Abstract
A reduction in diaphragm mobility has been identified in patients with chronic obstructive pulmonary disease (COPD) and has been associated with a decline in pulmonary function parameters. However, little information exists regarding the potential role of diaphragm mobility on hypercapnia in COPD. A new method of assessing the mobility of the diaphragm, using ultrasound, has recently been validated. The purpose of the present study was to investigate the relationship between diaphragm mobility and pulmonary function parameters, as well as that between arterial blood gas values and diaphragm mobility, in COPD patients. Thirty seven COPD patients were recruited for pulmonary function test, arterial blood gas analysis and diaphragm mobility using ultrasound to measure the craniocaudal displacement of the left branch of the portal vein. There were significant negative correlations between diaphragmatic mobility and PaCO2 (r = -0.373, P = 0.030). Diaphragmatic mobility correlated with airway obstruction (FEV1, r = 0.415, P = 0.011) and with ventilatory capacity (FVC, r = 0.302, P = 0.029; MVV, r = 0.481, P = 0.003). Diaphragmatic mobility also correlated significantly with pulmonary hyperinflation. No relationship was observed between diaphragm mobility and PaO2 (r = -0.028, P = 0.873). These findings support a possibility that the reduction in diaphragm mobility relates to hypercapnia in COPD patients.
Chronic obstructive pulmonary disease (COPD) is characterized by airflow limitation that is not fully reversible and abnormal inflammatory response of the lungs to noxious particles or gases (1). A number of pathophysiological mechanisms of COPD such as airway obstruction, pulmonary hyperinflation and air trapping, might be involved in this process of impairment of diaphragmatic function (2).
The respiratory muscles constitute a vital component of the respiratory pump. Their contraction during part of the breathing cycle changes the anatomic configuration of the thorax and displaces its components, so that air moves into and out of the gas exchanging portion of the lungs (3, 4). Diaphragm is the principal generator of tidal volume in normal subjects at rest. Studies have shown that the impairment of diaphragm mobility might be associated with alterations in the principal pulmonary function parameters (5, 6). Several methods are used to quantify the diaphragmatic mobility using chest radiographs (7), computed tomography scan (8), and dynamic magnetic resonance imaging (9). Radiological evaluation of diaphragm mobility has traditionally been performed through fluoroscopy (10), but recently ultrasound measurement of the craniocaudal displacement of the left branch of the portal vein has validated as a new method for assessing diaphragm mobility (11). Ultrasonography has several advantages over fluoroscopy, including lack of risk from ionizing radiation, equipment portability, and direct quantitative information (12).
The mechanisms which lead to chronic hypercapnia in patients with COPD are not completely understood. Several factors such as airway obstruction, ventilation-perfusion mismatch, abnormalities in ventilatory control, respiratory muscle weakness, the pattern of breathing, and dynamic pulmonary hyperinflation have been reported to contribute to chronic carbon dioxide retention in patients with COPD (13-15). To our knowledge, there are few reports available concerning the relationships between the diaphragm mobility and hypercapnia in patients with COPD. Therefore, the purpose of this study was designed to investigate the relationship between diaphragm mobility and pulmonary function parameters, as well as that between arterial blood gas values and diaphragm mobility, in COPD patients.
Patient with a diagnosis of COPD, determined according to the American Thoracic Society (ATS) guidelines (1), were recruited from January to December 2010 in the study. The inclusion criteria were a history of smoking, an FEV1 (forced expiratory volume in 1 second)/FVC (forced vital capacity) ratio < 70%, and an FEV1 < 80% of the predicted values. Exclusion criteria were respiratory disorders other than COPD, pulmonary embolism, infectious diseases, malignancy, left ventricular dysfunction, recent surgery, and severe endocrine, hepatic, or renal diseases. All patients were clinically stable COPD with no exacerbation of symptoms and no hospitalization with the preceding 2 months. At recruitment, height and weight were measured and the body mass index (BMI) was calculated (weight [kg]/height [m2]).
All pulmonary function tests were evaluated using a Sensormedics 2400 Unit (Sensormedics; San Diego, CA, USA) that met the standards of the ATS (1). Most pulmonary function tests were obtained on the same day as the ultrasonography and/or arterial blood gas analysis. Three technically acceptable measurements were performed in each patient, and highest one was included to the analyses. Acceptable maneuvers were defined as those with sharp peak in the flow curve and an expiratory duration greater than six seconds. FEV1, FVC, and lung volume were expressed as percentage of predicted values.
