinal deformity; distances at the carina level from the midline to each lateral rib margin differing by more than 20%, suggesting mediastinal deviation; undetectable internal margins of rib cage; unidentifiable cardiac borders, e.g. pleural effusion or tumor abutting a cardiac border; and thorax rotation of more than 10° on CT.
Chest AP and PA should have been simultaneously performed in a given patient for the corrected CTR to be compared with the conventional one. But it was difficult to select such patients under urgent situations in ED or ICU. From January 2005 to December 2009, instead, we selected other patients who were admitted for minor surgery, dermatological problems, or psychiatric illness without heart or renal failure and had undergone chest PA on admission and portable chest AP within 1 month after admission. The performance of chest PA on admission and chest AP within 1 month after admission in these stable patients could be considered as the simultaneous performance of the two radiographies, thus enabling the comparison between the corrected and conventional CTR (11, 12).
To estimate general applicability of the corrected CTR to all patients taking chest AP regardless of chest CT, we collected patients who received chest AP in ED or ICU on a randomly chosen day of each month during 2009 other than those in phase 1. The severely orthopneic patients could have been excluded in phase 1 for their inability to undergo chest CT impairing the external validity. Of these, patients who had undertaken chest PA within the prior 3 yr were reselected as they had the 'most recent TDChest PA' to calculate corrected CTR with. In these patients, the corrected CTR was validated for diagnosing congestive cardiomegaly with the classic criterion of 0.50. Before utilizing the ultimate clinical diagnoses of the presence or absence of congestive conditions, the authors tested the validity of these clinical diagnoses. For this purpose, we investigated all the echocardiography findings of the eligible patients as available as possible and recorded the diastolic and systolic internal diameter and ejection fraction of the left ventricle for comparison between the clinically diagnosed congestive and non-congestive patients. Finally, we compared the discriminating power of the corrected CTR with that of the 'non-corrected' CTR, which was calculated simply as CDChest AP/TDChest AP without any modification.
Demographic, clinical, laboratory and radiologic data were collected from all patients in phase 1 and 3. To investigate discriminatory power of the corrected CTR, patients were categorized into two subgroups: congestive vs non-congestive. The congestive subgroup included cases with predominantly congestive medical conditions (cardiac or chronic renal failure), cases with combined congestive and non-congestive medical conditions, and surgical cases with underlying congestive medical conditions. The non-congestive subgroup included cases with predominantly non-congestive medical conditions, and surgical cases without underlying congestive medical conditions. We also collected data on other co-morbid conditions such as hypertension, chronic obstructive pulmonary diseases, and diabetes mellitus.
On chest radiography, a vertical line was traced parallel to the vertebral column. The greatest distances from vertical line to each cardiac border were measured, and the sum of them rendered the CD (mm). In addition, the greatest width between the inner surfaces of ribs was defined as the TD (mm).
Two points at the farthest edge of both cardiac borders from the inner surface of contralateral ribs were identified on chest PA (Fig. 2A). The 2 CT images that included these points were then selected using the PiViewSTAR version 5.0 (INFINITT Healthcare Co., Ltd., Seoul, Korea) navigation function (Fig. 2B). All of the CDChest PA and CDChest AP at 3 simulated distances were measured on 1:10 reduction images, respectively (Fig. 3). Chest PA was simulated by placing the CT image in the direction that allowed radiation to penetrate the thorax from behind (Fig. 3A). The radiation then met the anterior thorax at 18 cm distance. Two lines were drawn from the imaginary radiation source to the imaging cassette: one perpendicular to the imaging cassette (SO) and the other a tangent meeting the farthest right cardiac border (SR). Next, the distance between the two points marked by these lines on the imaging cassette was measured (RO), the right half of the CD. To simulate chest AP, we rotated the CT image 180°. We set the distances on reduction images as 11, 13, and 15 cm, respectively (Fig. 3B-D). The right half of the CD for each simulation was measured (O'R'110, O'R'130, and O'R'150). The same was done to measure the left half of the CD. The sum of both halves rendered the CD for each simulation.
