Abstract
The peroxisome proliferator activated receptor (PPAR)γ agonist is used as antidiabetic agent with antihyperglycemic and antihyperinsulinemic actions. Beyond these actions, antifibrotic effects have been reported. We examined antifibrotic effects of PPARγ agonist and interaction with angiotensin receptor antagonist in the unilateral ureteral obstruction (UUO) model. After UUO, mice were divided to four groups: no treatment (CONT), pioglitazone treatment, L158809 treatment, and L158809+ pioglitazone treatment. On day 14, CONT mice showed severe fibrosis and all treated mice showed decreased fibrosis. The immunohistochmistry of PAI-1, F4/80 and p-Smad2 demonstrated that their expressions were increased in CONT group and decreased in the all treated groups compared to CONT. PAI-1 and p-Smad2 determined from Western blotting, among treated groups, was decreased compared to CONT group. The expression of TGF-β1 from real time RT PCR showed markedly increased in the CONT group and decreased in all treated groups compared to CONT. These data suggest the pioglitazone inhibited tubulointerstitial fibrosis, however, the synergism between pioglitazone and L158809 is not clear. Considering decreased expression of PAI-1 and TGF-β/Smad2 in the treated groups, PAI-1 and TGF-β are likely linked to the decreased renal tubulointerstitial fibrosis. According to these results, the PPARγ agonist might be used in the treatment of renal fibrotic disease.
The peroxisome proliferator activated receptor (PPAR)γ is a ligand specific transcription factor. It plays an important role in the regulation of diabetes mellitus (DM). It modulates insulin sensitivity, cell growth, inflammation, and adipocyte differentiation and lipid metabolism (1). PPARγ agonist is used worldwide for treatment of DM. In addition, PPARγ agonist has antifibrotic effects in both diabetic nephropathy and non-diabetic nephropathy. Animal models of diabetic nephropathy treated with PPARγ agonist have demonstrated decreased proteinuria and glomerular matrix deposition and glomerulosclerosis; these results appear to be independent of the antidiabetic effect of PPARγ (2, 3). In the 5/6-nephrectomy model, activation of PPARγ has been shown to reduce glomerulosclerosis (4). In addition, in vitro experiments have demonstrated that PPAR-γ activation had antiproliferative (5) and antifibrotic effects on the mesangial cells (6). Furthermore PPAR-γ activation reduced type I collagen synthesis and secretion. Its action probably was linked to a TGF-β1-dependent mechanisms (7). Based on these results, we speculate that the antifibrotic effects of PPAR-γ are linked to TGF-β. However, the mechanism involved in the antifibrotic effects has been little known.
Tubulointerstitial lesion containing tubular atrophies and interstitial fibrosis in the kidney are the final common pathways that lead to progressive renal failure regardless of the primary cause of renal disease (8). The unilateral ureteral obstruction (UUO) model induces interstitial inflammation and fibrosis; it has been used as a model for tubulointerstitial fibrosis. The expressions of tissue growth factor (TGF)-β and plasminogen activator inhibitor-1 (PAI-1) are increased in the UUO model (9, 10) and with decreased fibrosis, their expression decreases.
In this study, we evaluated whether the PPARγ agonist, pioglitazone, had antifibrotic and antiinflammatory effects in the UUO mouse model. In addition, we studied whether there are synergistic effects with angiotensin receptor blocker (ARB), widely used for anti-inflammatory and antifibrotic treatment in kidney diseases. In addition, we evaluated the mechanism involved in the antifibrotic effects of the PPARγ agonist that correlate with TGF-β and PAI-1.
Adult male wild type C57BL/6 mice, 10 to 12 weeks of age were used for the experiments. The mice were housed in microisolator cages in a pathogen-free barrier facility. The mice had free access to tap water and standard mouse chow (RP5015; PMI feeds Inc, St. Louis, MO, USA) or prepared chow containing the PPARγ agonist, pioglitazone. The mice were maintained in a temperature-controlled facility with 12-hr light/12-hr dark cycles. All procedures were followed the rules of the Inha University animal experiment committee.
