Abstract
Secondary lymphoid tissue chemokine (SLC), which is expressed in T cell zones of secondary lymphoid organs, including the spleen and lymph nodes, strongly recruits both T lymphocytes and mature dendritic cells. As appropriate interaction of tumor-specific T cells and mature dendritic cells, equipped with tumor antigens, is a prerequisite for effective T cell immunity against established tumors, we mobilized lymphocytes and dendritic cells to tumor sites by intratumoral injection of secondary lymphoid tissue chemokine-Fc (SLC-Fc) fusion protein using the B16F10 murine melanoma model. Activation of dendritic cells, another prerequisite for the effective activation of naïve tumor-specific T cells, was achieved by the addition of immunostimulatory cytosine-phosphorothioate-guanine oligodeoxynucleotide (CpG-ODN) into the tumor site. Intratumoral administration of SLC-Fc or CpG-ODN revealed antitumor effects against B16F10 murine melanoma grown in the subcutaneous space. Co-treatment of SLC-Fc and CpG-ODN displayed synergistic effects in reducing the tumor size. The synergistic antitumor effect in co-treatment group was correlated with the synergistic/additive increase in the infiltration of CD4+ T cells and CD11c+ dendritic cells in the tumor mass compared to the single treatment groups. These results suggest that the combined use of chemokines and adjuvant molecules may be a possible strategy in clinical tumor immunotherapy.
The effective induction of antitumor immune responses is crucial in tumor immunotherapy. The initiation of adaptive immunity against a tumor depends heavily on tumor-specific T cell interactions and activation with antigen presenting cells (APCs) appropriately loaded with tumor antigens on their MHC molecules (1). Dendritic cells (DCs) are the APCs for the initiation of an adaptive T cell immune response (2). Responding to appropriate activating signals, immature tissue DCs that take up tumor antigens turn into activated DCs and migrate into draining lymph nodes (LNs), activating tumor-specific T cells. Interaction of activated DCs and recirculating lymphocytes is facilitated within the T cell zones of LNs. Activated tumor-specific T cells in turn migrate from the LNs and move into the tumor site, attacking tumor cells (3).
Because most of the tumor-specific naïve T cells recirculate within the vascular system and the secondary lymphoid organ tumor antigens are confined in peripheral tissues, and because tissue-resident DCs are immature and thus tolerogenic, it is difficult for tumor-specific T cells to be fully activated. To create an environment in which naïve T cells and mature DCs meet, we propose to use a chemoattractant that can attract both of these cell components into the tumor site.
Chemokines are a group of homologous, functionally divergent proteins that mediate leukocyte migration and activation (4). Chemokines also function in maintaining immune homeostasis and secondary lymphoid organ architecture (5). Secondary lymphoid tissue chemokine (SLC, CCL21), a cystein-cystein (C-C) chemokine expressed by high endothelial venules in the T cell zones of secondary lymphoid organs such as the spleen and LNs, strongly recruits both naïve and central memory T cells and mature DCs (6, 7). Receptors of SLC, C-C chemokine receptor 7 (CCR7) and C-X-C chemokine receptor 3 (CXCR3), are specific G protein-coupled seven-transmembrane domain chemokine receptors (4). CCR7 is expressed in naïve and central memory T cells and mature DCs. CXCR3 is expressed preferentially in Th1 cytokine-producing lymphocytes with memory phenotypes (8, 9).
CpG-ODN is a synthetic oligodeoxynucleotide (ODN) containing unmethylated cytosine-phosphorothioate-guanine (CpG) motifs. Tokunaga et al. (10) was the first to report that DNA extracted from Mycobacterium bovis activated natural killer cells to produce interferon and to induce antitumor activity. This research group also established that particular sequences with 5'-CpG-3' motif(s) are essential for these effects (10). Synthetic CpG-ODN was reported to have similar immunologic effects (11) by activating innate immunity through Toll-like receptor 9 (12). CpG-ODN has less toxicity than other adjuvants, such as Freund's adjuvant (13), and particular interest is focused on the induction and activation of Th1 cells and CD8+ cytotoxic T lymphocytes through the activation and maturation of DCs (14, 15).
