Abstract
Early diabetic nephropathy is characterized by glomerular hyperpermeability as a result of impaired glomerular filtration structure caused by hyperglycemia, glycated proteins or irreversible advanced glycosylation endproducts (AGE). To investigate the effect of ginseng total saponin (GTS) on the pathologic changes of podocyte ZO (zonula occludens)-1 protein and podocyte permeability induced by diabetic conditions, we cultured mouse podocytes under: 1) normal glucose (5 mM, = control); 2) high glucose (HG, 30 mM); 3) AGE-added; or 4) HG plus AGE-added conditions and treated with GTS. HG and AGE increased the dextran filtration of monolayered podocytes at early stage (2-8 hr) in permeability assay. In confocal imaging, ZO-1 colocalized with actin filaments and β-catenin at cell contact areas, forming intercellular filtration gaps. However, these diabetic conditions suppressed ZO-1 immunostainings and disrupted the linearity of ZO-1. In Western blotting, diabetic conditions also decreased cellular ZO-1 protein levels at 6 hr and 24 hr. GTS improved such quantitative and qualitative changes. These findings imply that HG and AGE have an influence on the redistribution and amount of ZO-1 protein of podocytes thereby causing hyperpermeability at early stage, which can be reversed by GTS.
Diabetic nephropathy is the leading cause of end-stage renal disease (ESRD) requiring dialysis or renal transplantation, however, the predisposing factors and pathogenic mechanisms of diabetic nephropathy have remained unclear (1, 2). Early clinical manifestations of diabetic nephropathy result from hyperfiltration and microalbuminuria, and progress to overt proteinuria and progressive renal injury. Simultaneous morphological changes in glomeruli include glomerular hypertrophy, increase in mesangial matrix, thickening of glomerular basement membrane (GBM), and effacement, denudation, and loss of podocytes (1, 2). Accompanying biochemical alterations with pathological changes lead to increase in glomerular permeability as a result of impaired glomerular filtration structures. These changes are caused by hyperglycemia, secondary glycated proteins or irreversible advanced glycosylation endproducts (AGE) (3, 4).
As the glomerular capillary wall functions as an efficient and selective barrier that allows a high flow rate of filtration for plasma water and small solutes, glomerular capillary wall should have strong but selectively permeable cell-to-cell junction. Therefore, the glomerular slit diaphragm (SD), a main barrier component in glomerular filtration, serves as a selective barrier and is connected to intracellular adapter proteins including ZO (zonula occludens)-1 (5-7). ZO-1 is a peripheral membrane protein associated with the cytoplasmic surface of the tight junction or ZO in all epithelial and endothelial cell types studied (8). It is also expressed on the cytoplasmic surface of podocyte foot processes at the point of insertion of the SD and linked to the actin cytoskeleton on the other side (5-7). Therefore, ZO-1 protein as a component of the SD plays a pivotal role in maintaining the glomerular permeability by connecting SD structure and actin cytoskeleton.
The root of Panax ginseng has been widely used for cancer, diabetes, and cardiovascular diseases for thousands of years in oriental countries including Korea (9, 10). Several investigations strongly support the evidence that ginseng root possesses antidiabetic properties, such as inhibition of intestinal glucose absorption, increase in energy expenditure, improving sensitivity to insulin and stimulation of sugar metabolism, etc. (11-13). Moreover, ginseng root and its derivatives have been beneficial in both type 2 diabetic patients and healthy individuals (14-16) as well as type 1 and 2 animal diabetic models (10-13, 17, 18).
Ginseng has also been reported to be effective in prevention and treatment of diabetic nephropathy of type 1 and type 2 diabetic animal models as followings. In type 1 insulin-dependent diabetic nephropathy animal models induced by streptozotocin (STZ), sun ginseng (19), heat-processed American ginseng (20), and 20(S)-ginsenoside Rg3 (21) ameliorated elevated serum glucose and renal damage. In type 2 insulin-independent diabetic nephropathy animal models, 20(S)-ginsenoside Rg3 also decreased the elevated blood glucose and proteinuria and augmented creatinine clearance in type 2 diabetic Otsuka Long-Evans Tokushima Fatty (OLETF) (22). Although the renal protective effect of ginseng components in diabetic models has been reported, there were very few reports which have attempted to elucidate the changes of glomerular filtration structures. In this study, we investigated the effect of ginseng saponin on the pathologic changes of podocyte ZO-1 protein and podocyte permeability induced by diabetic conditions.
