Abstract
Glycine and γ-aminobutyric acid (GABA) are localized and released by the same interneurons in the spinal cord. Although the effects of glycine and GABA on analgesia are well known, little is known about the effect of GABA in strychnine-induced hyperalgesia. To investigate the effect of GABA and the role of the glycine receptor in thermal hyperalgesia, we designed an experiment involving the injection of muscimol (a GABAA receptor agonist), baclofen (a GABAB receptor agonist) or glycine with strychnine (strychnine sensitive glycine receptor antagonist). Glycine, muscimol, or baclofen with strychnine was injected into the cisterna magna or lumbar subarachnoidal spaces of mice. The effects of treatment on strychnine-induced heat hyperalgesia were observed using the pain threshold index via the hot plate test. The dosages of experimental drugs and strychnine we chose had no effects on motor behavior in conscious mice. Intracisternal or intrathecal administration of strychnine produced thermal hyperalgesia in mice. Glycine antagonize the effects of strychnine, whereas, muscimol or baclofen does not. Our results indicate that glycine has anti-thermal hyperalgesic properties in vivo; and GABA receptor agonists may lack the binding abilities of glycine receptor antagonists with their sites in the central nervous system.
Gamma-aminobutyric acid (GABA) and glycine are important inhibitory neurotransmitters in the mammalian central nervous system (CNS) (1). GABA and glycine are co-localized in interneurons in spinal laminae I-III (2) and are released from the same interneurons (3).
The temporary removal of spinal glycinergic inhibition by administration of intrathecal strychnine (strychnine sensitive glycine receptor antagonist) disturbs sensations and produces an effect resembling neuropathic pain. Strychnine treatments are used to investigate potential analgesics (4), particularly analgesics that directly or indirectly enhance the glycinergic mediation of nociceptive information (5). We hypothesized that if GABA and glycine are co-released from the same interneurons and GABA agonists act at GABA and glycine receptors to modulate nociceptive transmission via distinct but complementary mechanisms, GABA agonists might inhibit strychnine-induced nociception. In previous papers, we demonstrated that administration of intrathecal glycine, taurine, and muscimol antagonized bicuculline-induced thermal hyperalgesia (6) and intrathecal muscimol did not antagonize strychnine-induced allodynia or thermal hyperalgesia (7) in vivo. However, the nature of the interaction between glycine and GABA during sensory modulation remains unclear.
We undertook the present study with following objectives: first, to determine whether central administration of strychnine produce thermal hyperalgesia; second, to determine whether muscimol (GABAA receptor agonist), baclofen (GABAB receptor agonist), or glycine antagonizes strychnine-induced thermal hyperalgesia.
This study was conducted after obtaining approval from the Animal Care Committee of Korea University and in accordance with the guidelines of the Ethics Committee of the International Association for the Study of Pain. Male ICR mice weighing 23-27 g were housed one per cage before experiments in an environmentally controlled room (22℃ ± 2℃, relative humidity 55% ± 5%) under a 12:12 hr light cycle (lights on at 7:00 am) with free access to food and water.
Solutions were prepared in artificial cerebrospinal fluid (aCSF) containing (in mM/L): 124 NaCl, 26 NaHCO3, 1.25 NaH2PO4, 2.5 KCl, 2 MgCl2, 2 CaCl2, and 10 dextrose. The pH of the solution was 7.3-7.4 and its average osmolality was ~300 mOsm. Drugs were dissolved in sterile saline on experimental days and kept on ice until used. Immediately prior to use, drugs were diluted to the required concentrations with aCSF. Muscimol, baclofen, and glycine were purchased from Sigma (St. Louis, MO, USA) and sevoflurane was purchased from Abbott Laboratories (Queenborough, Kent, UK).