Arterial blood samples taken with the patients at rest and breathing room air were analyzed for oxygen (PaO2), carbon dioxide tension (PaCO2), and pH with a standard blood gas analyzer (Radiometer ABL 700 Series; Kopenhagen, Denmark).
Assessment of the mobility of the right hemidiaphragm was performed by ultrasound measurement of the craniocaudal displacement of the left branch of the portal vein, using an ultrasound scanner (ALOKA KEC-620; Tokyo, Japan) in mode B (16). All evaluations were made by the same pulmonologist who was blinded to the pulmonary function status of each patient. The patients were evaluated in the dorsal decubitus position, using a 3.5-MHz convex transducer positioned over the right subcostal region, at an angle of incidence perpendicular to the craniocaudal axis. The position of the left branch was then demarcated with the cursor during forced expiration and inspiration. The mobility of the right hemidiaphragm was defined as the craniocaudal displacement of this branch, in millimeters (Fig. 1). Three measurements were recorded for each patient, and the greatest value was used for analysis.
A statistical software package (SPSS, version 18.0; SPSS Inc; Chicago, IL, USA) was used for all statistical analyses. The data are reported as mean ± standard deviation. Correlations between diaphragmatic mobility and other parameters were assessed using Spearman's correlation test. A P value less than 0.05 was considered statistically significant.
The 37 patients with COPD (31 male and 6 female) were participated in this study. The mean age of the patients was 69.1 ± 9.0 yr, and the mean BMI was 21.2 ± 4.1 kg/m2. All patients presented airflow limitation (mean FEV1/FVC, 48.4% ± 15.7%) and pulmonary hyperinflation (mean total lung capacity [TLC], 119.4% ± 26.5% of predicted; mean residual volume [RV], 145.7% ± 29.7% of predicted) (Table 1).
The mean values of diaphragmatic mobility were 19.8 ± 7.5 mm, and the mean PaCO2 was 45.2 ± 16.0 mmHg. There were significant negative correlations between the diaphragm mobility and PaCO2 in patients with COPD (r = -0.373, P = 0.030) (Fig. 2). However, there was no correlation between diaphragm mobility and PaO2 (r = 0.028, P = 0.873).
Diaphragm mobility correlated with the pulmonary function parameters that quantify airway obstruction (FEV1: r = 0.415, P = 0.011) and pulmonary hyperinflation (RV: r = -0.501, P = 0.021; TLC: r = -0.281, P = 0.030; RV/TLC: r = -0.527, P = 0.001). Diaphragmatic mobility also correlated significantly with ventilator capacity (FVC: r = 0.302, P = 0.029; MVV: r = 0.481, P = 0.003). However, there was no correlation between diaphragm mobility and FEV1/FVC (r = 0.233, P = 0.166) (Table 2). In the multivariate analyses, PaCO2 and RV/TLC were independent factors for the diaphragm mobility.
The present study has shown that COPD patients with reduction in diaphragmatic mobility are associated with PaCO2 values. In addition, our result show that reduced diaphragmatic mobility is associated with airway obstruction and pulmonary hyperinflation.
Expiratory flow limitation is the pathophysiological hallmark of COPD. The disease is associated with systemic manifestations that can result in worsening dyspnea, impaired functional capacity, reduced healthy-related quality of life and increased mortality. Dyspnea could result from a decreased capacity of the respiratory muscle to meet an increased mechanical load. In addition, COPD patients commonly present increased airflow resistance as well as air trapping and pulmonary hyperinflation. Pulmonary hyperinflation reduces the flow and pressure-generating capacity of the diaphragm (17). As COPD patients develop hyperinflation, their diaphragm becomes flatter and shorter. COPD patients have decreased diaphragm mobility when compared with healthy humans (6, 9). The reduced diaphragmatic mobility observed in COPD patients might be attributable to excessive actin-myosin filament overlap and sarcomere remodeling (18, 19). The main cause of the reduction in diaphragmatic mobility is shortening of the apposition zone resulting from the absence of the piston-like movement of the diaphragm (20). A recent study demonstrated that reduction in diaphragm mobility correlated with airway obstruction, pulmonary hyperinflation and ventilator capacity (5). These findings are consistency with our results showing that diaphragm mobility is positively correlated with airway obstruction and ventilator capacity. Reduction in diaphragm mobility in patients with COPD is negatively correlated with pulmonary function parameters related to lung hyperinflation. Although FEV1 is essential to diagnosis and quantifying COPD-related ventilator impairment (1), it does not adequately reflect the systemic manifestation of COPD (21). Therefore, it has been proposed that other parameters should be evaluated in order to measure changes in the functional status of COPD patients. The results of the present study suggest that diaphragmatic mobility is another parameter that could provide information on respiratory mechanics and functional capacity in COPD patients.