Conditionally immortalized mouse podocytes were kindly provided by Dr. Peter Mundel (University of Harvard, Boston, MA, USA) and were cultured and differentiated as described previously (23). Briefly, to stimulate podocytes proliferation, cells were cultivated at 33℃ (permissive conditions) in a culture medium supplemented with 10 U/mL mouse recombinant γ-interferon (Roche, Mannheim, Germany) to induce expression of temperature-sensitive large T antigen. To induce differentiation, podocytes were maintained at 37℃ without γ-interferon (non-permissive conditions) for at least 2 weeks.
Cells were serum-deprived to reduce background 24 hr before each experiment, then exposed to glucose and/or AGE. Mouse podocytes were incubated in culture media containing either 5 mM (normal glucose, NG) or 30 mM glucose (HG) without insulin. AGE was produced by the technique previously described by Ha et al. (24). To imitate the long-term diabetic condition, AGE was added (5 µg/mL) and controls were established using unmodified bovine serum albumin (BSA, 5 µg/mL). To exclude the effect of additionally produced glycated proteins in culture conditions, no longer than 48 hr of incubation was used. Fetal bovine serum was reduced to 0.5% on the last media change to reduce background before protein and RNA extraction. For identification purposes, AGE or BSA was denoted as 'A' or 'B', and glucose at 5 or 30 mM by '5' or '30', respectively. Their meanings of each condition have been described before (24).
For ginseng treatment podocytes were incubated with ginseng total saponin (GTS) at the concentrations of 0, 0.2, 1, 5, 25 µg/mL for 6, 24, 48 hr. GTS was kindly provided by Korea Ginseng Corporation.
Podocytes were seeded and grown confluently in monolayer pattern on the surface of cellulose semi-permeable membranes (Millicell-HA, Millipore Corp., Bedford, MA, USA) having 0.45 µm of pore size. After differentiation and complete washings of previously described media, fresh maintenance media without AGE and BSA were replaced into each aspect. Hydrostatic pressure was applied continuously from (lower) to apical to basolateral aspect. Then, 1 mg/mL of FITC-tagged anionic dextran (Molecular Probes, Invitrogen, Eugene, OR, USA) were put into the apical media and the filtered amounts of dextran at each incubation time were determined by spectrophotometer at 492 nm.
Podocytes that were grown on type I collagen-coated glass cover slips incubated for 24 hr were fixed in 4% paraformaldehyde, permeabilized in phosphate buffer solution, blocked with 10% normal goat serum, and labeled with monoclonal rabbit anti-rat ZO-1 antibody (Invitrogen, Eugene, OR, USA) or TRITC-phalloidin (Sigma Chemical Co., St. Louis, MO, USA) for F-actin staining. Primary antibody-bound specimens were incubated with 1:500 (v/v) Alexa 594 for red (Molecular Probes, Invitrogen)-conjugated respective secondary antibodies at RT for 1 hr. Coverslips were mounted in aqueous mountant and viewed with a Fluorescence microscope (BX51; OLYMPUS, Tokyo, Japan).
The confluently grown cell layers incubated with additives during 48-hr for ZO-1 and various durations for AGE were extracted and protein concentrations were determined as previously described (24). For the Western blotting of ZO-1, 30 µg of boiled extracts was applied on 8% SDS-PAGE gels and transferred to polyvinylidene fluoride membranes (Bio-Rad Laboratories, Hercules, CA, USA). Then, the membranes were air-dried and blocked in 3% fat-free milk before incubation with anti-ZO-1 antibody. After incubation with horseradish peroxidase-conjugated secondary antibodies (Santa Cruz Biotechnology Inc., Santa Cruz, CA, USA), bands were detected by using the ECL chemiluminescence system (Amersham Biotech Ltd., Bucks, UK). Data on the densitometric analysis of ZO-1/β-tubulin ratio are expressed as mean ± SD.
At early stage (2-6 hr) of permeability assay, high glucose (B30) increased permeability, then, became to be a control level (B5) in Fig. 1A. On the other hand, AGE and high glucose (A30) increased permeability at somewhat late stage (4-8 hr), then, became to be a control level (B5) after 16 hr (Fig. 1B). Both hyperpermeable changes induced by B30 and A30 g were improved by GTS (1 µg/mL). These results indicated that GTS improved podocyte hyperpermeability induced by either high glucose and/or AGE during hyperpermeable conditions.
ZO-1 colocalized with actin filaments at cell contact areas (Fig. 2A). GTS affected ZO-1 distribution to be concentrated at over 5 µg/mL, therefore, we determined GTS concentration at 1 µg/mL (Fig. 2A). ZO-1 also colocalized with β-catenin at cell contact areas and diabetic conditions including B30, A5, and especially A30 suppressed and disrupted the immunostaining and linearity of ZO-1 protein (arrows, Fig. 2B) with β-catenin. GTS (1 µg/mL) improved the decreased and separated ZO-1 immunostaining to be as those in B5 (Fig. 2C). These findings imply that glucose and AGE might have an influence on the redistribution and amount of ZO-1 protein of podocytes thereby causing hyperpermeability, which could be reversed by GTS.
