Abstract
This study aimed to characterize and MRI track the mesenchymal stem cells labeled with chitosan-coated superparamagnetic iron oxide (Chitosan-SPIO). Chitosan-SPIO was synthesized from a mixture of FeCl2 and FeCl3. The human bone marrow derived mesenchymal stem cells (hBM-MSC) were labeled with 50 µg Fe/mL chitosan-SPIO and Resovist. The labeling efficiency was assessed by iron content, Prussian blue staining, electron microscopy and in vitro MR imaging. The labeled cells were also analyzed for cytotoxicity, phenotype and differentiation potential. Electron microscopic observations and Prussian blue staining revealed 100% of cells were labeled with iron particles. MR imaging was able to detect the labeled MSC successfully. Chitosan-SPIO did not show any cytotoxicity up to 200 µg Fe/mL concentration. The labeled stem cells did not exhibit any significant alterations in the surface markers expression or adipo/osteo/chondrogenic differentiation potential when compared to unlabeled control cells. After contralateral injection into rabbit ischemic brain, the iron labeled stem cells were tracked by periodical in vivo MR images. The migration of cells was also confirmed by histological studies. The novel chitosan-SPIO enables to label and track MSC for in vivo MRI without cellular alteration.
Stem cell therapy will improve preventive and therapeutic care of many devastating diseases with the replacement of defective or injured tissues (1). The survival and proof of homing and engraftment of transplanted stem cells in the target tissues or organs is critical for the success of cell therapy (2). Biochemical or immunohistochemical analysis of biopsy or autopsy of tissue may give partial or simple "snap shot" information about the fate of transplanted cells. But a real time fate of transplanted cells may be known by noninvasive magnetic resonance (MR) imaging, provided the cell are labeled with an MR contrast agent before transplantation.
Cellular MR imaging using superparamagnetic iron oxide (SPIO) as contrast agent offers high spatial resolution down to submillimeter range. Iron oxide nanoparticles are usually stabilized by coating with polymeric layer using dextran, carboxydextran, starch, albumin, silicones or polyethylene glycol to overcome the problem of particle aggregation due to large surface-to-volume ratio (3, 4). Resovist is a clinically approved MR contrast agent that is coated with carboxydextran. In our previous study, SPIO nanoparticles were synthesized by coating with chitosan (5, 6). Chitosan is a biodegradable natural polymer which is useful for many biological applications due to its biocompatibility, high charge density, non-toxicity, gelling property and ability to improve dissolution (7). Chitin, a poly (-[1,4]-N-acetyl-D-glucosamine), is the second most abundant natural biopolymer after cellulose, and it is abstracted from the crust of crabs and shrimp. Chitosan can be prepared by N deacetylation of chitin.
The purpose of this study is to analyze the functional capacity of mesenchymal stem cells (MSC) after labeled with chitosan-SPIO compared to clinically approved MR contrast agent, Resovist and to detect the transplanted MSC in the rabbit ischemic brain by in vivo MRI.
As was mentioned in our previous study (5, 6), chitosan coated superparamagnetic iron oxide (Chitosan-SPIO) nanoparticles were synthesized by sonochemical method followed by chitosan coating process. Transmission electron microscopy indicated that Chitosan-SPIO nanoparticles are spherical and crystalline. The physical properties of Chitosan-SPIO are as follows: the mean core iron size is 6 nm, the hydrodynamic size including the chitosan coat is 65 nm. The clinically approved MR contrast agent, carboxydextran-coated SPIO (Resovist; Schering AG, Berlin, Germany) was used for comparison. These values of Resovist (mean core size 4.2 nm, mean hydrodynamic size 62 nm) are similar to those of Chitosan-SPIO (8).
MR phantom studies were conducted using aqueous solution of Resovist and Chitosan-SPIO compounds in 4 mL plastic bottle with iron concentration ranging from 0.03-100 µg Fe/mL. The samples were placed in a plastic box containing distilled water to obtain T2 weighted images (turbo spin echo; TR 2,128 msec, TE 80 msec, FOV 230×230 mm, matrix 700×625, 1-mm thickness, number of excitation 8, resolution 0.32×0.37×1.0 mm) using a 3.0 T MR system (Intera Achieva; Philips, Eindhoven, The Netherlands). The signal intensities of the SPIO samples were determined from a 30-mm2 region of interest. The MR signal intensities of 3 replicates were plotted against the different concentration of SPIO.