The mice underwent UUO (n=40) under general anesthesia and sterile conditions (11). After the UUO, they were divided into 4 groups. The first group (n=10) did not receive any treatment (CONT). The second group (n=10) received the PPAR-γ agonist, pioglitazone, p.o. (30 mg/kg/day) (pioglitazone treated group). The third group (n=10) received the angiotensin receptor antagonist, L158809 (1.5 mg/kg/day, drinking water [DW]) (L158809 treated group). The fourth group (n=10) received combined therapy including pioglitazone and L158809 of the same dose (combined treatment group). On day five or 14 days after the surgery, the mice were sacrificed and both the obstructed and nonobstructed contralateral kidneys were collected for morphological, immunostaining, and molecular assessment. Four mice were underwent sham operation as normal controls.
For light microscopy, the tissue fixed in 4% paraformaldehyde was embedded in paraffin and 4 µm sections were prepared. After Masson's trichrome staining, the degree of tubulointerstitial fibrosis and injury were scored from 0 to 4+ on the day 5 specimens. Fibrosis and injury were assessed as 0 for 0%, 1 for <25%, 2 for 25 to 50%, 3 for >50 to 75%, and 4 for >75% for each field with tubulointerstitial fibrosis. Interstitial fibrosis, in the cortex, was assessed by point counting (12) in the cortical area on the day 14 specimens. All sections were examined without knowledge of the treatment protocol.
For immunostaining for PAI-1, antigen retrieval was performed using microwaves for 10 min. The slides were exposed to Power Block (BioGenex Laboratories, San Ramon, CA, USA) for 45 min and incubated with rabbit anti-rat PAI-1 antibody (1:50, American Diagnostica, Inc., Greenwich, CT, USA) at 4℃ overnight followed by biotinylated goat anti-rabbit immunoglobulin (BioGenex Laboratories) for 45 min and peroxidase-conjugated streptavidin for 45 min. Diaminobenzidine was added as a chromogen. Positive PAI-1 staining was graded from 0 to 4+ (grade 0, No significant increase, diffuse very weak stain similar to nonobstructed kidney; grade 1, very weak focal staining, <5% increase staining; grade 2, mild/moderate intensity in <20% of areas; grade 3, moderate intensity in 20-50%; grade 4, moderate intensity >50%).
Infiltration of macrophages was detected by rat anti-mouse F4/80 antibody (1:2,000; Serotec Inc., Raleigh, NC, USA) incubated at room temperature for 1 hr, followed by Envision kit (Dako, Carpinteria, CA, USA). Infiltrating macrophages, in the interstitium, were counted and expressed as the number of macrophages per high-power field.
For immunostaining of phosphorylated Smad2 (p-Smad2), antigen retrieval was performed by microwave for 10 min. Rabbit anti-human p-Smad 2 (Cell Signaling Technology, Beverly, MA, USA) was used at room temperature overnight followed by Envision kit (Dako). Positive p-Smad2 staining was graded from 0 to 3+ (grade 0, No significant increase, diffuse very weak nuclear stain similar to nonobstructed kidney; grade 1, nuclear staining with mild intensity <20% increase staining; grade 2, nuclear staining with moderate intensity in ≤50% of areas; grade 3, nuclear staining with moderate intensity in >50%).
1) p-Smad 2, β-actin and PAI-1: The proteins were extracted using PRO-PREP protein extraction solution (Intron, Seongnam, Korea) from the frozen kidney tissues. The protein concentration was measured using Dc Protein Assay kit (Bio-Rad Laboratories, Hercules, CA, USA). Remaining procedures was performed to an established procedure (13). The primary antibodies used were as follows: rabbit anti-p-Smad2 polyclonal antibody, 1:500 (Upstate Biotechnology, Lake Placid, NY, USA), mouse anti-β-actin monoclonal antibody, 1:5,000, (Sigma, St. Louis, MO, USA), rabbit anti-PAI-1 antibody, 1:100 (American Diagnostica). 2) The intensities of individual bands were semiquantified using the TINA 2.0 program.
Total RNA was obtained from frozen mouse kidney tissues (normal and obstructed kidney) using the TRIZOL (Invitrogen, San Diego, CA, USA) according to the manufacturer's protocols. Mouse β-actin mRNA was used as an internal control and also used to assess RNA integrity.