Melanoma is the main cause of death due to skin malignancies. Although surgery can be curative for most patients with early thin melanoma, 5-yr survival rates of patients with high-risk, thick melanoma are as low as 25% despite recent advances in melanoma therapy (16). B16F10 melanoma cells, which originated from C57BL/6 (B6) mice, produce tumor masses in syngeneic B6 mice when injected subcutaneously. Several tumor-specific antigens characterized from melanoma cells are weakly immunogenic and do not raise an effective anti-melanoma immune response in syngeneic B6 mice.
To produce an effective antitumor immune response directly inside the tumor, we set up an experimental melanoma model by subcutaneously injecting B16F10 melanoma cells into B6 mice and applied SLC directly into the established tumor, which could recruit T lymphocytes and DCs (17). We used the SLC-Fc fusion protein to enhance in vivo molecular stability (18) and CpG-ODN as an adjuvant immune stimulator to produce the required DC activation during the initial interaction with tumor-specific T cells. Combined chemokine and adjuvant therapy was highly efficient in inhibiting tumor growth, and this effect was dependent on T cells and activated DCs accumulating in the tumor sites.
B16F10 mouse melanoma cells were obtained from the American Type Culture Collection (ATCC no. CRL-6475) and were cultured in RPMI 1640 media supplemented with 10% heat-inactivated fetal bovine serum and 1% antibiotics. NS0 cells were purchased from the European Collection of Cell Cultures (ECACC no. 85110503) and cultured in conditions identical to the B16F10 cells, except with 1% L-glutamine added to the media.
The oligonucleotide sequence containing the unmethylated CpG motifs (underlined; CpG-ODN: 5'-TCCATGACGTTCCTGACGTT-3') was synthesized at GenoTech (Daejeon, Korea).
Total RNA was isolated from the spleen using Trizol (Invitrogen, Carlsbad, CA, USA). cDNA was generated from 1 µg total RNA by Superscript II (Invitrogen). PCR of the murine SLC gene was performed using the following primers: 5'-CCG CTC GAG GCC ACC ATG GCT CAG A-3'/5'-GAA GAT CTT CTT CTT GAG GG-3'. Murine SLC and human IgG1-Fc genes were cloned into pcDNA3.1 (Invitrogen) vector. NS0 cells were stably transfected with pcDNA3.1-SLC-Fc using lipofectamine 2000 (Invitrogen) and selected with 0.5 mg/mL geneticin (G418). Stably transfected clones were tested for SLC-Fc expression by ELISA and chemotaxis assay. Selected clones were cultured in UltraCulture serum free media (BioWhittaker, Walkersville, MD, USA) supplemented with 1% L-glutamine and 1% antibiotics. SLC-Fc protein was purified using HiTrap Protein G column (GE Healthcare, Piscotaway, NJ, USA).
ELISA plates (Costar, Cambridge, MA, USA) were coated overnight with anti-SLC antibody (BD Pharmingen, San Diego, CA, USA) at 4℃. Wells were blocked with PBS containing 1% bovine serum albumin (Sigma Aldrich, St. Louis, MO, USA) for 1 hr at room temperature (RT) and incubated with NS0 transfectant culture supernatants overnight at 4℃. After washing, plates were incubated with biotinylated anti-human IgG1 antibody for 3 hr at RT followed by strepavidin-conjugated horseradish peroxidase for 1 hr at RT. Color was developed using o-phenylenediamine dihydrochloride (Sigma Aldrich), and absorbance was read at 495 nm with a Multiskan Ascent (Labsystems, Santa Clara, CA, USA).
Lymphocytes were prepared from peripheral LNs. They were gently ground between frosted glass slides, passed over nylon mesh (70 µm), and resuspended in RPMI 1640 media containing 10% fetal bovine serum. Assay was performed in 24-well plates with a 5-µm pore polycarbonate Transwell insert (Costar). NS0 transfectant culture supernatants or recombinant murine SLC (R&D Systems, Minneapolis, MN, USA) was added to the lower chamber at the indicated concentration in a volume of 600 µL and incubated at 37℃ for 30 min prior to the addition of cells. Then 1 × 106 cells in 100 µL medium were added to the top chamber and incubated for 4 hr. Cells migrating to the bottom chamber were stained with anti-CD4, anti-CD8, and anti-B220 antibodies (BD Pharmingen, San Diego, CA, USA); counted; and analyzed by FACSCalibur according to the previous reports. Chemotaxis index was calculated as the fold increase in lymphocytes migrating in response to the chemoattractant versus the media control.