Mesenchymal stem cells derived from human bone marrow (#PT-2501, Cambrex Bio Science, Rockland, ME, USA) were grown in DMEM that contain 10% fetal bovine serum, 200 mM L-glutamine, and 1% antibiotic-antimycotic (Invitrogen, Carlsbad, CA, USA) at 37℃ and in 5% CO2 atmosphere. The culture medium was changed every 3-4 days once and the cells were sub-cultured at 80-90% growth confluence.
The polyamine, Poly L-lysine hydrobromide (PLL) of molecular weight 388,100 d (#P1524, Sigma, St. Louis, MO, USA) was used as the transfection agent to facilitate the endocytosis of SPIO nanoparticles into the mesenchymal stem cells. In order to facilitate binding iron particles with transfection agent, PLL at 1.5 µg/mL and SPIO at 100 µg Fe/mL concentrations, both prepared in culture medium were gently shaken for 60 min. The MSC were supplied with culture media containing the SPIO-PLL complex such that one milliliter of medium contain 50 µg Fe/mL SPIO and 750 ng/mL PLL as final concentration to ensure labeling (50 µg Fe/mL SPIO was equivalent to 8.81 µg Fe/cm2 surface area in culture dish). The cell cultures were incubated overnight at 37℃ in a 5% CO2 atmosphere. The cells were treated with 3.1-1,600 µg Fe/mL of SPIO nanoparticles for dose response study, and time-course study was performed at 25 and 100 µg Fe/mL concentrations. All the experiments were conducted with at least three replicates.
Prussian blue staining was performed to localize the iron particles in SPIO labeled MSC or rabbit tissues that received MSC. The iron labeled cells were washed twice with phosphate buffered saline (PBS) to remove excess contrast agent, fixed with 4% glutaraldehyde, again washed and incubated for 30 min with 2% potassium ferrocyanide in 6% hydrochloric acid. After washing 3 times with PBS, the cells were counterstained with nuclear fast red.
The SPIO-labeled cells (1.5×106) were fixed in 3% glutaraldehyde-cocadylate buffer at 4℃ overnight. After an hour incubation in 1% OsO4 the cells were dehydrated in graded dilutions of ethanol, embedded in artificial resin (Epon; Merck, Darmstadt, Germany), and processed for electron microscopy. Thin sections of the cell probes were evaluated unstained to prevent false-positive findings. Electron microscopy (EM 10 CR; Carl Zeiss, Oberkochen, Germany) was performed at 60-80 kV. Unstained thin sections were evaluated for endocytosis of SPIO nanoparticles.
The iron accumulation by labeled cells was assessed by inductively coupled plasma-atomic emission spectroscopy (ICP-AES, JY Ultima 2; Jobin Yvon, Longjumeau, France). SPIO-labeled MSC were digested in 400 µL cocktail of sulphuric acid and hydrogen peroxide (1:2) at 100℃ for 18 hr using oil bath. The colorless digested acid solution was made up to 5 mL and undissolved impurities were removed by filtering through 0.22 µm syringe-filter. A standard curve was plotted using Iron AA standard solution (Aldrich, Milwaukee, WI, USA). The iron content of samples was expressed in pg of Fe per cell.
SPIO labeled cells (5×105) were washed twice with PBS and centrifuged to get cell pellet in Eppendorf tube to obtain T2* weighted image (gradient echo) of cell pellet along with the PBS supernatant using 3.0 T MR system (Intera Achieva; Philips). Images were taken by using TR 30 msec, TE 13.8 msec, FA 15°, FOV 128×128 mm, matrix size 512×512, 1-mm thickness, number of excitation 2 and 0.25×0.25×1.0 mm resolution.