One micrograms of total RNA was reverse transcribed into cDNA using RT system kit (Promega, Madison, WI, USA). Reverse transcription was carried out in a total volume of 25 µL containing 10 mM Tris-HCl, (pH 8.8), 50 mM KCl, 5 mM MgCl2, 1 mM each dNTPs, 0.5 µg oligo (dT) primers, 25 U RNase inhibitor, and 15 U AMV reverse transcriptase for 1 hr at 37℃, followed by 10 min at 95℃. The polymerase chain reaction (PCR) was performed using 1 µL cDNA in a total volume of 25 µL in the presence of 12.5 µL 2×iQ Supermix (Bio-rad), 200 nM TGF-β primer set, 100 nM TGF-β probe. The PCR condition was 40 cycles at 95℃ for 30 sec, 60℃ for 1 min using iCycler iQ real-time PCR detection system (Bio-Rad). Amplification of β-actin was carried out in parallel with the genes of interest. Each assay included a no template control and all measurements were performed in duplicate. All samples with a coefficient of variation >10% were retested. Primers and probes used for Real-Time RT-PCR were purchased from TIB MOLBIOL (Berlin, Germany) and their sequences were represented as following.
The trichrome staining revealed interstitial fibrosis and tubular atrophy at 5 days in all of the groups. There was no significant statistical difference among the groups. On the day 14, the interstitial fibrosis was markedly increased in the CONT group (0.27±0.03). The pioglitazone treated group revealed decreased fibrosis compared to CONT (0.15±0.02, P<0.05 vs. CONT). The interstitial fibrosis was more decreased in the L158809 treated group and combined treatment group compared to CONT and pioglitazone treated group (L158809 treated group; 0.06±0.004, combined treatment group; 0.09±0.01, P<0.05 vs. CONT and pioglitazone treated group) (Fig. 1).
The number of macrophages was increased 5 days after UUO compared to contralateral non-obstructed kidney. However, there was no significant difference among treated and non-treatment groups. By 14-day, the number of macrophages was significantly increased in the CONT group (117.9±9.5/HPF). The all treated groups showed significantly small macrophage numbers compared to CONT (pioglitazone treated group 63.9±5.4/HPF, L158809 treated group 80.9±10.2/HPF, combined treatment group 76.5±4.4/HPF, P<0.05 vs. CONT) (Fig. 2).
PAI-1 expression of IHC was slightly increased at 5-day. However, there was no statistical difference between the treated groups. The PAI-1 was detected at the cytoplasm of damaged or atrophic tubular epithelial cells, interstitial inflammatory cells and parietal epithelial cells. Their expression was significantly increased at 14-day in the CONT group (2.85±0.12). PAI-1 expression cells were similar to the CONT but PAI-1 intensity was decreased compared to CONT in the pioglitazone treated groups (1.91±0.08, P<0.05 vs. CONT). For the L158809 and in the combined treatment groups, the PAI-1 expression patterns were similar to CONT. Its intensity was decreased but there was no statistically significant difference (L158809; 2.65±0.10, P=0.3 vs. CONT, combined treated group; 2.52±0.16, P=0.06 vs. CONT) (Fig. 3). The PAI-1 determined from Western blotting, among the day 14 all treated groups (pioglitazone treated group, L158809 treated group, and combined treatment group), was decreased compared to CONT group (P<0.05); the L158809 treated group showed most decreased expression, the combined treatment group had the second most decreased expression and pioglitazone treated group had the smallest decrease in expression compared to CONT (Fig. 4).
P-Smad2 expression of IHC, a marker of TGF-β signaling activation, was detected at the nuclei of damaged or atrophied tubular epithelial cells and interstitial inflammatory cells. Its expression was not increased at day 5 compared to normal control. At day 14, their intensity was increased in the CONT group. In the all of treated groups (pioglitazone treated group, L158809 treated group, combined treatment group), the p-Smad2 expressions were decreased compared to CONT (Fig. 5).
For the day 14 specimens, the p-Smad2 from Western blotting analysis showed that the markedly increased in the CONT group compared to normal control and decreased in the combined treatment group compare to CONT (Fig. 6).