Male C57BL/6 (B6, H-2b) mice were purchased from the Jackson Laboratory (Bar Harbor, ME, USA), bred, and maintained in pathogen-free conditions at the animal facility of Seoul National University College of Medicine. All animal experiments were performed with the approval of the Institutional Animal Care and Use Committee of Seoul National University. A total of 2 × 105 B16F10 tumor cells resuspended in 10 µL PBS were inoculated subcutaneously in the shaved left flank area. When tumor volume reached approximately 150 µL, intratumoral injection of 3 µg purified murine SLC-Fc and/or 10 µg CpG-ODN resuspended in 10 µL pyrogen-free water was administered twice a week for 1 or 2 weeks (19). Tumor size was monitored every 2 days, and tumor volumes were estimated as (width × length × height) × π/6. Tumor-infiltrating cells were analyzed at day 11 after tumor inoculation. Lymphocytes from the draining LNs were prepared by gentle grinding between frosted glass slides and passing over nylon mesh (70 µm). Cells from the spleen and tumor mass were obtained by chopping and treating with collagenase D (100 µg/ea) for 25 min at 37℃ and then passing it over nylon mesh (70 µm). Isolated cells were stained with murine antibodies against CD4, CD8, CD11c, CD86, I-Ab (Y3P), CD25, CD62L, and CD44 (BD Pharmingen) (20).
The chemotaxis index and migrated cell numbers were expressed as means ± SD. A two-tailed Student's t-test was used to compare measurements taken from the two samples. For in vivo experiments, differences in tumor volume were analyzed using a two-way ANOVA. All P values < 0.05 were deemed to indicate statistical significance. The SPSS software (ver. 11.0; SPSS Inc., Chicago, IL, USA) was used for all statistical analyses.
The bioactivity of SLC-Fc was tested by investigating the migration of T lymphocytes using a chemotaxis assay. Chemotactic activity of the lymphocytes was assessed by quantifying lymphocytes migrating across 5-µm pore membranes in a Transwell with chemotactic stimuli placed in the lower chamber. Responder cells were obtained from the peripheral LNs of B6 mice. After 4 hr incubation time, the number of migrated cells increased > 20 fold compared to the isotype control. The percentage of CD4+ and CD8+ T cells also increased compared to the original responder cells (Fig. 1A). The bioactivity of SLC-Fc fusion protein with recombinant SLC was also compared using chemotaxis assay. The chemotactic activity of SLC-Fc was comparable to that of recombinant SLC. The result was also dose dependent (Fig. 1B, C).
An in vivo melanoma model was established by subcutaneous injection of 2 × 105 B16F10 melanoma cells into 10- to 12-week-old B6 male mice. Tumor size was measured every 2 days. To evaluate the effect of SLC-Fc on the reduction of established tumors, we performed intratumoral injection of 3 µg SLC-Fc two or four times when the tumor volume reached approximately 150 µL. Compared to the vehicle-treated control, SLC-Fc treatment reduced the tumor size in a dose-dependent manner (Fig. 2A).
The effect of CpG-ODN was also evaluated by intratumoral injection when the tumor volume reached approximately 150 µL. Tumor growth was inhibited by a single treatment of CpG-ODN in a dose-dependent manner (Fig. 2B), and treatment with 10 µg CpG-ODN twice per week for 2 weeks greatly reduced the growth of the established tumor (Fig. 2C).
Synergistic antitumor effects of SLC-Fc and CpG-ODN were evaluated by-intratumoral co-treatment when the tumor volume reached approximately 150 µL. Tumor growth was inhibited most efficiently with SLC-Fc/CpG-ODN co-treatment compared to single treatment (Fig. 3).