We constructed 1:10 reduction images using HanWord 2007 (Hancom, Inc., Seoul, Korea). As the minimum resolving power was 0.25 mm, the maximum error of the real CD would be 2.5 mm. A non-radiologist clinician performed the measurements throughout the analysis to eliminate inter-rater variability and assure the general usage of CTR.
Echocardiography findings taken within 1 month from the chest AP were sought for the newly diagnosed congestive patients. As for those with known congestive conditions and those with no evidence of congestion at all, the most recent echocardiography within 3 yr was recruited. The end diastolic and systolic internal diameter (mm) and ejection fraction (%) of the left ventricle were recorded.
In phase 1, the means, standard deviations, and 95% confidence intervals (CI) of the CDChest PA/CDChest AP ratio at each simulation were calculated. In phase 2, the difference and degree of correlation between the corrected and conventional CTR were calculated. In phase 3, Wilcoxon rank sum test was performed to compare the end diastolic and systolic internal diameter and ejection fraction of the left ventricle on the echocardiography findings between the clinically congestive and non-congestive patients. Then, a one-tailed t-test was used to evaluate whether the corrected CTR of the congestive patient is larger than that of non-congestive patient. On the basis of CTR of ≥ 0.50 for diagnosing congestive cardiomegaly, we calculated the sensitivity, specificity, positive predictive value (PPV) and negative predictive value (NPV) of the corrected CTR. In addition, we drew the receiver operating characteristic (ROC) curves of both the corrected and non-corrected CTR to discriminate congestive conditions. By calculating the area-under-curve (AUC) and P value of each ROC curve, we compared this ability of each CTR.
A P of < 0.05 was considered to be statistically significant for all analyses. IBM PASW Statistics 18.0 (SPSS Inc., Chicago, IL, USA) was used.
A total of 75 patients (41 in ED and 34 in ICU) met the inclusion criteria in phase 1. Of these, 14 were excluded: 4 for distorted thoraces, 7 for undetermined cardiac borders, 1 for undetermined thoracic borders, and 2 for rotated thoraces. Finally, 61 patients (34 in ED and 27 in ICU) were included, and their characteristics are summarized in Table 1.
CDChest PA, CDChest AP, and CDChest PA/CDChest AP ratio for each simulation are shown in Table 2. The 95% CI of the CDChest PA/CDChest AP ratio at given distances varied only by 0.01. In the regression analysis, therefore, the CDChest PA/CDChest AP ratio could be expressed as a function of the radiation distance (cm) as follows:
CDChest PA/CDChest AP ratio = [0.00099 × (radiation distance)] + 0.79 where both the P values of the slope (0.00099) and y-intercept (0.79) were < 0.001. Its coefficient of correlation (r) was 1.00.
Using this formula, we calculated the CDChest PA/CDChest AP ratios at some ranges of distance for further usage (Table 3).
In phase 2, a total of 32 patients were included (mean age, 60 ± 16 yr; male:female, 22:10). They comprised 22 (69%) patients with neuropsychiatric disorders, 7 (22%) with otolaryngologic disorders, 2 (6%) with ophthalmologic disorders, and 1 (3%) with cosmetic problem.
To calculate the corrected CTR, the radiation distance to perform chest AP was mandatory. In clinical practice, there was some variation in radiation distance whenever performing portable chest AP. This distance was repeatedly measured at the time of the present study. As the result, they clustered around 140 cm (range, 132-147 cm), ranging between 130-140 and 141-150 cm. Thus, we adopted the rounded value of 0.925 at 140 cm as a 'uniform' CDChest PA/CDChest AP ratio hereafter to calculate the expected CD (Table 3). Using this ratio of 0.925, the maximum error of the CDChest PA/CDChest AP ratio would be 0.005, because the ratio ranges 0.92-0.93 with the radiation distance ranging 132-147 cm (Table 3).
The difference between the conventional and corrected CTR was 0.00016 ± 0.029 (range, -0.074 to 0.056) with 95% CI of -0.011 to 0.010. This difference was considered to be clinically negligible. The coefficient of correlation (r) was 0.92 (P < 0.001).