On the day 5, TGF-β1 expression was increased in all groups. However, there was no statistically significant difference among the groups. For the day 14 samples, the TGF-β1 expression was increased more in the CONT group and decreased in all of the treated groups (pioglitazone treated group, L158809 treated group, combined treatment group, P<0.05 vs. CONT). The relative value for the real time RT PCR of TGF-β1 was the following: CONT, 1.52±0.53; pioglitazone treated group, 0.83±0.12; L158809 treated group, 0.63±0.13; and combined treated group, 0.54±0.06 (Fig. 7).
PPARγ agonists are used worldwide for their antidiabetic activity including the regulation of insulin sensitivity and lipid metabolism. In addition to these effects, PPARγ has antifibrotic and anti-inflammatory effects. Clinical and animal studies have shown that PPARγ agonists not only regulate insulin sensitivity but also may have a protective effect against kidney damage, such as reduced microalbuminuria and delaying progression to diabetic nephropathy in humans with type 2 diabetes and diabetic animal model (2, 14-16). These renoprotective effects appear to be independent from blood glucose control activity of PPARγ. In vitro studies also have revealed that PPAR-γ agonists can inhibit cell proliferation and suppress the expression of extracellular matrix components (7, 17). UUO is a well established experimental model of progressive tubulointerstitial fibrosis. In this study, we observed the antifibrotic and anti-inflammatory effects of PPARγ agonist, pioglitazone. Pioglitazone had an antifibrotic effect on the UUO kidney. The interstitial fibrosis was decreased in the pioglitazone-treated group compared the CONT group.
The mechanism underlying the antifibrotic effects of the PPARγ agonist has little known. TGF-β has been known to be increased in progressive renal disease and PAI-1 plays an important role in the progression of renal fibrosis. Angiotensin, aldosterone, and TGF-β induce PAI-1 expression, and inhibition of these compounds resulted in decreased PAI-1 and sclerosis in experimental models of chronic kidney disease (18-20). In this connection, we tried to evaluate that TGF-β and PAI-1 were linked to the antifibrotic effects of pioglitazone.
The results of our study showed that PAI-1 and TGF-β expression were increased in the UUO kidneys, and decreased in all of the treatment groups including the PPARγ agonist treated group. Based on these results, the mechanism underlying the antifibrotic effects of pioglitazone appeared to be correlated with the decrease of TGF-β and PAI-1. There are several prior studies that support these findings. In vitro, pioglitazone inhibited fibronectin production induced by TGF-β in mesangial cells (7). PPARγ agonists had an antifibrotic effect on human proximal tubular cells in a high glucose environment by inhibiting the increase in AP-1 and TGF-β1, and decreasing the production of the fibronectin (21). The PPARγ agonist also inhibited TGF-β1 mediated alpha smooth muscle actin, fibronectin, and PAI-1 expression in the mesangial cells (22). In the 5/6 nephrectomy model, PPARγ ameliorated the development of sclerosis and this effect was linked to decreased TGF-β and PAI-1 (4).
Our findings showed that the p-Smad2 expression was also decreased in the PPARγ agonist treated group. The Smad proteins are essential mediators and regulators of the intracellular signaling pathways, acting as transcription factors of TGF-β-mediated responses, including the fibrotic process (23). After TGF-β binds to the TGF-β receptor II, phosphorylation of the TGF-β receptor I, and the intracellular substrates, Smad2 and Smad3, occurs. When activated, these Smads with their common partner, Smad4 complexes, are translocated to the nucleus, where they regulate transcriptional responses together with additional DNA binding cofactors. An in vitro study showed that PPARγ agonists inhibited the Smad signaling pathway in the renal fibroblasts and blocked TGF-beta/Smad-mediated gene transcription in mesangial cells (22). Therefore, PPARγ appears to have antifibrotic effects correlated with PAI-1, and TGF-β/Smad2 signaling.