Density values for ZO-1 protein of representative immunoblots from each group revealed decreased cellular ZO-1 protein levels in B30 and A30 conditions at 6 and 24 hr incubations (P < 0.05) but not at 48 hr incubations compared with B5 (P > 0.05, Fig. 3A). High glucose (B30) suppressed ZO-1 protein amount by 16.0% at 6 hr, which was reversed by GTS and by 27.5% at 24 hr incubation (Fig. 3A). Focusing on AGE and high glucose (A30) according to the incubation times, A30 condition decreased ZO-1 protein amount by 21.7% at 6 hr and by 21.8% at 24 hr incubation (P < 0.05). GTS (1 µg/mL) improved the decreased ZO-1 protein at both incubation times, especially at 6 hr significantly (Fig. 3B).
There have been several reports of Panax ginseng root (American and Asia ginseng) and its derivatives improving diabetic conditions in both type 2 human diabetes as well as type 1 and 2 animal diabetic models (11-18). Ginseng has also been reported to be effective in diabetic nephropathy of type 1 and type 2 diabetic animal models (19-22).
There were various mechanisms explained as a mode of effective action by Panax ginseng root and its derivatives on diabetic nephropathy. In streptozotocin-induced model, Kang et al. (19, 21) found that 20(S)-ginsenoside Rg3 and sun ginseng ameliorated elevated serum glucose, glycosylated protein, and thiobarbituric acid-reactive substance levels and, furthermore, sun ginseng decreased the levels of 3-nitrotyrosine, carboxymethyllysine, and receptors for AGE, which indicated that ginseng components could prevent diabetic renal damage by attenuating the oxidative stress. Similarly, ginseng non-saponin (GNS) treatment increased Cu/Zn-SOD activity in this model (25). In type 2 insulin-independent diabetic nephropathy animal models (OLETF), 20(S)-ginsenoside Rg3 also decreased the elevated blood glucose and proteinuria via inhibiting oxidative stress and advanced glycation endproduct formation (22).
In cultured mesangial cells, one of the major pathologic glomerular cells in diabetic nephropathy, the total saponin and ginsenoside-Rd fractions suppressed the proliferation of mesangial cells (26) and ginsenoside Rb1 prevented the high glucose-induced increase of fibronectin expression via the inhibition of MAPK-Akt signaling cascade (27). However, there were no reports in this field using cultured podocytes, a major glomerular filtration component.
In type 1 insulin-dependent diabetic nephropathy animal models induced by streptozotocin (STZ), Kim and Chung (25) reported that GTS and GNS treatments slightly decreased blood pressure and ameliorated renal hypertrophy with the down-regulations of TGF-β1 and fibronectin expressions and without affecting plasma glucose levels. Focusing on glomerular filtration structures, the main determinant of the development of hyperfiltration and proteinuria, Zhang et al. (28) found that ginsenoside Rgl improved the pathologic diabetic changes of glomerular filtration, such as, GBM thickness and podocytopenia with the reduction of urine protein and serum creatine. As ginsenoside Rgl also improved the overexpressed levels of serum MCP-1 and TNF-α, which correlated with the improved clinical and pathologic indices. In summary, the components of Panax ginseng root and its derivatives may play preventive or therapeutic roles in diabetic nephropathy via the modulation of oxidative stress, signaling pathways, cell cycling, and/or cytokines. However, we could not find any reports on the effects of ginseng on the podocyte molecules.
We previously reported that the increased albumin permeability through podocytes monolayer by AGE and high glucose could be induced by a disruption of cell-to-cell size-selective SD barrier including ZO-1 (29), which supported by Rincon-Choles et al. (30). In this study, we found the positive effects of ginseng saponin on the pathologic changes of podocyte ZO-1 protein and podocyte hyperpermeability induced by diabetic conditions. Further studies on the connecting mechanisms, such as signaling and oxidative stress, and the changes of other podocyte proteins would be needed.
In conclusion, the results of our in vitro study document that both high glucose- and AGE-added condition induce the distributional change and suppress the production of ZO-1 protein, thus causing the phenotypical changes and hyperpermeability of podocytes, which can be improved by GTS. Therefore, GTS will be helpful to prevent the early hyperpermeable condition in diabetic nephropathy.