Anesthesia was induced using 4% sevoflurane in air in a 500 mL induction chamber. A rubber facemask was used for anesthetic maintenance (sevoflurane 4% through a Mapleson D circuit) and to immobilize heads. In addition, mice were placed in a holder molded from modeling clay to fit the body. A space was molded in the posterior neck area to allow the region between the occiput and atlas to be palpated and to enable the injection to be made. Intracisternal injections were performed using a 25 µL Hamilton syringe (Hamilton Co., NV, USA) attached to a 27G stainless steel needle, the tip of which was modified with a stopper to ensure an insertion depth of 2.4 mm. The needle was carefully advanced to the cisterna magna and after the injection was made the syringe was held in position for a few seconds before gradually being removed to avoid drug outflow along the needle tract. Injection sites were verified by injecting a similar volume of 1% methylene blue solution and confirming the distribution of dye in the cisternal space. Throughout the experiment, the dye was observed throughout the cisterna magna and reached the occipital surface of the brain and the upper cervical portion of the spinal cord. The experimenter was trained using dye injections prior to beginning the experiments and achieved an accuracy of > 98% in test mice. One experimenter performed all procedure. The entire injection procedure, including anesthesia, took about less than three minutes per animal. Spontaneous face scratching, backward folding of the ears, eye squinting or markedly increased responsiveness to light touch in trigeminal areas such as head withdrawal, intense face scratching were observed after injection of strychnine.
All intrathecal injections were made at the L5-L6 intervertebral space using a 30 gauge 0.5 inch needle mounted on a 25 µL Hamilton syringe, as previously described by Hylden and Wilcox (8). Injection sites were verified by injecting a similar volume of 1% methylene blue solution and determining the distribution of the dye in the spinal cord. The dye was distributed rostrally and caudally but over a short distance (less than 1 cm) and no dye was found in the brain in all animals. The experimenter was similarly trained using dye prior to the experiments and achieved an accuracy of > 95% in test mice. One experimenter performed all procedure. The entire intrathecal injection procedure, including handling, also took about less than three minutes per animal. Spontaneous tail biting, tail flick or light touch-evoked allodynia of the hind feet and tail were observed after injecting mice with strychnine.
To examine the effects of different strychnine doses, 5 µL of strychnine at concentrations of 50 (0.083575 µg), 100 (0.16715 µg), 200 (0.3344 µg), or 400 µM (0.6688 µg) were injected intracisternally or intrathecally. Mice that received > 0.08 µg showed allodynia. Two of the four mice treated with 0.08 µg of strychnine failed to respond to a light touch. On the other hand, 0.33 µg strychnine induced touch-evoked allodynia in a predictable manner. One of six mice treated with strychnine 0.33 µg intracisternally showed a dyskinetic behavior or four of the four mice treated with strychnine 0.66 µg intracisternally showed a convulsive behavior (body rollover, loss of postural control, falling, motor arrest with a myoclonic jerk, frequent dyskinesia) (9). Accordingly, we used 0.16 µg of strychnine to induce pain in all subsequent experiments.
To determine muscimol doses, we injected 5 µL at concentrations of 0.025 (0.0142625 µg), 0.05 (0.028525 µg), 0.25 (0.142625 µg), 0.5 (0.28525 µg), 1 (0.5705) or 5 (2.8525 µg) mM. Mice that received 0.28 µg intracisternally were sedated for more than 4 min or showed intermittent dyskinetic hyperactivity; we were unable to perform the hot plate test on these mice. Mice administered 2.8 µg of muscimol intrathecally showed paresis of both hindlimbs for more than 2 hr. Finally, we used muscimol doses of 0.01, 0.02, and 0.1 µg for the subsequent experimental group.
To determine baclofen doses, we injected 5 µL at concentrations of 0.01 (0.010685 µg), 0.05 (0.053425 µg), 0.1 (0.10685 µg), or 1 (1.0685 µg) mM. Mice that received 0.05 µg intracisternally were sedated for more than 4 min; we were unable to perform the hot plate test on these mice. Mice administered 1.06 µg of baclofen intrathecally showed paresis of both hindlimbs for more than 1 hr. Finally, we used baclofen doses of 0.001, 0.002, and 0.01 µg for the subsequent experimental group.