WST-8 Cell Counting Kit-8 (#CK04; Dojindo Laboratories, Kumamoto, Japan) was used to evaluate the viability of SPIO-labeled MSC in three replicates. The human MSC were grown in 96-well plates at 5,000 cells in 200 µL culture medium per well. Cells were labeled with the Iron oxide-PLL complex overnight at 37℃ in a 5% CO2 atmosphere. Unlabeled cells which were kept under identical conditions served as control cells. After overnight incubation, 20 µL of WST-8 solution per well was added to the growing cells and were incubated for an additional 1-4 hr. The absorbance of the formazen product was then measured at a wavelength of 450 nm subtracting absorbance at 600 nm or above according to manufacturer's instructions.
Human MSC at 4-5 passages were labeled overnight with chitosan-SPIO nanoparticles or Resovist at 25 and 100 Fe µg/mL, washed twice in PBS and maintained in culture medium for one week. To verify if the SPIO-labeling process affected the cell-surface expression of typical marker proteins, both unlabeled and SPIO-labeled MSC were incubated with anti-human antibodies. The cells were analyzed using CD44-PE, CD73-PE (Becton Dickinson, Heidelberg, Germany), CD29-FITC, CD105-PE, CD166-PE (Beckman Coulter, Miami, FL, USA) antibodies as positive markers, and CD14-FITC, CD34-PE, CD45-FITC (Beckman Coulter) as negative markers. Mouse isotype antibodies served as respective controls (Becton Dickinson and Beckman Coulter). Ten thousand antibody-labeled cells were acquired and analyzed using a Cytomics FC 500 flow cytometer running CXP software (Beckman Coulter).
For inducing adipogenic differentiation, both unlabeled and SPIO-labeled MSCs were cultured in NH AdipoDiff Medium (Miltenyi Biotec, Bergisch Gladbach, Germany) for 3 weeks in eight-chamber slides (Nunc, Naperville, IL, USA) by changing medium twice a week. The induction of adipogenic differentiation was apparent by intracellular lipid-rich vacuoles that can be seen under a light microscope. Lipid vacuoles of cells were detected by staining with Oil Red O (Sigma). Formalin fixed cells were washed and stained with 0.18% Oil Red O for 5 min and counterstained with hematoxylin to find red colored lipids and blue nuclei.
For inducing osteogenic differentiation, SPIO-labeled MSCs were cultured in NH OsteoDiff Medium (Miltenyi Biotec) using eight-chamber slides (Nunc) for 3 weeks changing media twice a week. The osteogenic differentiation was evaluated by the calcium accumulation that was detected by von Kossa staining with modifications (9). The cells were fixed in 10% formalin, washed and were incubated with 5% silver nitrate (Sigma) under UV light for 15 to 30 min. To develop and fix the signal, 1% pyrogallal and 5% sodium thiosulfate were used, followed by counterstaining with nuclear fast red. Staining gave brownish black color to calcium deposits and red color to nuclei.
For inducing chondrogenic differentiation, 2.5×105 SPIO-labeled cells were centrifuged at 150 g for 5 min in a 15-mL polypropylene tube (Falcon, Rockfalls, NJ, USA) to form a pellet (10) and cultured in complete NH ChondroDiff Medium (Miltenyi Biotec) without disturbing the pellet. The medium was changed 2 times a week for 3 weeks. The pellets were fixed in 10% formalin, embedded in paraffin blocks, and analyzed by Alcian Blue staining. The deparaffinized sections were stained for 30 min with 1% Alcian Blue solution (pH 1.0) to blue colored cartilaginous elements. Cell nuclei were counterstained with nuclear fast red that gives red or pink color.