Figures and Tables
Fig. 1
Effects of ginseng total saponin (GTS) on the increased monolayered podocyte permeability by diabetic conditions. High glucose (B30) increases permeability at early stage (A) and AGE and high glucose (A30) also increases permeability at mid stage (B).
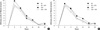
Fig. 2
Distributional changes of ZO-1 by diabetic conditions and ginseng total saponin (GTS). ZO-1 colocalizes with actin filaments (A) and β-catenin (B) at cell contact areas. Diabetic conditions including B30, A5, and A30 suppresses and disrupts the immunostainings and linearity of ZO-1 protein (arrows, B and C), which improved by GTS (1 µg/mL) (C). Magnification, × 1,000; Scale bar = 20 µm.
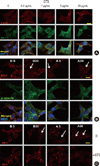
Fig. 3
Effects of diabetic conditions and ginseng total saponin (GTS) on the ZO-1 protein assayed by Western blotting. The major band for ZO-1 protein at 205 kDa decreased in B30 and A30 conditions at 6 and 24 hr incubations, which improved by GTS (1 µg/mL) (A, B). Data on the densitometric analysis of ZO-1/β-tubulin (not shown) ratio are expressed as mean ± SD (n = 4). Control (100%); the value of B5. *P < 0.01 versus control.
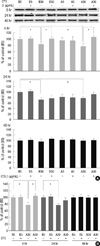
ACKNOWLEDGMENTS
The author thanks E-M Ahn for their technical assistances and Dr. Peter Mundel for mouse podocytes.
AUTHOR SUMMARY
Ginseng Total Saponin Improves Podocyte Hyperpermeability Induced by High Glucose and Advanced Glycosylation Endproducts
Tae-Sun Ha, Ji-Young Choi, Hye-Young Park and Jin-Seok Lee
Early diabetic nephropathy is characterized by glomerular hyperpermeability as a result of podocyte injury. This study is to evaluate effects of ginseng total saponin (GTS) on the pathologic changes of podocyte ZO (zonula occludens)-1, a key molecule in podocyte permeability, induced by diabetic conditions. Diabetic conditions suppressed ZO-1 protein and mRNA expression and disrupted the linearity of ZO-1. GTS improved such quantitative and qualitative changes. These findings imply that diabetic conditions induce glomerular hyperpermeability by modulating podocytes ZO-1 and GTS can be a candidate for early renal protective agent in diabetes.
References
1. Parving HH, Mauer M, Ritz E. Brenner BM, editor. Diabetic nephropathy. The kidney. 2008. Philadelphia, PA: WB Saunders;1265–1298.
2. Kanwar YS, Wada J, Sun L, Xie P, Wallner EI, Chen S, Chugh S, Danesh FR. Diabetic nephropathy: mechanisms of renal disease progression. Exp Biol Med (Maywood). 2008. 233:4–11.
3. Sharma K, Ziyadeh FN. Biochemical events and cytokine interactions linking glucose metabolism to the development of diabetic nephropathy. Semin Nephrol. 1997. 17:80–92.
4. Vlassara H. Protein glycation in the kidney: role in diabetes and aging. Kidney Int. 1996. 49:1795–1804.
5. Mundel P, Kriz W. Structure and function of podocytes: an update. Anat Embryol (Berl). 1995. 192:385–397.
6. Reiser J, Kriz W, Kretzler M, Mundel P. The glomerular slit diaphragm is a modified adherens junction. J Am Soc Nephrol. 2000. 11:1–8.
7. Mundel P, Shankland SJ. Podocyte biology and response to injury. J Am Soc Nephrol. 2002. 13:3005–3015.
8. Stevenson BR, Siliciano JD, Mooseker MS, Goodenough DA. Identification of ZO-1: a high molecular weight polypeptide associated with the tight junction (zonula occludens) in a variety of epithelia. J Cell Biol. 1986. 103:755–766.
9. Chang YS, Seo EK, Gyllenhaal C, Block KI. Panax ginseng: a role in cancer therapy? Integr Cancer Ther. 2003. 2:13–33.
10. Attele AS, Zhou YP, Xie JT, Wu JA, Zhang L, Dey L, Pugh W, Rue PA, Polonsky KS, Yuan CS. Antidiabetic effects of Panax ginseng berry extract and the identification of an effective component. Diabetes. 2002. 51:1851–1858.
11. Chung SH, Choi CG, Park SH. Comparisons between white ginseng radix and rootlet for antidiabetic activity and mechanism in KKAy mice. Arch Pharm Res. 2001. 24:214–218.