Similarly, preliminary experiments indicated that analgesic doses of 25 µg for glycine were optimal for both intracisternal and intrathecal injections. The dosages of glycine used had no effects on motor behavior in conscious mice.
All experiments were blinded and randomized. The experimenter who injected the drug and the observer were unaware of treatment details. Drug and control solutions were injected in a random, computer-generated order (http://www.randomizer.org). Each animal was subjected to one treatment only. Hot plate test sessions were conducted for 40 min after drug injections. Experiments were conducted between 9:00 am and 16:00 pm in a quiet room.
Control mice were administered 5 µL of strychnine (the strychnine groups; n = 8) or aCSF vehicle (the vehicle controls; n = 8) only. Experimental groups were divided randomly into subgroups, namely, vehicle control (n = 8); glycine (5, 10, 25 µg intracisternally or intrathecally; n = 8, per dosage); muscimol (0.01, 0.02, 0.1 µg intracisternally or intrathecally; n = 8, per dosage); and baclofen (0.001, 0.002, 0.01 µg intracisternally or intrathecally; n = 8, per dosage). Each mouse in the experimental groups received strychnine along with one of the drugs listed above. In experiments exploring the combined effects of the drugs, the drugs were co-administered intracisternally or intrathecally to ensure co-localization and to minimize injection volumes. After injections, the mice were placed on a clear glass plate for the hot plate test. The timing of the hot plate test was started after completion of injections.
To assess whether drugs induced sedation or motor impairment, a naïve observer utilized a five-point scale to evaluate posture 5 min after drug injection (10). The scale was as follows: 0 = normal posture, rearing and grooming; 1 = moderate atonia and ataxia; 2 = weight support, but severe ataxia; 3 = muscle tone but not weight support and 4 = flaccid atonia, fully immobilized. The level of sedation of each mouse was assessed by testing the animal's response to passive head movement on stimulation of the vibrissae (11). The scale was as follows: 0 = awake; active exploring, brisk head response; 1 = slightly drowsy, not actively exploring but easily stimulated by manipulating head or vibrissae; 2 = drowsy, responding only to vigorous manipulation of head or vibrissae; 3 = unresponsive to head manipulation. The animals were observed for 45 min after drug injection.
In a rotarod test, the ability of mice to stay on a rotating rod was used to assess motor performance by a rotarod. The rotarod consisted of a rotating rod (2.8 cm diameter) and individual compartments for each mouse. Mice were selected for their ability to remain on the rod (5 rpm) for two consecutive 5 min trials separated by a 5 min rest period. For all experiments two control trials obtained 6 min apart preceded the drug injection. Each mouse was then given trials at 6 min-intervals after the drug injection. If a mouse fell before 5 min, his failure time was recorded and the results were averaged to obtain a single value for each group.
Mice were allowed to acclimate within an acrylic enclosure on a clear glass plate before each experiment. A radiant heat source was then focused onto the plantar surface of a hindpaw. The test involved placing a mouse on a hot plate maintained at 55℃ and recording the time taken to first response (foot licking, jumping, or rapidly removing paws), as described by Minami et al. (12). A timer stopped automatically when withdrawal of the paw was detected by a photodetector (a cut-off value of 30 sec was used to prevent tissue damage). Response times were measured twice at 3 min intervals and mean values were recorded as pain thresholds. Basal pain thresholds were measured before drug administration (time = 0). Responses were observed at 5, 15, 25, 35 and 45 min after drug injection. These times were chosen based on the results of preliminary studies, which showed that the hot plate response peaked between 5 and 25 min after injecting strychnine intracisternally. In the preliminary study, we also knew that the intrathecal strychnine 0.3 µg showed a significant hot plate response for 60 min after injection (7). In terms of drug localization, we believe that the intrathecal route is better than the intracisternal route because drug dissipation is probably the main cause of the transient effect in mobile animals, making it more likely for the drugs to diffuse to the brainstem and more difficult to localize their actions on the spinal cord. Therefore we chose a dose of strychnine 0.16 µg and an observation time of 45 min after injection for the purpose of comparison between the intracisternal and intrathecal effect of drug in the present study.