Eleven days after the initiation of intratumoral injections of SLC-Fc and/or CpG-ODN, infiltrating cells inside the tumor were analyzed using a flow cytometer. The percentages of CD4+ and CD8+ T cells were increased in SLC-Fc-treated (CD4+ T: 14.7%, CD8+ T: 13.1%), CpG-ODN-treated (CD4+ T: 10%, CD8+ T: 15.6%), and SLC-Fc/CpG-ODN co-treated (CD4+ T: 15.2%, CD8+ T: 11.7%) groups compared to the vehicle-treated control group (CD4+ T: 0.76%, CD8+ T: 0.79%). The SLC-Fc-treated group (CD11c+: 7.7%, CD11c+CD86+: 16.2%) and the CpG-ODN-treated group (CD11c+: 13.5%, CD11c+CD86+: 36.3%) showed a greater increase in DC population and mature DCs compared to the vehicle-treated control group (CD11c+: 3.35%, CD11c+CD86+: 15.6%). Co-treatment of SLC-Fc and CpG-ODN further increased the tumor-infiltrating DC population (CD11c+: 18.2%, CD11c+CD86+: 49.2%) (Fig. 4A). Expression of MHC class II molecule I-Ab, detected by Y3P antibody, on DCs was also increased in SLC-Fc and/or CpG-ODN treated mice compared to the vehicle-treated control group (Fig. 4A). Total numbers of the tumor-infiltrating cells were increased in CpG-ODN- or SLC-Fc- treated mice compared to the vehicle-treated control group; co-treatment of SLC-Fc and CpG-ODN further increased these cells compared to the CpG-ODN- or SLC-Fc- treated groups (Fig. 4B). When the phenotypes of the subpopulations of tumor-infiltrating cells were further analyzed, the synergistic effect of co-treated SLC-Fc and CpG-ODN in in vivo tumor growth was explained by the synergistic/additive increase of CD4+ T cells and CD11c+ DCs in co-treatment group compared to the single treatment groups (Fig. 4C).
Most tumor vaccine strategies rely on the availability of well-characterized tumor antigens or their antigenic peptides. Because few tumor-specific antigens have been characterized, and antigen-specific treatment of tumors is frequently ineffective, alternative or supplemental strategies are required. We focused on inducing a tumor-specific adaptive immune response inside the tumor. For an effective antitumor immune response, we assumed two prerequisites: a chemokine that can recruit lymphocytes and APCs, and an adjuvant that can activate APCs. We chose SLC, which recruits lymphocytes and DCs into the secondary lymphoid organ through the CCR7 receptor, and CpG-ODN, which mimics prokaryotic stimulation. In addition, SLC induces angiostatic activity through interaction with another receptor, CXCR3, which potentiates an antitumor response.
Intratumoral injection of SLC-Fc inhibited tumor growth and induced T cell and DC accumulation in the tumor mass. Single treatment of CpG-ODN reduced the tumor in a dose-dependent manner, and repeated intratumoral injection of CpG-ODN resulted in strong inhibition of the growth of established tumors. CpG-ODN does not directly activate T lymphocytes (data not shown) but exerts its effect via induction of migration and maturation of DCs inside the tumor mass. Thus, repeated CpG-ODN treatment leads to a continuous priming of T cells by activated APCs. One caveat for this regimen is that repeated administration of CpG-ODN was recently reported to alter the structure and function of secondary lymphoid organs (21). CpG-ODN treatment over 7 days strongly reduced primary humoral immune responses and immunoglobulin class switching. By day 20 of CpG-ODN administration, mice developed multifocal hepatic necrosis and hemorrhagic ascites (21). Thus, repeated application of CpG-ODN has potential problems as a long-term treatment regimen. In this context, synergistic effects of SLC-Fc and CpG-ODN shown in this report could reduce the dosage of CpG-ODN, reducing the potential harmful side effects of this reagent.
Chemokines have recently been introduced in tumor immunotherapy as a means of recruiting effector immune cells (22). Among these, CXCL12, CXCL14, CCL16, CCL20, and CCL21 (SLC) have provided for tumor regression in various treatment modalities, including direct injection and induced expression in tumor cells (23-26). In addition to the recruitment of inflammatory cells, chemokines are involved in lymphoid organogenesis. The presence of intratumoral lymphoid structures is correlated with the long-term survival of cancer patients (27); a recent report showing that lymphoid-like structures are induced surrounding tumors expressing CCL21 (28) is intriguing. As recruitment of inflammatory cells is not sufficient for full activation of tumor-specific T cells, a combination with an activating signal would represent an improved strategy. In this context, forced expression of CCL21 and LIGHT had a synergistic antitumor effect (29). Induced expression of LIGHT in the tumor can induce substantial infiltration of naïve T cells correlating with an upregulation of both chemokine (notably CCL21) production and expression of adhesion molecules (30). Thus, the effect of LIGHT expression is not immediate but requires the upregulation of downstream effector molecules. Thus, co-administration of CCL21 and CpG-ODN in this study would act immediately by recruiting inflammatory cells and activating them in the tumor sites.