12. Banz WJ, Iqbal MJ, Bollaert M, Chickris N, James B, Higginbotham DA, Peterson R, Murphy L. Ginseng modifies the diabetic phenotype and genes associated with diabetes in the male ZDF rat. Phytomedicine. 2007. 14:681–689.
13. Yuan HD, Shin EJ, Chung SH. Anti-diabetic effect and mechanism of Korean red ginseng in C57BL/KsJ db/db mice. J Ginseng Res. 2008. 32:187–193.
14. Sotaniemi EA, Haapakoshi E, Rautio A. Ginseng therapy in non-insulin-dependent diabetic patients. Diabetes Care. 1995. 18:1373–1375.
15. Vogler BK, Pittler MH, Ernst E. The efficacy of ginseng. A systematic review of randomised clinical trials. Eur J Clin Pharmacol. 1999. 55:567–575.
16. Vuksan V, Sievenpiper JL, Wong J, Xu Z, Beljan-Zdravkovic U, Arnason JT, Assinewe V, Stavro MP, Jenkins AL, Leiter LA, Francis T. American ginseng (Panax quinquefolius L) attenuates postprandial glycemia in a time-dependent but not dose-dependent manner in healthy individuals. Am J Clin Nutr. 2001. 73:753–758.
17. Liu TP, Liu IM, Cheng JT. Improvement of insulin resistance by panax ginseng in fructose-rich chow-fed rats. Horm Metab Res. 2005. 37:146–151.
18. Luo JZ, Luo L. Ginseng on hyperglycemia: effects and mechanisms. Evid Based Complement Alternat Med. 2009. 6:423–427.
19. Kang KS, Kim HY, Yamabe N, Nagai R, Yokozawa T. Protective effect of sun ginseng against diabetic renal damage. Biol Pharm Bull. 2006. 29:1678–1684.
20. Kim HY, Kang KS, Yamabe N, Nagai R, Yokozawa T. Protective effect of heat-processed American ginseng against diabetic renal damage in rats. J Agric Food Chem. 2007. 55:8491–8497.
21. Kang KS, Yamabe N, Kim HY, Park JH, Yokozawa T. Therapeutic potential of 20(S)-ginsenoside Rg(3) against streptozotocin-induced diabetic renal damage in rats. Eur J Pharmacol. 2008. 591:266–272.
22. Kang KS, Yamabe N, Kim HY, Park JH, Yokozawa T. Effects of heat-processed ginseng and its active component ginsenoside 20(S)-Rg3 on the progression of renal damage and dysfunction in type 2 diabetic Otsuka Long-Evans Tokushima Fatty rats. Biol Pharm Bull. 2010. 33:1077–1081.
23. Mundel P, Reiser J, Zúñiga Mejía Borja A, Pavenstädt H, Davidson GR, Kriz W, Zeller R. Rearrangements of the cytoskeleton and cell contacts induce process formation during differentiation of conditionally immortalized mouse podocyte cell lines. Exp Cell Res. 1997. 236:248–258.
24. Ha TS, Song CJ, Lee JH. Effects of advanced glycosylation endproducts on perlecan core protein of glomerular epithelium. Pediatr Nephrol. 2004. 19:1219–1224.
25. Kim YL, Chung SH. Renoprotective effects of Korean red ginseng. J Ginseng Res. 2004. 28:111–119.
26. Yokozawa T, Iwano M, Dohi K, Hattori M, Oura H. Inhibitory effects of ginseng on proliferation of cultured mouse mesangial cells. Nihon Jinzo Gakkai Shi. 1994. 36:13–18.
27. Park MJ, Bae CS, Lim SK, Kim DI, Lim JC, Kim JC, Han HJ, Moon JH, Kim KY, Yoon KC, Park SH. Effect of protopanaxadiol derivatives in high glucose-induced fibronectin expression in primary cultured rat mesangial cells: role of mitogen-activated protein kinases and Akt. Arch Pharm Res. 2010. 33:151–157.
28. Zhang LN, Xie XS, Zuo C, Fan JM. Effect of ginsenoside Rgl on the expression of TNF-alpha and MCP-1 in rats with diabetic nephropathy. Sichuan Da Xue Xue Bao Yi Xue Ban. 2009. 40:466–471.
29. Ha TS. High glucose and advanced glycosylation end products increased podocyte permeability via PI3K/Akt signaling. J Mol Med (Berl). 2010. 88:391–400.
30. Rincon-Choles H, Vasylyeva TL, Pergola PE, Bhandari B, Bhandari K, Zhang JH, Wang W, Gorin Y, Barnes JL, Abboud HE. ZO-1 expression and phosphorylation in diabetic nephropathy. Diabetes. 2006. 55:894–900.