Behavioral results were analyzed using the binomial test. Hot plate test results were analyzed by ANOVA. Post hoc analysis was performed using Dunn's test or Holm-Sidak methods. Student's two-tailed test was used to compare two means. The level of significance was set at 5% (P < 0.05). Data are presented as means ± SEM. Statistical analysis was performed using SigmaStat 3.0 (Systat Software Inc., Chicago, IL, USA).
The behavioral effects of glycine, muscimol or baclofen at the injected doses were studied in an open field chamber. All mice scored 0 (0 = normal posture, rearing and grooming; see methods) during this test. Sedation scores of more than 1 (1 = slightly drowsy, not actively exploring but easily stimulated by manipulating head or vibrissae; see methods) were not observed in any mice.
The time fell from the rod of conscious mice was not affected by aCSF, glycine, muscimol, or baclofen (P > 0.05). The strychnine groups exhibited no effects on the time fell from the rod compared with aCSF group (P > 0.05) (Table 1).
The basal latencies of all conscious mice as observed by hot plate tests were similar before drug injection. Mice that received intracisternal glycine (25 µg) alone had a higher HPPI at 15 min than at baseline (time = 0) and than vehicle controls at 15 min (P < 0.05). When given alone, intracisternal or intrathecal muscimol or baclofen had no effect on the pain threshold index in the hot plate test at the doses tested (P > 0.05 vs vehicle controls) (data not shown).
Intracisternal strychnine decreased the HPPI from 5 to 25 min (P < 0.05 vs vehicle controls). Intrathecal strychnine decreased the HPPI from 5 to 45 min (P < 0.05 vs vehicle controls). There were significant differences of the HPPI at 5 to 25 min and the baseline value (time = 0) in the intrathecal strychnine group (P < 0.05 vs baseline, time = 0) (Fig. 1).
In drug + strychnine groups, glycine (intracisternally 25 µg or intrathecally 25 µg) increased the HPPI (P < 0.05 vs strychnine + aCSF group) (Fig. 2). In drug + strychnine groups, intracisternal or intrathecal muscimol or baclofen did not increase the HPPI (Figs. 3, 4).
In the drug + strychnine group, intracisternal glycine (25 µg) increased the HPPI at 15 min (P < 0.05 vs baseline, time = 0) (Fig. 2A).
The present study demonstrates that glycine receptor antagonists produce thermal hyperalgesia, confirming the hypothesis that the loss of endogenous glycine tone leads to hyperalgesia. Muscimol or baclofen was ineffective to reduce strychnine-induced thermal hyperalgesia. These results suggest that GABA lacks an antinociceptive effect on the strychnine induced thermal hyperalgesia and an interaction between GABA and glycine receptors was insufficient to regulate anti-thermal hyperalgesia.
Glycine is important in the spinal processing of innocuous tactile information (13). Glycine is a selective modulator of non-nociceptive input and the blockade of spinal glycinergic receptors with strychnine yields allodynia. Therefore, previous studies have focused on strychnine-induced mechanical allodynia. Allodynia is triggered by input from Aβ fibers, rather than abnormally sensitized C-fibers (14). In contrast, hyperalgesia arises from a decrease in the threshold of activation of nociceptive C-fibers (15). According to previous studies, the loss of spinal strychnine sensitive inhibition augments development of thermal hyperalgesia by chronic nerve compression (16) and spinal glycine produces analgesia in thermal nociception (17). In the present study, we demonstrated that central strychnine produces thermal hyperalgesia of hindfoot, which supports the hypothesis that the loss of glycine tone leads to not only allodynia but also hyperalgesia.