On the 12 randomly selected days, 607 patients visited ED and 190 stayed in ICU. Of these, 47 (7.7%) in ED and 99 (52.1%) in ICU were more than 25 yr-old and underwent chest AP. The characteristics of these 146 patients are shown in Table 4. Chest PA had been performed within the prior 3 yr in 22 of the 47 patients in ED and 65 of the 99 patients in ICU. Three of these were excluded: 2 for undetermined cardiac borders, and 1 for undetermined thoracic borders. Therefore, the remaining 84 patients (21 in ED and 63 in ICU) were included to evaluate the validity of corrected CTR.
Among these, echocardiography reports were available from 35 (18 congestive and 17 non-congestive) patients. Wilcoxon rank sum test revealed that the end diastolic and systolic internal diameters of the left ventricle on echocardiography were significantly larger in the clinically congestive than in clinically non-congestive patients, while the results of the ejection fractions were the opposite. The medians of these variables were as follows, respectively, with the inter-quartile ranges (IQR) in parentheses: 50 mm (13 mm) vs 45 mm (11 mm), P = 0.017; 34 mm (14 mm) vs 29 mm (11 mm), P = 0.006; and 51.5% (20%) vs 66% (7%), P < 0.001.
The corrected CTR of congestive cases was higher than that of non-congestive cases (0.53 ± 0.085; n = 38 vs 0.49 ± 0.061; n = 46, P = 0.006). For diagnosing congestive cardiomegaly, the sensitivity, specificity, PPV, and NPV of the corrected CTR was 61% (95% CI, 43%-76%), 54% (39%-69%), 52% (37%-67%), and 63% (46%-77%), respectively.
ROC curves revealed that the discriminating power of the corrected CTR was shown to be statistically significant, while that of the non-corrected CTR was not. The AUC and P value of each CTR was: 0.649 (95% CI, 0.532-0.766), P = 0.019 vs 0.621 (95% CI, 0.50-0.741), P = 0.058 (Fig. 4).
In the present study, we deduced relatively fixed CDChest PA/CDChest AP ratios at several ranges of radiation distance by means of the regression analysis. In addition, by incorporating the relevant ratio to calculate the expected CDChest PA and substituting the most recent TD on CPA for the expected TDChest PA, we calculated the corrected CTR, which showed high degree of coincidence with the conventional one. Finally, the corrected CTR was found to be higher in the congestive patients than the non-congestive patients. It also showed superiority to the 'non-corrected' CTR in discriminating congestive conditions.
In 3 previous studies, to our knowledge, investigators tried to calculate CTR from chest AP (13-15). However, there were several limitations in these studies: cases showing partial inhalation were excluded in one study (13), only the patients who could easily undergo chest PA were included in another (14), and phantoms were used in the other (15). Additionally, the effect of radiation distances on CTR was considered only in one (15). Despite these limitations, CDChest PA/CDChest AP ratio at 150 cm was 0.93 in a previous study (13), while that was 0.94 in this study. This difference of 0.01 seems to be clinically acceptable.
In a previous study using echocardiography as a reference method, the sensitivity and specificity of the CTR of ≥ 0.5 to detect left ventricular enlargement (end-diastolic volume ≥ 102 mL/m2) were 88% and 41%, while those to detect reduced ejection fraction ( ≤ 50%) were 86% and 35%, respectively (16). In the present study, the sensitivity and specificity of corrected CTR to diagnose congestive cardiomegaly with the criterion of ≥ 0.5 was 61% (95% CI, 43%-76%) and 54% (95% CI, 39%-69%), showing lower sensitivity and slightly higher specificity. This discrepancy could be explained by the different criteria to define cardiomegaly: left ventricular enlargement or ejection fraction measured on echocardiography vs congestive status determined clinically.
Recently, a study showed that CD was significantly correlated with the left ventricular size, the well-known predictor of cardiovascular morbidity and mortality, while CTR was not (r = 0.52, P = 0.001 vs r = 0.12, P = 0.51) (2). We think that the CDChest PA/CDChest AP ratio calculated from this study could be used to infer CDChest PA with CDChest AP. Furthermore, knowledge emphasizing the utility of CTR is still reported: CTR ≥ 0.42 is associated with higher mortality in patients undergoing coronary angiography (6); Baseline CTR > 0.50 is associated with increased mortality and morbidity in ambulatory patients with chronic heart failure (7); CTR is an important prognostic tool in heart failure patients who are candidates for heart transplantation (8); CTR is an important predictor of left ventricular systolic dysfunction (9); CTR is related to sudden cardiac death (10) etc. Utilizing the concept of corrected CTR, these might be applied to more patients performing chest AP.