Several factors such as airway obstruction, ventilation-perfusion mismatch, abnormalities in ventilatory control, respiratory muscle weakness, pattern of breathing, and dynamic pulmonary hyperinflation have been reported to contribute to carbon dioxide retention in patients with COPD (13-15). A previous study demonstrated that hypercapnia increases with the severity of the obstruction, obesity, and inspiratory muscle weakness (22). Hypercapnic respiratory failure due to inspiratory muscle weakness is associated with morbidity in patients with COPD (23). Rapid and shallow breathing in patients with COPD is the consequence of an excessive load imposed on the inspiratory muscles. The shallow breathing reduces alveolar ventilation and predisposes to hypercapnia. A study demonstrated that, in patients with stable COPD, PaCO2 was significantly related to positive end expiratory alveolar pressure which suggests that dynamic pulmonary hyperinflation could play a part in chronic carbon dioxide retention (24). To our knowledge, however, no previous studies have specifically examined the correlations between diaphragm mobility and PaCO2 values. Our results show that significant negative correlations between the diaphragm mobility and PaCO2 levels in patients with COPD.
In conclusion, ultrasonography was used to determine the diaphragm mobility in this study. Our study demonstrates that the reduction of diaphragm mobility in patients with COPD is associated with carbon dioxide retention. In addition, diaphragmatic mobility correlates with airway obstruction, ventilatory capacity and pulmonary hyperinflation. These findings support a possibility that the reduction in diaphragm mobility relates to hypercapnia in COPD patients. Further studies are required to better understand the diaphragm mobility on hypercapnia in patients with COPD.
Figures and Tables
Fig. 1
B-mode ultrasonography measurement of craniocaudal displacement of the left intrahepatic portal vein branch. The line shows displacement of the left branch of the portal vein during maximal inspiratory and expiratory breathing in the sagittal plane. The P cursor marks the initial position of this vessel during maximal expiration, and another cursor (+) marks the position of the vessel during maximal inspiration. The craniocaudal displacement of this branch was recorded in millimeters.
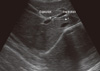
Fig. 2
The relationship between diaphragmatic mobility and the PaCO2. There was a negative linear correlation between the two measurements (r = -0.373, P = 0.030). PaCO2, arterial carbon dioxide tension.
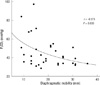
AUTHOR SUMMARY
Influence of Diaphragmatic Mobility on Hypercapnia in Patients with Chronic Obstructive Pulmonary Disease
Hyun Wook Kang, Tae Ok Kim, Bo Ram Lee, Jin Yeong Yu, Su Young Chi, Hee Jung Ban, In Jae Oh, Kyu Sik Kim, Yong Soo Kwon, Yu Il Kim, Young Chul Kim and Sung Chul Lim
A reduction in diaphragm mobility has been identified in patients with chronic obstructive pulmonary disease (COPD) and has been associated with a decline in pulmonary function parameters. However, little information exists regarding the potential role of diaphragm mobility on hypercapnia in COPD. We investigated the relationship between diaphragm mobility and arterial blood gas values in COPD patients. There were significant negative correlations between diaphragmatic mobility and PaCO2. Also, diaphragmatic mobility correlated with airway obstruction, ventilatory capacity and hyperinflation of lungs.
References
1. Rabe KF, Hurd S, Anzueto A, Barnes PJ, Buist SA, Calverley P, Fukuchi Y, Jenkins C, Rodriguez-Roisin R, van Weel C, Zielinski J. Global strategy for the diagnosis, management, and prevention of chronic obstructive pulmonary disease. Am J Respir Crit Care Med. 2007. 176:532–555.
2. Iwasawa T, Kagei S, Gotoh T, Yoshiike Y, Matsushita K, Kurihara H, Saito K, Matsubara S. Magnetic resonance analysis of abnormal diaphragmatic motion in patients with emphysema. Eur Respir J. 2002. 19:225–231.