In previous studies, intracerebroventricular or intrathecal injections of glycine significantly increased the pain threshold index in the hot plate test for 45 min after injection (17). We observed slightly shorter duration of thermal antinociception, but we administered different doses of glycine than those used in the previous study. In our study we used 25 µg of glycine, while the previous study used 100 µg for analgesia. In the previous study, the researchers did not observe any abnormal behaviors such as sedation or dyskinesia, and they performed a motor test 10 min after injection, whereas we observed the sedative effect of intracisternal glycine for more than 4 min if we injected more than 25 µg in our preliminary experiment. We believe that the amounts of amino acids used in our study better allow experimenters to blindly assess heat hyperalgesia of the hindfoot after drug injection. The observed differences may be due to the different drug administration routes used as well as parameters checked. In previous studies, the drugs were injected intracerebroventricularly (17), whereas we injected drugs intracisternally. As a wide-spread inhibitory neurotransmitter, glycine invariably affects normal behavior, and therefore we think it is prudent to quantify glycine potency in producing motoric effects using the routes of administration used for the analgesia test. We observed that intracisternal, and not intrathecal, injection of glycine induced sedative effects according to the doses injected in preliminary tests (data not shown). Taken together, glycine and glycine receptors play important roles in anti-hyperalgesic effects and these effects continue for less than one hour.
GABA is densely localized in the spinal cord, where it appears to modulate both nociceptive and non-nociceptive neurotransmission. GABA and glycine are co-localized in interneurons in lamina I-III of the dorsal horn. GABAA agonists such as muscimol and GABAB agonists like baclofen, when given intrathecally, significantly increase response latency in the tail flick and hot plate tests (18, 19). If glycine and GABA are released together into the synapse upon stimulation and if GABA and glycine affect distinct but complimentary inhibition of input in the spinal cord, then the pain arising from the blockade of spinal glycine receptors should be attenuated by the concurrent activation of GABA receptors. However, in the present study, we could not demonstrate that muscimol or baclofen, at a dose that does not affect the basal hot plate response, antagonizes strychnine-induced hyperalgesic response. These findings are not in agreement with our hypothesis that GABA and glycine are co-localized and modulate nociceptive transmission. Our findings are in agreement with previous studies (20, 21). However, contrary to our results, Balerio and Rubio reported that intraperitoneal baclofen (1-3 mg/kg, rat) elicits a dose-dependent antinociceptive effect during the hot plate test (22). Chung et al. (23) showed that intracerebroventricular baclofen (5-10 ng) reduces the behavioral hyperalgesic response induced by the formalin test in mice. Hammond and Drower reported that intrathecal muscimol (0.25 µg) or baclofen (1 µg) increases tail flick or hot plate latencies in mice, respectively (18). This discrepancy may be due differences in pain models, different drug administration sites or to different doses. We used a strychnine-induced hyperalgesia model of the hot plate test and administered muscimol 0.1 µg or baclofen 0.01 µg at their maximum doses respectively. We assume that in an experimental pain model like the one used in the present study, in the absence of nerve injury or inflammation, GABA receptors do not contribute significantly to such modulation. Possible explanations of this difference may include formalin-induced second phase pain that resulted from activation of central sensitized neurons due to peripheral inflammation, while the hot plate test assesses pain reactions involving higher brain centers that are affected by both motor and emotional components. We believe that different nociception causes different pain and that the analgesic mechanisms of drugs are also different.
We did not observe any animal showing motor impairment or sedative effects after drug injection. According to previous studies (18, 20, 24), muscimol produced motor weakness at analgesic doses ranges and baclofen impaired performance on the rotarod apparatus in the antinociceptive, antiallodynic, and antihyperalgesic dose ranges, suggesting that motor dysfunction may have impaired withdrawal from the evocative stimuli, thereby mimicking antinociception, antiallodynia, and antihyperalgesia. Therefore, the lack of antihyperalgesic effects of GABA agonists at doses producing motor dysfunction is consistent with the limited effectiveness of them observed in many clinical studies of neuropathic pain. We can not suggest that the reason of the lack of antihyperalgesic effects of GABA agonists is resulted from only the lack of interaction with glycine receptor because of we did not perform an experiment of co-administration of glycine and GABA agonist to determine the pharmacological interaction of two receptors.