Ischemic condition in rabbit brain was created by infusion of 150-250 µm polyvinyl alcohol particles (Contour; Boston Scientific, Natick, MA, USA) through a microcatheter (Microferret-18 Zeta; William Cook Europe, Bjaeverskov, Denmark) which was inserted through right femoral artery till occluding the left internal carotid artery. On day 4 of ischemia, by directly inserting 1-mL syringe with 25 G needle through burr hole using Stoelting stereotaxic system (Stoelting, Wood Dale, IL, USA), 106 Chitosan-SPIO-labeled MSCs were injected into the contralateral right hemisphere of rabbit brain. A series of in vivo MR images including T2 weighted (turbo spin echo; TR 2,548.6 msec, TE 80 msec, FOV 80×80 mm, thickness 1.5 mm, matrix 224×205, number of excitation 8), diffusion weighted (TR 4,763 msec, TE 50 msec, FOV 80×80 mm, thickness 1.5 mm, matrix 96×94, number of excitation 3) and susceptibility weighted (TR22.6, TE 32.6, FA 10.0, FOV 100×100 mm, thickness 1.4 mm, matrix 144×144, number of excitation 1) images were taken on 1, 8, 11, 17 days after stem cell transplantation. On day 17 the rabbits were sacrificed, brains were harvested and fixed in 4% paraformaldehyde for histological studies.
MR signal intensities in terms of arbitrary values were obtained from each image of Resovist and Chitosan-SPIO at 0.03-100 µg Fe/mL concentrations using an MR system (Philips) and the intensities for 3 replicates were plotted against the range of SPIO concentrations (Fig. 1). The MR signals dropped gradually from 0.03 to 100 µg Fe/mL concentrations and both compounds followed a similar trend.
To visualize the cells through in vitro MRI, the SPIO-labeled MSC were centrifuged to a pellet in microtubes. Iron labeled cells emitted a dark signal in T2* weighted image but not unlabeled control cells (Fig. 2A). Further Prussian blue staining of SPIO labeled MSC to detect internalization of iron reveals that almost all the cells were labeled efficiently with Chitosan-SPIO as well as with Resovist at 50 µg Fe/mL concentrations (Fig. 2B). Endocytosis of SPIO particles was also clearly evident through electron microscopic studies (Fig. 2C). Dark colored SPIO crystals were located in cytoplasmic vacuoles at 8,000-10,000×magnification. The iron loaded cells did not show any abnormal structural changes. ICP-AES data for iron estimation reveals that similar amounts of iron (approximately 18 pg per cell) was taken up by MSC when labeled with Chitosan-SPIO or Resovist iron particles (Table 1).
Dose response of SPIO nanoparticles ranging 3.1-1,600 µg Fe/mL concentrations on cell viability was studied using Resovist and Chitosan-SPIO (Fig. 3A). Resovist and Chitosan-SPIO compounds appear to be safer and did not show any cytotoxicity below 200 µg Fe/mL concentration. However the concentrations of SPIO compounds used in this study were 100 µg Fe/mL or less. Cell viability of iron labeled cells was also monitored until 3 weeks in a time course study after labeling the cells with 25 and 100 µg Fe/mL of SPIO and did not find any significant differences in proliferation compared to control cells (Fig. 3B). FACS immunophenotypic analysis reveals that positive cell surface markers, CD29, CD44, CD73, CD105, and CD166 or negative markers, CD14, CD34, and CD45 of MSC were not altered due to iron labeling with Chitosan-SPIO or Resovist (Fig. 4, Table 2). But a little increase in CD34 marker expression has been observed due to labeling cells with 100 µg Fe/mL of Chitosan-SPIO.
To verify if the iron labeling affected the differentiation capacity, adipogenic, osteogenic and chondrogenic differentiation was induced with iron labeled MSC. MSC labeled with 100 µg Fe/mL of SPIO underwent adipogenic differentiation as efficiently as unlabeled cells that apparent from red colored lipid vacuoles following Oil red O staining (Fig. 5A, B). Also, iron labeling did not affect the osteogenic differentiation potential of MSC (Fig. 5C, D). There were no significant differences found between Resovist and Chitosan-SPIO compounds pertaining to adipogenic or osteogenic differentiation capacity. But chondrogenesis was greatly inhibited by Resovist but not by Chitosan-SPIO, which is evident from Alcian blue staining (Fig. 5E, F). Iron particles were detected by Prussian blue staining in chondro-induced masses 3 weeks after labeling.