Antitumor effects of SLC-Fc and CpG-ODN co-treatment continued until 27 days of tumor inoculation. Very small tumors eventually slowly grew out after this time. Thus, the treatment schedule for these reagents should be optimized to ensure more effective long-term tumor growth inhibition. Moreover, the use of additional treatment modalities that use other immunomodulating strategies, such as blocking tumor-induced immune tolerance mechanisms, could result in improved tumor immunotherapies.
Figures and Tables
Fig. 1
SLC-Fc is chemotactic for CD4+ and CD8+ T cells in vitro. A total of 1 × 106 lymphocytes from peripheral LNs of B6 male mice were incubated in the upper chamber of the Transwell. After 4 hr incubation, migrated cells in the lower chamber were stained with anti-CD4, anti-CD8, and anti-B220 antibodies and analyzed by FACSCalibur. (A) Flow cytometric analysis of migrated cells in the lower chamber with culture media, 300 ng SLC-Fc, or 200 ng recombinant SLC (rSLC). Data shown are representative of two independent experiments. (B, C) Chemotaxis assay performed with 0.2 µg recombinant SLC or 3, 0.3, or 0.03 µg purified SLC-Fc in the lower chamber. The chemotaxis index was calculated as described in Materials & Methods. Data represent means ± SD, based on three independent determinations using samples in triplicate. *P ≤ 0.05; †P ≤ 0.005.
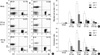
Fig. 2
Intratumoral injection of CpG-ODN induces the rejection of established tumors. Ten- to 12-week-old B6 male mice were inoculated subcutaneously with 2 × 105 B16F10 melanoma cells in the shaved left flank. Tumor size was recorded every 2 days. Intratumoral injection was performed when the tumor volume reached approximately 150 µL. (A) SLC-Fc treatment (3 µg), two or four times. (B) CpG-ODN treatment (1, 5, or 10 µg) once. (C) CpG-ODN treatment (1, 5, or 10 µg), two times. Data represent means ± SD of two independent determinations with n = 6 mice/group. *P < 0.05; †P < 0.01; ‡P < 0.001.
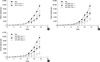
Fig. 3
Synergistic antitumor effect of SLC-Fc and CpG-ODN. Ten- to 12-week-old B6 male mice were inoculated subcutaneously with 2 × 105 B16F10 melanoma cells in the shaved left flank. Tumor size was recorded every 2 days. Intratumoral injection was performed when the tumor volume reached approximately 150 µL. Data represent means ± SD of two independent determinations with n = 6 mice/group.
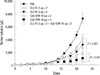
Fig. 4
Recruitment of T cells and DCs inside the tumor. B16F10 melanoma cells (2 × 105) were inoculated in the shaved left flank of B6 mice. When the tumor volume reached approximately 150 µL, mice were received with PBS, 10 µg CpG-ODN (twice), 3 µg SLC-Fc (twice), or 10 µg CpG-ODN plus 3 µg SLC-Fc (twice). Eleven days after intratumoral administration of CpG-ODN and/or SLC-Fc, tumors were removed. Infiltrating cells in the tumor mass were obtained by treatment with collagenase D (100 µg/ea) for 25 min at 37℃ and stained with anti-CD4, anti-CD8, anti-CD11c, anti-CD86, and anti-I-Ab (Y3P) antibodies. (A) Populations of the infiltrating cells in the tumor mass were analyzed by flow cytometry. (B) Total numbers of the infiltrating cells in the tumor mass were counted. (C) Each population of the infiltrating cells in the tumor mass was calculated from data based on flow cytometry analysis. Data represent means±SD of two independent determinations with n = 4 mice/group.
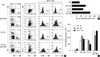
AUTHOR SUMMARY
Intratumoral Administration of Secondary Lymphoid Chemokine and Unmethylated Cytosine-phosphorothioate-guanine Oligodeoxynucleotide Synergistically Inhibits Tumor Growth in Vivo
So Mi Oh, Keunhee Oh and Dong-Sup Lee
Appropriate interaction of tumor-specific T cells and mature dendritic cells equipped with tumor antigens is a prerequisite for effective T cell immunity against tumors. We mobilized lymphocytes and dendritic cells to tumor sites by intratumoral injection of SLC-Fc fusion protein using the B16F10 murine melanoma model. Such treatment showed antitumor effects against the melanoma grown in the subcutaneous space. Co-treatment of SLC-Fc and CpG-ODN displayed synergistic antitumor effects, and the effects were correlated with the infiltration of T cells and dendritic cells. These results suggest that the combined use of chemokines and adjuvant molecules may be a possible strategy in clinical tumor immunotherapy.