3. Roussos C, Macklem PT. The respiratory muscles. N Engl J Med. 1982. 307:786–797.
4. Rochester DF. The diaphragm: contractile properties and fatigue. J Clin Invest. 1985. 75:1397–1402.
5. Dos Santos Yamaguti WP, Paulin E, Shibao S, Chammas MC, Salge JM, Ribeiro M, Cukier A, Carvalho CR. Air trapping: The major factor limiting diaphragm mobility in chronic obstructive pulmonary disease patients. Respirology. 2008. 13:138–144.
6. Unal O, Arslan H, Uzun K, Ozbay B, Sakarya ME. Evaluation of diaphragmatic movement with MR fluoroscopy in chronic obstructive pulmonary disease. Clin Imaging. 2000. 24:347–350.
7. Singh B, Eastwood PR, Finucane KE. Volume displaced by diaphragm motion in emphysema. J Appl Physiol. 2001. 91:1913–1923.
8. Cassart M, Pettiaux N, Gevenois PA, Paiva M, Estenne M. Effect of chronic hyperinflation on diaphragm length and surface area. Am J Respir Crit Care Med. 1997. 156:504–508.
9. Suga K, Tsukuda T, Awaya H, Takano K, Koike S, Matsunaga N, Sugi K, Esato K. Impaired respiratory mechanics in pulmonary emphysema: evaluation with dynamic breathing MRI. J Magn Reson Imaging. 1999. 10:510–520.
10. Harris RS, Giovannetti M, Kim BK. Normal ventilatory movement of the right hemidiaphragm studied by ultrasonography and pneumotachography. Radiology. 1983. 146:141–144.
11. Toledo NS, Kodaira SK, Massarollo PC, Pereira OI, Mies S. Right hemidiaphragmatic mobility: assessment with US measurement of craniocaudal displacement of left branches of portal vein. Radiology. 2003. 228:389–394.
12. Houston JG, Fleet M, Cowan MD, McMillan NC. Comparison of ultrasound with fluoroscopy in the assessment of suspected hemidiaphragmatic movement abnormality. Clin Radiol. 1995. 50:95–98.
13. West JB. Causes of carbon dioxide retention in lung disease. N Engl J Med. 1971. 284:1232–1236.
14. Rochester DF, Braun NM. Determinants of maximal inspiratory pressure in chronic obstructive pulmonary disease. Am Rev Respir Dis. 1985. 132:42–47.
15. Gorini M, Spinelli A, Ginanni R, Duranti R, Gigliotti F, Scano G. Neural respiratory drive and neuromuscular coupling in patients with chronic obstructive pulmonary disease (COPD). Chest. 1990. 98:1179–1186.
16. Toledo NS, Kodaira SK, Massarollo PC, Pereira OI, Mies S. Right hemidiaphragmatic mobility: assessment with US measurement of craniocaudal displacement of left branches of portal vein. Radiology. 2003. 228:389–394.
17. McKenzie DK, Butler JE, Gandevia SC. Respiratory muscle function and activation in chronic obstructive pulmonary disease. J Appl Physiol. 2009. 107:621–629.
18. Hoppin FG Jr. Theoretical basis for improvement following reduction pneumoplasty in emphysema. Am J Respir Crit Care Med. 1997. 155:520–525.
19. Orozco-Levi M, Gea J, Lloreta JL, Félez M, Minguella J, Serrano S, Broquetas JM. Subcelullar adaptation of the human diaphragm in chronic obstructive pulmonary disease. Eur Respir J. 1999. 13:371–378.
20. Gauthier AP, Verbanck S, Estenne M, Segebarth C, Macklem PT, Paiva M. Three-dimensional reconstruction of the in vivo human diaphragm shape at different lung volumes. J Appl Physiol. 1994. 76:495–506.
21. Gross NJ. Extrapulmonary effects of chronic obstructive pulmonary disease. Curr Opin Pulm Med. 2001. 7:84–92.
22. Bégin P, Grassino A. Inspiratory muscle dysfunction and chronic hypercapnia in chronic obstructive pulmonary disease. Am Rev Respir Dis. 1991. 143:905–912.
23. Zielinski J, MacNee W, Wedzicha J, Ambrosino N, Braghiroli A, Dolensky J, Howard P, Gorzelak K, Lahdensuo A, Strom K, Tobiasz M, Weitzenblum E. Causes of death in patients with COPD and chronic respiratory failure. Monaldi Arch Chest Dis. 1997. 52:43–47.
24. Haluszka J, Chartrand DA, Grassino AE, Milic-Emili J. Intrinsic PEEP and arterial PCO2 in stable patients with chronic obstructive pulmonary disease. Am Rev Respir Dis. 1990. 141:1194–1197.