Brain ischemic condition in rabbit was generated by infusion of polyvinyl alcohol particles into internal carotid artery at one side. At day 4, MSC that are labeled with 50 µg Fe/mL chitosan-SPIO were injected into contralateral position of brain and the ischemic site as well as transplanted stem cells were visualized by T2WI (Fig. 6A) and diffusion weight image (DWI) (Fig. 6B) images. At day 16, T2WI (Fig. 6C) and susceptibility weighted image (SWI) (Fig. 6D) were used to visualize the stem cell fate. Many of the transplanted stem cells were located at the injected site but some of them migrated to the ischemic site which is more evident from SWI (Fig. 6D). The size of ischemic area was decreased when compared to that of day 4 (Fig. 6A-D). To verify the MRI results brain slices were stained with Prussian blue stain and found SPIO labeled stem cells in the tissue (Fig. 6E, F).
The results of this study demonstrated that the chitosan-SPIO labeling does not alter the proliferation, surface marker expression or differentiation capacity of MSC and the labeled cells can be visualized in the post-ischemic rabbit brain by MRI. Resovist labeling also did not alter functional capacity of MSC except chondrogenesis. The core size of SPIO particles coated with chitosan is approximately 10 nm but only 4.2 in carboxydextran coated Resovist.
A gradual concentration dependent signal-drop was found in MR phantom study that is consistent with previous report (11). Poly-L-Lysine mediated incorporation of chitosan-SPIO into endosomes of cell is a simple but efficient method that enables almost 100% of the cells to be labeled. Efficient labeling of various kinds of cells was also performed by several others using various kinds of transfection agents (12-14). Iron labeling efficiency was evaluated by in vitro cellular MRI, Prussian blue staining, electron microscopy and iron estimation. There is no significant difference found in labeling efficiency with chitosan-SPIO and Resovist. As was reported by others with some iron particles (13, 15), chitosan-SPIO or Resovist also did not affect the cell viability, proliferation, surface marker expression and adipogenic and osteogenic differentiation potential. All the experimental SPIO concentrations were maintained below 100 µg Fe/mL, since chitosan-SPIO and Resovist appear cytotoxic beyond 200 µg Fe/mL concentrations. Chitosan-SPIO labeling did not affect the chondrogenic potential of MSC, however Resovist did affect. Few reports of recent past made the chondrogenesis of feridex labeled MSC described that inhibition of chondrogenesis by feridex labeling could be corrected by using protamine sulfate as transfection agent instead of PLL (13, 15-17). In this study chondrogenesis was not inhibited by chitosan-SPIO but inhibited by Resovist when PLL used as transfection agent. Further studies on the exact molecular mechanism of chondrogenesis in SPIO labeled MSC are warranted. PLL seems not to interfere with chondrogenesis of MSC (15). Although Arbab et al. (17) attributed the inhibition of chondrogenesis to PLL they did not substantiate well how PLL can prevent chondrogenesis.
MR images (T2W, DW, and SW) of rabbit brain before and after transplantation of cells are able to detect brighter ischemic area and darker chitosan-SPIO labeled MSC. Prussian blue staining confirmed the existence of labeled stem cells in the brain tissue. Previous studies were able to trace the transfused CD34-positive cells (18), mononuclear cells (19) and cord blood cells (20) following brain ischemia. This study shows that chitosan-SPIO labeling does not interfere with cell functions and in vivo MRI is able to trace iron labeled cells following transplantation.
For in vivo MR imaging chitosan-SPIO labeling of MSC using PLL as transfection agent did not have any toxic effects on viability or proliferation up to 3 weeks. The iron labeling did not alter the cell surface markers or differentiation capacity including chondrogenesis of MSC when compared to clinically approved Resovist. In the context of serious debate on the effect of SPIO labeling on chondrogenesis (16), chitosan-SPIO labeled MSC did not alter the chondrogenic differentiation capacity of MSC. Further in vivo MR imaging by T2WI, DWI, and SWI enabled to track the chitosan-SPIO labeled MSC in brain ischemic rabbit model up to 2 weeks. Therefore chitosan-SPIO may potentially become a better MR contrast agent especially to track the transplanted stem cells and other cells without compromising cell functional quality.