References
1. Slingluff CL Jr, Chianese-Bullock KA, Bullock TN, Grosh WW, Mullins DW, Nichols L, Olson W, Petroni G, Smolkin M, Engelhard VH. Immunity to melanoma antigens: from self-tolerance to immunotherapy. Adv Immunol. 2006. 90:243–295.
2. Steinman RM, Hemmi H. Dendritic cells: translating innate to adaptive immunity. Curr Top Microbiol Immunol. 2006. 311:17–58.
3. Balkwill F. Cancer and the chemokine network. Nat Rev Cancer. 2004. 4:540–550.
4. Rot A, von Andrian UH. Chemokines in innate and adaptive host defense: basic chemokinese grammar for immune cells. Annu Rev Immunol. 2004. 22:891–928.
5. Randall TD, Carragher DM, Rangel-Moreno J. Development of secondary lymphoid organs. Annu Rev Immunol. 2008. 26:627–650.
6. Cyster JG. Chemokines and the homing of dendritic cells to the T cell areas of lymphoid organs. J Exp Med. 1999. 189:447–450.
7. Chan VW, Kothakota S, Rohan MC, Panganiban-Lustan L, Gardner JP, Wachowicz MS, Winter JA, Williams LT. Secondary lymphoid-tissue chemokine (SLC) is chemotactic for mature dendritic cells. Blood. 1999. 93:3610–3616.
8. Yoshida R, Nagira M, Kitaura M, Imagawa N, Imai T, Yoshie O. Secondary lymphoid-tissue chemokine is a functional ligand for the CC chemokine receptor CCR7. J Biol Chem. 1998. 273:7118–7122.
9. Jenh CH, Cox MA, Kaminski H, Zhang M, Byrnes H, Fine J, Lundell D, Chou CC, Narula SK, Zavodny PJ. Cutting edge: species specificity of the CC chemokine 6Ckine signaling through the CXC chemokine receptor CXCR3: human 6Ckine is not a ligand for the human or mouse CXCR3 receptors. J Immunol. 1999. 162:3765–3769.
10. Tokunaga T, Yamamoto H, Shimada S, Abe H, Fukuda T, Fujisawa Y, Furutani Y, Yano O, Kataoka T, Sudo T. Antitumor activity of deoxyribonucleic acid fraction from Mycobacterium bovis BCG. I. Isolation, physicochemical characterization, and antitumor activity. J Natl Cancer Inst. 1984. 72:955–962.
11. Kleeberg UR, Engel E, Israels P, Bröcker EB, Tilgen W, Kennes C, Gérard B, Lejeune F, Glabbeke MV, Lentz MA. Palliative therapy of melanoma patients with fotemustine. Inverse relationship between tumor load and treatment effectiveness. A multicentre phase II trial of the EORTC-Melanoma Cooperative Group (MCG). Melanoma Res. 1995. 5:195–200.
12. Hemmi H, Takeuchi O, Kawai T, Kaisho T, Sato S, Sanjo H, Matsumoto M, Hoshino K, Wagner H, Takeda K, Akira S. A toll-like receptor recognizes bacterial DNA. Nature. 2000. 408:740–745.
13. Jones TR, Obaldia N 3rd, Gramzinski RA, Charoenvit Y, Kolodny N, Kitov S, Davis HL, Krieg AM, Hoffman SL. Synthetic oligodeoxynucleotides containing CpG motifs enhance immunogenicity of a peptide malaria vaccine in Aotus monkeys. Vaccine. 1999. 17:3065–3071.
14. Vabulas RM, Pircher H, Lipford GB, Häcker H, Wagner H. CpG-DNA activates in vivo T cell epitope presenting dendritic cells to trigger protective antiviral cytotoxic T cell responses. J Immunol. 2000. 164:2372–2378.
15. Jakob T, Walker PS, Krieg AM, Udey MC, Vogel JC. Activation of cutaneous dendritic cells by CpG-containing oligodeoxynucleotides: a role for dendritic cells in the augmentation of Th1 responses by immunostimulatory DNA. J Immunol. 1998. 161:3042–3049.