In summary, glycine reduces strychnine-induced thermal hyperalgesia responses in vivo. The inhibitory effects of muscimol or baclofen on strychnine-induced thermal hyperalgesia are not observed. Thus, GABA receptor agonists may lack the binding abilities of glycine receptor antagonists with their sites in the central nervous system.
Figures and Tables
Fig. 1
Effect of strychnine or artificial cerebrospinal fluid (aCSF) on hot plate test pain index (HPPI). Strychnine (0.16715 µg) or aCSF was injected intracisternally (IC, A) or intrathecally (IT, B). Data are expressed as mean ± SEM. *P < 0.05 vs vehicle (aCSF) controls by the t-test; †P < 0.05 vs basal time point (time = 0) by repeated measures ANOVA (n = 8 mice per group).
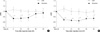
Fig. 2
Effect of glycine on HPPI in strychnine treated mice. Glycine (5, 10, and 25 µg) with strychnine (0. 16715 µg) was injected intracisternally (IC, A) or intrathecally (IT, B). Data are expressed as mean ± SEMs. *P < 0.05 vs the strychnine + aCSF group by two-way ANOVA, Dunn's test, or the Holm-Sidak method (n = 8 mice per group). †P < 0.05 vs. basal time point (time = 0) by repeated measures ANOVA (n = 8 mice per group).
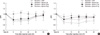
Fig. 3
Effect of muscimol on HPPI in strychnine treated mice. Muscimol (0.01, 0.02, or 0.1 µg) with strychnine (0.16715 µg) was injected intracisternally (IC, A) or intrathecally (IT, B). Data are expressed as means ± SEMs (n = 8 mice per group).
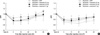
AUTHOR SUMMARY
Pharmacology of Intracisternal or Intrathecal Glycine, Muscimol, and Baclofen in Strychnine-induced Thermal Hyperalgesia of Mice
Il Ok Lee, Jin Kuk Son, Eui-Sung Lim and Yeon-Soo Kim
The present study demonstrates that glycine receptor antagonists produce thermal hyperalgesia, confirming the hypothesis that the loss of endogenous glycine tone leads to hyperalgesia. Muscimol or baclofen was ineffective to reduce strychnine-induced thermal hyperalgesia. These results suggest that GABA lacks an antinociceptive effect on the strychnine induced thermal hyperalgesia, and GABA receptor agonists may lack the binding abilities of glycine receptors in the central nervous system.
References
1. Curtis DR, Watkins JC. The excitation and depression of spinal neurones by structurally related amino acids. J Neurochem. 1960. 6:117–141.
2. Mitchell K, Spike RC, Todd AJ. An immunocytochemical study of glycine receptor and GABA in laminae I-III of rat spinal dorsal horn. J Neurosci. 1993. 13:2371–2381.
3. Jonas P, Bischofberger J, Sandkühler J. Corelease of two fast neurotransmitters at a central synapse. Science. 1998. 281:419–424.
4. Chen Y, Dai TJ, Zeng YM. Strychnine-sensitive glycine receptors mediate the analgesic but not hypnotic effects of emulsified volatile anesthetics. Pharmacology. 2007. 80:151–157.
5. Lynch JW, Callister RJ. Glycine receptors: a new therapeutic target in pain pathways. Curr Opin Investig Drugs. 2006. 7:48–53.
6. Lee IO, Lim ES. Intracisternal or intrathecal glycine, taurine, or muscimol inhibit bicuculline-induced allodynia and thermal hyperalgesia in mice. Acta Pharmacol Sin. 2010. 31:907–914.