The PPARγ agonist also has anti-inflammatory effects. Our results showed that the number of infiltrated interstitial macrophage was markedly decreased in the pioglitazone treated groups. PAI-1 affects recruitment of interstitial macrophages and myofibroblasts, a potential source of both PAI-1, TGF-β and other profibrotic cytokines such as PDGFs, macrophage chemoattractant protein-1 (MCP-1), osteopontin, and integrins (24). Persistent infiltration of activated macrophages is profibrotic and closely correlated with progressive fibrosis in the kidney (25). Therefore inhibition of macrophage accumulation can produce not only antiinflammatory but also antifibrotic effects.
Angiotensin II is involved in the pathogenesis of renal disease, through the regulation of two key processes inflammation and fibrosis. Treatment of angiotensin II receptor antagonist ameliorates the renal interstitial fibrosis caused by UUO by decreasing the TGF-β and the number of interstitial macrophages (26). We also evaluated the antifibrotic effects of ARB, L158809. Renal interstitial fibrosis and the number of infiltrating macrophage were decreased in the L158809 treated group. Interstitial fibrosis was decreased more in the L158809 treated group compared to the pioglitazone treated group. However, the number of interstitial macrophages was greater in the L158809 treated group than in the pioglitazone treated group. The PAI-1 and TGF-β expression were also decreased in the L158809 treated group. However, the synergistic effects of pioglitazone and L158809 were not clear; a synergistic effect was only observed with TGF-β expression. Combination of pioglitazone and L158809 is almost equally effective compared to their single applications concerning attenuation of fibrosis, infiltration of macrophages, PAI-1 and TGF-β/p-Smad2 expression after UUO. The synergistic effect between pioglitazone and L158809 was not clear in our study. The studies about synergism of these two drugs are few and the results are still controversial. In vitro, combination therapy of a PPARγ agonist and an ARB suppresses proinflammatory signaling and stimulates expression of Smad7 in human peritoneal mesothelial cells (27). However, in vivo examination of ischemia induced brain injury of rat, there was no synergistic effect between candesartan and pioglitazone (28). The synergistic effect with ARB is not clear. It needs further investigation.
In the conclusion, the PPARγ agonist inhibited tubulointerstitial fibrosis and infiltration of interstitial macrophages in UUO model. The findings of decreased expression of PAI-1, TGF-β, and p-Smad2 in the treated groups, suggest that PAI-1 and TGF-β/Smad2 were linked to the decreased renal tubulointerstitial fibrosis observed. Therefore, PPARγ agonists might be used for the treatment of renal fibrotic diseases other than those associated with diabetes mellitus.
Figures and Tables
Fig. 1
Tubulointerstititial changes in obstructed kidneys. (A) At day 5, tubulointerstitial fibrosis is slightly increased at all groups with no significant difference among groups. At day 14, CONT mice show severe progressive fibrosis and three treated groups show decreased fibrosis compared to CONT. a, sham operated kidney; b, CONT at day 5; c, pioglitazone treated group at day 5; d, L158809 treated group at day 5; e, combined treatment group at day 5; f, CONT at day 14; g, pioglitazone treated group at day 14; h, L158809 treated group at day 14; i, combined treatment group at day 14 (Masson-trichrome stain, ×200). (B) Point counting scoring of fibrosis at day 14 shows less fibrosis in all treated groups compared to CONT (Comb; combined treatment group) (*P<0.05 vs. CONT).
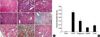
Fig. 2
Interstitial macrophage numbers in obstructed kidneys at day 14. CONT mice show markedly increased number of interstitial macrophages and all treated groups show decreased number of macrophages compared to CONT (*P<0.05 vs. CONT).
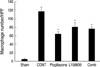
Fig. 3
PAI-1 protein expression by Immunohistochemical staining. (A) PAI-1 expression is identified in the cytoplasm of atrophic tubular epithelial cells, interstitial inflammatory cells and parietal epithelial cells of glomerulus. At day 5, PAI-1 expression is minimally increased with no significant difference among groups. At day 14, PAI-1 expression is increased at CONT. At pioglitazone treated group, the PAI-1 expressions is decreased comparing to CONT. a, sham operated kidney; b, CONT at day 5; c, pioglitazone treated group at day 5; d, L158809 treated group at day 5; e, combined treatment group at day 5; f, CONT at day 14; g, pioglitazone treated group at day 14; h, L158809 treated group at day 14; i, combined treatment group at day 14 (immunohistochemical staining for PAI-1, ×200). (B) Semiquantative grading of PAI-1 expression at day 14 shows significantly decreased PAI-1 expression only at the pioglitazone treated groups compared to CONT (*P<0.05 vs. CONT).