Question may arise about the sample size. We determined the number of patients to let the error of α and β less than 0.05 and 0.20, respectively. When the coefficient of correlation (r) is 0.8, nine or more samples are required (17). In phase 1 and 2, r was 1.00 and 0.92, respectively, and 61 and 32 patients for each phase were sufficient. Regarding phase 3, we used phase 1 as a pilot study to calculate the sample size. The mean difference of corrected CTR between congestive and non-congestive patients in phase 1 was 0.03 (0.52 ± 0.042; n = 15 vs 0.49 ± 0.058; n = 46, P = 0.038). The pooled standard deviation was 0.055. Applying these, the minimal sample size per group for comparing two means was 40 (17). Phase 3 just met this criterion.
This study has some limitations. First, to compare the corrected CTR with the conventional one, we chose patients who had chest PA and AP within one month (phase 2). With this indirect method, we risked overestimating the difference and underestimating the degree of correlation. Nevertheless, the result showed negligible difference (0.00004 ± 0.029) and high correlation (r = 0.92). We believe that the actual values, if calculable, would have shown even favorable results. More basically, it could be pointed out that CTR is not the only index of congestion on chest radiography. We targeted CTR for its simplicity and ability to differentiate congestive cardiomegaly from non-cardiogenic respiratory distress syndrome. Finally, by limiting the time for a previous chest PA within the prior 3 yr, corrected CTR could be calculated from 84 (57.5%) of 146 patients in phase 3. It might have been expanded without greatly affecting the accuracy by increasing the interval somewhat longer (12).
In conclusion, the reliable CTR can be calculated from portable chest AP, by multiplying CDChest AP by the relevant CDChest PA/CDChest AP ratio and being divided by the most recent TDChest PA. The corrected CTR of ≥ 0.5 might be an easy-to-use index to detect congestive cardiomegaly among patients being unable to undergo chest PA.
Figures and Tables
![]() | Fig. 1Three pitfalls which prevent antero-posterior chest radiograph from being used to measure the cardiothoracic ratio: (A) The effect of reverse position; (B) The effect of shorter distance from radiation source; (C) The effect of not fully inflated thorax. |
![]() | Fig. 2Identification of the images that include the furthest 2 cardiac borders on postero-anterior chest radiography (A) and the cross-sectional image using the navigation function intrinsic to the picture archiving system (B). Image number 32 includes right cardiac border, here. |
![]() | Fig. 3Cross-sectional images simulating posteroanterior (A) and anteroposterior chest radiographies (B-D). SO is perpendicular to thorax and SR is tangent at the right cardiac border S, radiation source; O, R, points on the imaging cassette; numbers, radiation distances (cm)). |
![]() | Fig. 4Receiver operating characteristic (ROC) curves of the corrected and non-corrected cardiothoracic ratio (CTR) from anteroposterior chest radiography to discriminate congestive conditions. AUC, area-under-curve; CI, confidence interval. |
Table 2
Cardiac diameters on posteroanterior chest radiography and 3 simulated anteroposterior chest radiographies, and the CDChest PA/CDChest PA ratio at each distance
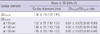
ACKNOWLEDGMENTS
We thank Gil Joon Suh, MD, Joong Eui Rhee, MD, and Kilsoo Yie, MD for their review, criticism, and encouragement of the manuscript.