Figures and Tables
Fig. 1
Comparative MR phantom study of Resovist and chitosan coated SPIO showing similar relation between signal intensity and iron concentration on T2 weighted image.
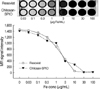
Fig. 2
In vitro evaluation of iron labeling of mesenchymal stem cells by cellular MRI that show labeled cells indicated by arrows (A), Prussian blue staining of in vitro cultured cells (B) and electron microscopic images that show internalized SPIO nanoparticles (C).
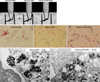
Fig. 3
Cytotoxicity assay of SPIO labeled mesenchymal stem cells. (A), A comparative dose response along with control cells (0), in cells treated overnight with PLL only (PL), SPIO only (IO) and 3.1-1,600 µg/mL SPIO (Resovist or chitosan-SPIO) plus PLL. (B), Time course study on day 4, 7, 14, and 21 after labeling MSC with 25 and 100 µg Fe/mL of Resovist (R25, R100) and chitosan-SPIO (C25, C100) including control (C) and, SPIO only (Fe-only) cells.
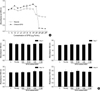
Fig. 4
Phenotypic characterization of SPIO labeled mesenchymal stem cells. Chitosan-SPIO (100 µg Fe/mL) labeled MSC were analyzed for both positive (CD29, CD44, CD73, CD105, CD166) and negative (CD14, CD34, CD45) surface markers of MSC. A little increase in CD34 marker expression is observed (21.3%).
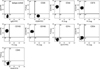
Fig. 5
Differentiation potential of Resovist and chitosan-SPIO labeled mesenchymal stem cells. Iron (100 µg Fe/mL) labeled MSC were exposed to adipogenic (A, B), osteogenic (C, D) and chondrogenic differentiation media (E, F) for 3 weeks. Red colored oil granules are detected by Oil red O staining in adipogenic cultures of Resovist (A) and chitosan-SPIO labeled mesenchymal stem cells (B). Calcium mineralization is detected by von Kossa staining in osteogenic cultures of MSC labeled with Resovist (C) or chitosan-SPIO (D). Alcian Blue staining reveals that chitosan-SPIO labeled MSC (F) exhibit chondrogenic differentiation (asterisk, blue color), but not Resovist labeled cells (E).
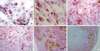
Fig. 6
In vivo MRI of rabbit ischemic brain that received chitosan-SPIO labeled mesenchymal stem cells. Asterisk indicates ischemic area and open arrows indicate chitosan-SPIO-labeled mesenchymal stem cells at the injection site. (A, B) T2WI and diffusion weighted image (DWI) of brain immediately after injection of mesenchymal stem cell at contralateral side of ischemic area (open arrows) on day 4 of ischemia. (C, D) T2WI and susceptibility weighted image (SWI) 16 days after stem cell transplantation. At the ischemic site, dark signal (white arrows) especially on SWI matches mesenchymal stem cells (black arrows) on Prussian staining (E, F). (E, F) Prussian blue staining detected iron labeled stem cells at the liquefied infarct area in a section of 4% paraformaldehyde-fixed brain tissue on day 16.
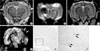
Table 1
Iron content in mesenchymal stem cells one day after 50 µg/mL iron labeling (pg/cell±SD). All data are expressed as mean±SD of at least 5 samples
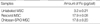
Notes
This research was supported by the Chung-Ang University Research Grants in 2009, and by a grant (07102KFDA430) from Korea Food & Drug Administration in 2007, and was financially supported by the Ministry of Knowledge Economy (MKE) and Korea Industrial Technology Foundation (KOTEF) through the Human Resource Training Project for Strategic Technology.
References
1. Deans RJ, Moseley AB. Mesenchymal stem cells: biology and potential clinical uses. Exp Hematol. 2000. 28:875–884.
3. Berry CC, Wells S, Charles S, Curtis AS. Dextran and albumin derivatised iron oxide nanoparticles: influence on fibroblasts in vitro. Biomaterials. 2003. 24:4551–4557.