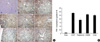
Fig. 4
PAI-1 protein expression by western blotting at day 14. PAI-1 expression is decreased at all treated groups compared to CONT (*P<0.05 vs. CONT).
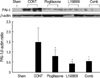
Fig. 5
P-Smad2 expression by Immunohistochemical staining. P-Smad2 is detected at the nuclei of tubular epithelial cells and interstitial inflammatory cells. (A) At day 5, p-Smad2 expression of IHC at treated groups is not increased compared to normal control. Weak cytoplasm stainings are present at all groups. At day 14, p-Smad2 expression is increased at CONT. At all treated groups, the p-Smad2 expressions are decreased compared to CONT. a, sham operated kidney; b, CONT at day 5; c, pioglitazone treated group at day 5; d, L158809 treated group at day 5; e, combined treatment group at day 5; f, CONT at day 14; g, pioglitazone treated group at day 14; h, L158809 treated group at day 14; i, combined treatment group at day 14 (immunohistochemical staining for p-Smad 2, ×200). (B) Semiquantative grading of p-Smad2 expression at day 14 shows significantly decreased p-Smad2 expression at the all treated groups compared to CONT (*P<0.05 vs. CONT) .
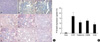
References
1. Guan Y, Zhang Y, Breyer MD. The role of PPARs in the transcription control of the cellular processes. Drug News Perspect. 2002. 15:147–154.
2. McCarthy KJ, Routh RE, Shaw W, Walsh K, Welbourne TC, Johnson JH. Troglitazone halts diabetic glomerulosclerosis by blockade of mesangial expansion. Kidney Int. 2000. 58:2341–2350.


3. Smith SA, Lister CA, Toseland CD, Buckingham RE. Rosiglitazone prevents the onset of hyperglycemia and proteinuria in the Zucker diabetic fatty rats. Diabetes Obes Metab. 2000. 2:363–372.
4. Ma LJ, Marcantoni C, Linton MF, Fazio S, Fogo AB. Peroxisome proliferator-activated receptor-gamma agonist troglitazone protects against non-diabetic glomerulosclerosis in rats. Kidney Int. 2001. 59:1899–1910.
5. Ghosh SS, Gehr TW, Ghosh S, Fakhry I, Sica DA, Lyall V, Schoolwerth AC. PPARgamma ligand attenuates PDGF-induced mesangial cell proliferation: role of MAP kinase. Kidney Int. 2003. 64:52–62.
6. Routh RE, Johnson JH, McCarthy KJ. Troglitazone suppresses the secretion of type I collagen by mesangial cells in vitro. Kidney Int. 2002. 61:1365–1376.


7. Guo B, Koya D, Isono M, Sugimoto T, Kashiwagi A, Haneda M. Peroxisome proliferator-activated receptor-gamma ligands inhibit TGF-beta 1-induced fibronectin expression in glomerulomesangial cells. Diabetes. 2004. 53:200–208.
8. Eddy AA. Experimental insights into the tubulointerstitial disease accompanying primary glomerular lesions. J Am Soc Nephrol. 1994. 5:1273–1287.


9. Miyajima A, Chen J, Lawrence C, Ledbetter S, Soslow RA, Stern J, Jha S, Pigato J, Lemer ML, Poppas DP, Vaughan ED, Felsen D. Antibody to transforming growth factor-beta ameliorates tubular apoptosis in unilateral ureteral obstruction. Kidney Int. 2000. 58:2301–2313.
10. Duymelinck C, Dauwe SE, De Greef KE, Ysebaert DK, Verpooten GA, De Broe ME. TIMP-1 gene expression and PAI-1 antigen after unilateral ureteral obstruction in adult male rat. Kidney Int. 2000. 58:1186–1201.