AUTHOR SUMMARY
Calculation of the Cardiothoracic Ratio from Portable Anteroposterior Chest Radiography
Sung Bin Chon, Won Sup Oh, Jun Hwi Cho, Sam Soo Kim and Seung-Joon Lee
Cardiothoracic ratio (CTR), the ratio of cardiac diameter (CD) to thoracic diameter (TD), is a useful screening method to detect cardiomegaly, but is reliable only on posteroanterior chest radiography (chest PA). This study was performed to establish reliable CTR from anteroposterior chest radiography (chest AP). CDChest PA/CDChest AP ratios were determined at different radiation distances by manipulating chest computed tomography to simulate chest PA and AP. Reliable CDChest PA was inferable from multiplying CDChest AP by these ratios. The 'corrected' CTR was highly correlated with the conventional one. This study demonstrates that reliable CTR is calculable from chest AP with a previous chest PA.
References
1. Danzer CS. The cardiothoracic ratio. Am J Med Sci. 1919. 157:513–554.
2. Schlett CL, Kwait DC, Mahabadi AA, Bamberg F, O'Donnell CJ, Fox CS, Hoffmann U. Simple area-based measurement for multidetector computed tomography to predict left ventricular size. Eur Radiol. 2010. 20:1590–1596.
3. Clark AL, Coats AJ. Unreliability of cardiothoracic ratio as a marker of left ventricular impairment: comparison with radionuclide ventriculography and echocardiography. Postgrad Med J. 2000. 76:289–291.
4. Philbin EF, Garg R, Danisa K, Denny M, Gosselin G, Hassapoyannes C, Horney A, Johnstone DE, Lang RM, Ramanathan K, Safford RE, Sarma RJ, Weiss R, Williford WO, Fleg JL. Digitalis Investigation Group. The relationship between cardiothoracic ratio and left ventricular ejection fraction in congestive heart failure. Arch Intern Med. 1998. 158:501–506.
5. Dakin J, Griffiths M. The pulmonary physician in critical care 1: pulmonary investigations for acute respiratory failure. Thorax. 2002. 57:79–85.
6. Zaman MJ, Sanders J, Crook AM, Feder G, Shipley M, Timmis A, Hemingway H. Cardiothoracic ratio within the "normal" range independently predicts mortality in patients undergoing coronary angiography. Heart. 2007. 93:491–494.
7. Giamouzis G, Sui X, Love TE, Butler J, Young JB, Ahmed A. A propensity-matched study of the association of cardiothoracic ratio with morbidity and mortality in chronic heart failure. Am J Cardiol. 2008. 101:343–347.
8. Berman M, Aravot D, Ben-Gal T, Sahar G, Sagie A, Vidne B. Cardiothoracic ratio: important prognostic tool in heart failure patients who are candidates for heart transplantation. Transplant Proc. 2000. 32:727–728.
9. Shah S, Davies MK, Cartwright D, Nightingale P. Management of chronic heart failure in the community: role of a hospital based open access heart failure service. Heart. 2004. 90:755–759.
10. Nolan J, Batin PD, Andrews R, Lindsay SJ, Brooksby P, Mullen M, Baig W, Flapan AD, Cowley A, Prescott RJ, Neilson JM, Fox KA. Prospective study of heart rate variability and mortality in chronic heart failure results of the United Kingdom heart failure evaluation and assessment of risk trial (UK-Heart). Circulation. 1998. 98:1510–1516.
11. Lauder IJ, Milne JS. Longitudinal study of heart size in older people. Br Heart J. 1976. 38:1286–1290.
12. Potter JF, Elahi D, Tobin JD, Andres R. Effect of aging on the cardiothoracic ratio of men. J Am Geriatr Soc. 1982. 30:404–409.
13. Kabala JE, Wilde P. The measurement of heart size in the antero-posterior chest radiograph. Br J Radiol. 1987. 60:981–986.
14. van der Jagt EJ, Smits HJ. Cardiac size in the supine chest film. Eur J Radiol. 1992. 14:173–177.
15. Milne EN, Burnett K, Aufrichtig D, McMillan J, Imray TJ. Assessment of cardiac size on portable chest films. J Thorac Imaging. 1988. 3:64–72.
16. Hammermeister KE, Chikos PM, Fisher L, Dodge HT. Relationship of cardiothoracic ratio and plain film heart volume to late survival. Circulation. 1979. 59:89–95.
17. Ahn YO, Yoo KY, Park BJ. Manual for medical statistics. 1996. Seoul: Seoul National University Press;321–322.