16. Gray RJ, Pockaj BA, Kirkwood JM. An update on adjuvant interferon for melanoma. Cancer Control. 2002. 9:16–21.
17. Lo JC, Chin RK, Lee Y, Kang HS, Wang Y, Weinstock JV, Banks T, Ware CF, Franzoso G, Fu YX. Differential regulation of CCL21 in lymphoid/non-lymphoid tissues for effectively attracting T cells to peripheral tissues. J Clin Invest. 2003. 112:1495–1505.
18. Krautwald S, Ziegler E, Förster R, Ohl L, Amann K, Kunzendorf U. Ectopic expression of CCL19 impairs alloimmune response in mice. Immunology. 2004. 112:301–309.
19. Yang SH, Oh TK, Kim ST. Increased anti-tumor effect by a combination of HSV thymidine kinase suicide gene therapy and interferon-gamma/GM-CSF cytokine gene therapy in CT26 tumor model. J Korean Med Sci. 2005. 20:932–937.
20. Park JN, Suh C, Yang J, Park JS, Park KU, Min YJ, Kim HJ, Kim YH, Kim SH. Active immunization using dendritic cells mixed with tumor cells inhibits the growth of lymphomas. J Korean Med Sci. 2003. 18:372–380.
21. Heikenwalder M, Polymenidou M, Junt T, Sigurdson C, Wagner H, Akira S, Zinkernagel R, Aguzzi A. Lymphoid follicle destruction and immunosuppression after repeated CpG oligodeoxynucleotide administration. Nat Med. 2004. 10:187–192.
22. Dubinett SM, Lee JM, Sharma S, Mulé JJ. Chemokines: can effector cells be redirected to the site of the tumor? Cancer J. 2010. 16:325–335.
23. Ruffini PA, Morandi P, Cabioglu N, Altundag K, Cristofanilli M. Manipulating the chemokine-chemokine receptor network to treat cancer. Cancer. 2007. 109:2392–2404.
24. Zhang T, Somasundaram R, Berencsi K, Caputo L, Rani P, Guerry D, Furth E, Rollins BJ, Putt M, Gimotty P, Swoboda R, Herlyn M, Herlyn D. CXC chemokine ligand 12 (stromal cell-derived factor 1 alpha) and CXCR4-dependent migration of CTLs toward melanoma cells in organotypic culture. J Immunol. 2005. 174:5856–5863.
25. Shurin GV, Ferris RL, Tourkova IL, Perez L, Lokshin A, Balkir L, Collins B, Chatta GS, Shurin MR. Loss of new chemokine CXCL14 in tumor tissue is associated with low infiltration by dendritic cells (DC), while restoration of human CXCL14 expression in tumor cells causes attraction of DC both in vitro and in vivo. J Immunol. 2005. 174:5490–5498.
26. Thanarajasingam U, Sanz L, Diaz R, Qiao J, Sanchez-Perez L, Kottke T, Thompson J, Chester J, Vile RG. Delivery of CCL21 to metastatic disease improves the efficacy of adoptive T-cell therapy. Cancer Res. 2007. 67:300–308.
27. Dieu-Nosjean MC, Antoine M, Danel C, Heudes D, Wislez M, Poulot V, Rabbe N, Laurans L, Tartour E, de Chaisemartin L, Lebecque S, Fridman WH, Cadranel J. Long-term survival for patients with non-small-cell lung cancer with intratumoral lymphoid structures. J Clin Oncol. 2008. 26:4410–4417.
28. Shields JD, Kourtis IC, Tomei AA, Roberts JM, Swartz MA. Induction of lymphoid like stroma and immune escape by tumors that express the chemokine CCL21. Science. 2010. 328:749–752.
29. Hisada M, Yoshimoto T, Kamiya S, Magami Y, Miyaji H, Yoneto T, Tamada K, Aoki T, Koyanagi Y, Mizuguchi J. Synergistic antitumor effect by coexpression of chemokine CCL21/SLC and costimulatory molecule LIGHT. Cancer Gene Ther. 2004. 11:280–288.
30. Yu P, Lee Y, Liu W, Chin RK, Wang J, Wang Y, Schietinger A, Philip M, Schreiber H, Fu YX. Priming of naive T cells inside tumors leads to eradication of established tumors. Nat Immunol. 2004. 5:141–149.