11. Ma J, Nishimura H, Fogo A, Kon V, Inagami T, Ichikawa I. Accelerated fibrosis and collagen deposition develop in the renal interstitium of angiotensin type 2 receptor null mutant mice during ureteral obstruction. Kidney Int. 1998. 53:937–944.
12. Bonegio RG, Fuhro R, Wang Z, Valeri CR, Andry C, Salant DJ, Lieberthal W. Rapamycin ameliorates proteinuria associated tubulointerstitial fibrosis inflammation and fibrosis in experimental membranous nephropathy. J Am Soc Nephrol. 2005. 16:2063–2072.
13. Yang J, Liu Y. Dissection of key events in tubular epithelial to myofibroblast transition and its implications in renal interstitial fibrosis. Am J Pathol. 2001. 159:1465–1475.


14. Buckinham RE, Al-Barazanji KA, Toseland CD, Slaughter M, Connor SC, West A, Bond B, Turner NC, Clapham JC. Peroxisom proliferator-activated receptor gamma agonist, rosiglitazone, protects against nephropathy and pancreatic islet abnormalities in Zucker fatty rats. Diabetes. 1998. 47:1326–1334.
15. Fujii M, Takemura R, Yamaguchi M, Hasegawa G, Shigeta H, Nakano K, Kondo M. Troglitazone (CS-045) ameliorates albuminuria in streptozotocin-induced diabetic rats. Metabolism. 1997. 46:981–983.


16. Imano E, Kanda T, Nakatani Y, Nishida T, Arai K, Motomura M, Kajimoto Y, Yamasaki Y, Hori M. Effect of troglitazone halts diabetic glomerulosclerosis by blockade of mesangial expansion. Diabetes Care. 1998. 21:2135–2139.
17. Zafirious S, Stanners SR, Saad S, Polhill TS, Poronnik P, Pollock CA. Pioglitazone inhibits cell growth and reduces matrix production in human kidney fibroblasts. J Am Soc Nephrol. 2005. 16:638–645.
18. Eddy AA. Plasminogen activator inhibitor-1 and the kidney. Am J Physiol Renal Physiol. 2002. 283:F209–F220.


19. Fogo AB. The role of angiotensin II and plasminogen activator inhibitor-1 in progressive glomerulosclerosis. Am J Kidney Dis. 2000. 35:179–188.


20. Brown NJ, Vaughan DE, Fogo AB. Aldosterone and PAI-1: implications for renal injury. J Nephrol. 2002. 15:230–235.
21. Panchapakesan U, Sumual S, Pollock CA, Chen X. PPARgamma agonists exert antifibrotic effects in renal tubular cells exposed to high glucose. Am J Physiol Renal Physiol. 2005. 289:F1153–F1158.
22. Li Y, Wen X, Spataro BC, Hu K, Dai C, Liu Y. Hepatocyte growth factor is a downstream effector that mediates the antifibrotic action of peroxisome proliferator-activated receptor-gamma agonists. J Am Soc Nephrol. 2006. 17:54–65.
23. Massague J, Chen YG. Controlling TGF-beta signaling. Genes Dev. 2000. 14:627–644.
25. Diamond JR. Macrophages and progressive renal disease in experimental hydronephrosis. Am J Kidney Dis. 1995. 26:133–140.


26. Ishidoya S, Morrissey J, McCracken R, Reyes A, Klahr S. Angiotensin II receptor antagonist ameliorates renal tubulointerstitial fibrosis caused by unilateral ureteral obstruction. Kidney Int. 1995. 47:1285–1294.


27. Yao Q, Ayala ER, Qian JQ, Stenvinkel P, Axelsson J, Lindholm B. A combination of PPAR gamma agonist and an angiotensin receptor blocker attenuates proinflammatory signaling and stimulates expression of Smad7 in human peritoneal mesothelial cells. Clin Nephrol. 2007. 68:295–301.
28. Schmerbach K, Schefe JH, Krikov M, Müller S, Villringer A, Kintscher U, Unger T, Thoene-Reineke C. Comparison between single and combined treatment with candesartan and pioglitazone following transient focal ischemia rat brain. Brain Res. 2008. 7:225–233.