7. Lim ES, Lee IO. Effect of intrathecal glycine and related amino acids on the allodynia and hyperalgesic action of strychnine or bicuculline in mice. Korean J Anesthesiol. 2010. 58:76–86.
8. Hylden JL, Wilcox GL. Intrathecal morphine in mice: a new technique. Eur J Pharmacol. 1980. 67:313–316.
9. Jobe PC, Dailey JW. Neurobiology of seizure predisposition in the genetically epilepsy prone rat. Proc West Pharmacol Soc. 1991. 34:223–225.
10. Kim HK, Park SK, Zhou JL, Taglialatela G, Chung K, Coggeshall RE, Chung JM. Reactive oxygen species (ROS) play an important role in a rat model of neuropathic pain. Pain. 2004. 111:116–124.
11. Ewen A, Archer DP, Samanani N, Roth SH. Hyperalgesia during sedation: effects of barbiturates and propofol in the rat. Can J Anaesth. 1995. 42:532–540.
12. Minami T, Uda R, Horiguchi S, Ito S, Hyodo M, Hayaishi O. Allodynia evoked by intrathecal administration of prostaglandin E2 to conscious mice. Pain. 1994. 57:217–223.
13. Yaksh TL. Behavioral and autonomic correlates of the tactile evoked allodynia produced by spinal glycine inhibition: effects of modulatory receptor systems and excitatory amino acid antagonists. Pain. 1989. 37:111–123.
14. Campbell JN, Raja SN, Meyer RA, Mackinnon SE. Myelinated afferents signal the hyperalgesia associated with nerve injury. Pain. 1988. 32:89–94.
15. Shir Y, Seltzer Z. A-fibers mediate mechanical hyperesthesia and allodynia and C-fibers mediate thermal hyperalgesia in a new model of causalgiform pain disorders in rats. Neurosci Lett. 1990. 115:62–67.
16. Yamamoto T, Yaksh TL. Effects of intrathecal strychnine and bicuculline on nerve compression-induced thermal hyperalgesia and selective antagonism by MK-801. Pain. 1993. 54:79–84.
17. Cheng W, Yin Q, Cheng MY, Chen HS, Wang S, Feng T, Zeng YM, Liu GJ. Intracerebroventricular or intrathecal injection of glycine produces analgesia in thermal nociception and chemical nociception via glycine receptors. Eur J Pharmacol. 2009. 614:44–49.
18. Hammond DL, Drower EJ. Effects of intrathecally administered THIP, baclofen and muscimol on nociceptive threshold. Eur J Pharmacol. 1984. 103:121–125.
19. Wilson PR, Yaksh TL. Baclofen is antinociceptive in the spinal intrathecal space of animals. Eur J Pharmacol. 1978. 51:323–330.
20. Aanonsen LM, Wilcox GL. Muscimol, gamma-aminobutyric acidA receptors and excitatory amino acids in the mouse spinal cord. J Pharmacol Exp Ther. 1989. 248:1034–1038.
21. Hao JX, Xu IS, Xu XJ, Wiesenfeld-Hallin Z. Effects of intrathecal morphine, clonidine and baclofen on allodynia after partial sciatic nerve injury in the rat. Acta Anaesthesiol Scand. 1999. 43:1027–1034.
22. Balerio GN, Rubio MC. Baclofen analgesia: involvement of the GABAergic system. Pharmacol Res. 2002. 46:281–286.
23. Chung KM, Kim YH, Song DK, Huh SO, Suh HW. Differential modulation by baclofen on antinociception induced by morphine and beta-endorphin administered intracerebroventricularly in the formalin test. Neuropeptides. 1999. 33:534–541.
24. Malan TP, Mata HP, Porreca F. Spinal GABA(A) and GABA(B) receptor pharmacology in a rat model of neuropathic pain. Anesthesiology. 2002. 96:1161–1167.