4. Harris LA, Goff JD, Carmichael AY, Riffle JS, Harburn JJ, St Pierre TG, Saunders M. Magnetic nanoparticle dispersions stabilized with triblock copolymers. Chem Mater. 2003. 15:1367–1377.
5. Kim EH, Lee HS, Kwak BK, Kim BK. Synthesis of ferrofluid with magnetic nanoparticles by sonochemical method for MRI contrast agent. J Magn Magn Mater. 2005. 289:328–330.
6. Lee HS, Shao H, Huang Y, Kwak BK. Synthesis of MRI contrast agent by coating superparamagnetic iron oxide with chitosan. IEEE Trans Magn. 2005. 41:4102–4104.
7. Sinha VR, Singla AK, Wadhawan S, Kaushik R, Kumaria R, Bansal K, Dhawan S. Chitosan microspheres as a potential carrier for drugs. Int J Pharm. 2004. 274:1–33.


8. Wang YX, Hussain SM, Krestin GP. Superparamagnetic iron oxide contrast agents: physicochemical characteristics and applications in MR imaging. Eur Radiol. 2001. 11:2319–2331.


9. Cheng SL, Yang JW, Rifas L, Zhang SF, Avioli LV. Differentiation of human bone marrow osteogenic stromal cells in vitro: induction of the osteoblast phenotype by dexamethasone. Endocrinology. 1994. 134:277–286.


10. Mackay AM, Beck SC, Murphy JM, Barry FP, Chichester CO, Pittenger MF. Chondrogenic differentiation of cultured human mesenchymal stem cells from marrow. Tissue Eng. 1998. 4:415–428.


11. Bowen CV, Zhang X, Saab G, Gareau PJ, Rutt BK. Application of the static dephasing regime theory to superparamagnetic iron-oxide loaded cells. Magn Reson Med. 2002. 48:52–61.


12. Arbab AS, Bashaw LA, Miller BR, Jordan EK, Lewis BK, Kalish H, Frank JA. Characterization of biophysical and metabolic properties of cells labeled with superparamagnetic iron oxide nanoparticles and transfection agent for cellular MR imaging. Radiology. 2003. 229:838–846.


13. Arbab AS, Yocum GT, Kalish H, Jordan EK, Anderson SA, Khakoo AY, Read EJ, Frank JA. Efficient magnetic cell labeling with protamine sulfate complexed to ferumoxides for cellular MRI. Blood. 2004. 104:1217–1223.


14. Ittrich H, Lange C, Tögel F, Zander AR, Dahnke H, Westenfelder C, Adam G, Nolte-Ernsting C. In vivo magnetic resonance imaging of iron oxide labeled arterially injected mesenchymal stem cells in kidney of rats with acute ischemic kidney injury: detection and monitoring at 3T. J Magn Reson Imaging. 2007. 25:1179–1191.
15. Kostura L, Kraitchman DL, Mackay AM, Pittenger MF, Bulte JW. Feridex labeling of mesenchymal stem cells inhibits chondrogenesis but not adipogenesis or osteogenesis. NMR Biomed. 2004. 17:513–517.


16. Bulte JW, Kratchman DL, Mackay AM, Pittenger MF. Chondrogenic differentiation of mesenchymal stem cells is inhibited after magnetic labeling with ferumoxides. Blood. 2004. 104:3410–3413.


17. Arbab AS, Yocum GT, Rad AM, Khakoo AY, Fellowes V, Read EJ, Frank JA. Labeling of cells with ferumoxides. Protamine sulfate complexes does not inhibit function or differentiation capacity of hematopoitic or mesenchymal stem cells. NMR Biomed. 2005. 18:553–559.
18. Taguchi A, Soma T, Tanaka H, Kanda T, Nishimura H, Yoshikawa H, Tsukamoto Y, Iso H, Fujimori Y, Stern DM, Naritomi H, Matsuyama T. Administration of CD34+ cells after stroke enhances neurogenesis via angiogenesis in a mouse model. J Clin Invest. 2004. 114:330–338.