Abstract
Corticotrophin-releasing factor (CRF), a key regulator of the hypothalamic-pituitary axis, is expressed in the central nucleus of the amygdala (CeA) and its expression is upregulated in stress-related disorders. We investigated here the effect of noxious colorectal distension (CRD) on the expression of CRF in the CeA of conscious and unconscious rats. Adult male rats with or without general anesthesia were exposed to visceral pain induced by CRD for 5 min; this procedure was repeated 3 times with 1 min resting after each distension. The rats were sacrificed and sections of the CeA were immunostained for CRF as an indicator for anxiety response, and for phosphorylated extracellular signal-regulated kinase (p-ERK) as a marker for pain-specific activation of neurons; sections of lumbosacral spinal cord were immunostained for c-Fos as a marker for activation of spinal neurons. CRD elicited a significant increase in the expression of CRF and p-ERK in the CeA and of c-Fos in the spinal cord. General anesthesia attenuated the increase in CRF and p-ERK in the CeA, but did not affect the expression of spinal c-Fos. These results suggest that conscious recognition of pain at higher brain centers is an important determinant of CRF expression in the CeA.
Corticotrophin-releasing factor (CRF) is not only a stress hormone but also acts as a neuromodulator outside the hypothalamic-pituitary-adrenocortical (HPA) axis, playing an important role in anxiety, depression, and pain modulation (1). Previous human studies have also found elevated levels of CRF in the cerebrospinal fluid of patients with depression (2, 3) or posttraumatic stress disorder (PTSD) (4-6).
There is accumulating evidence that the central pain circuits may be anatomically related to those that control depression and anxiety (7). Thus, a major site of extra-hypothalamic expression of CRF and its receptors is the CeA, which not only mediates many aspects of anxiety and fear (8), but is also called the nociceptive amygdala for being an important site for central processing of nociceptive information (9).
Colorectal distension (CRD) is commonly used to model visceral pain in experimental animals and induces significant up-regulation of the immediate early gene product c-Fos, as well as the phosphorylated extracellular signal-regulated kinase (p-ERK) in the spinal cord, brainstem, limbic areas, and neocortex in conscious rats (10-13). Previous studies have indicated that CRF and its receptors in the brain play a critical role in CRD-elicited anxiogenic responses and visceral sensitivity in conscious rats (14-17). Indeed, intracerebral administration of CRF stimulated anxiety-like behavior and hypersensitivity to CRD (17) and a CRF receptor 1 antagonist attenuated the CRD-induced visceral pain and anxiety in rats (16).
However, few studies have investigated the differences in visceral pain-induced CRF expression in the CeA between conscious and unconscious rats (18, 19). Since the CeA integrates descending cortical control with ascending nociceptive input from the parabrachial nucleus, and the conscious recognition of pain attributes the behavioral or emotional response to pain (20), we wanted to ascertain whether the suppression of cortical activation during noxious visceral stimulation affects the expression of CRF in the CeA. For this, we studied the expression of CRF, together with that of p-ERK as a marker for pain-specific activation of neurons in the CeA, and of c-Fos as a marker for activation of spinal neurons.
All experiments were performed on adult male Sprague-Dawley rats (280-300 g; Orient Bio Inc., Gyeonggi-do, Korea) ORIENT bio, Korea) according to the protocol 2008-08 approved by the Institutional Animal Investigation Committee of Hanyang University. Rats were divided into a colorectal distention group (CRD, n=6), a colorectal distention with general anesthesia group (CRD+Anesthesia, n=6), and a control group with balloon insertion without distention (Con, n=6). All animals were handled for 7 days prior to the experiment to minimize stress induced by manipulation.
For CRD, a 6-7 cm-long polyethylene catheter with a latex balloon at the end was inserted through the anus into the descending colon and rectum and fastened to the tail with surgical tape (21). The length of the balloon was 4 cm and a total length of 5 cm (with catheter) was inserted into the anus and placed in the descending colon and the rectum. After 30 min, distension was produced by inflating the balloon to 80 mmHg three times for 5-min with a 1-min inter-trial interval. Rats were allowed to survive for 30 min after CRD to study the expression of p-ERK and c-Fos or for 3 days to study the expression of CRF.
For tissue fixation, rats were deeply anesthetized with a mixture of tiletamine+zolazepam (27.78 mg/kg, intraperitoneal [i.p.]) and xylazine (0.647 mg/kg, i.p.) and perfused intracardially with 100 mL of normal saline, followed by 600 mL of 4% paraformaldehyde in 0.1 M phosphate buffer (pH 7.2). The brain and spinal cord at the level of L6-S1 were removed and postfixed for 4 hr in the same fixative and then transferred to a 30% sucrose solution for a minimum of 18 hr. Sections of amygdala and spinal cord were cut on a cryostat at 40 and 30 µm, respectively, and processed for immunofluorescence. Sections were blocked with 10% normal donkey serum in 0.01 M phosphate buffered saline (PBS, pH 7.4) for 40 min at room temperature and incubated overnight at 4℃ with rabbit anti-CRF (1:1,000; Phoenix Pharmaceuticals, Inc., Belmont, CA, USA), rabbit anti-phospho-p44/42MAP Kinase (1:100; Cell signaling, Beverly, MA, USA) or goat anti-c-Fos (1:500; Serotec, Raleigh, NC, USA) antibodies in PBS. Sections were then incubated with Cy3-conjugated donkey antibodies (1:200 in PBS; Jackson Immunolab, West Grove, PA, USA) for 3 hr, rinsed, mounted, and coverslipped with Vectashield (Vector Laboratories Inc., Burlingame, CA, USA).
For quantitative assessment of immunostaining (22), the CRF-positive area in the CeA was measured with image analysis software (Analysis Pro.V3.1 SIS Münster, Germany). The immunofluorescence images of the bilateral CeA in each of 5 sections per rat 400 µm apart between coronal levels -0.80 mm and -2.80 mm (Paxinos) were acquired by a cooled CCD camera attached to the microscope under a constant exposure and digital gain, and a frame size of 1,760×1,321 µm2. In each image, the CRF-positive area was segmented by setting a value for a given threshold, and the number of segmented pixels was counted. p-ERK-positive neurons were counted in 12 images of the bilateral CeA from 6 sections per rat 400 µm apart (magnification 200×, frame size=439×330 µm2). c-Fos positive nuclei were counted in 12 images of the bilateral dorsal horn from 6 sections per rat 300 µm apart (magnification 100×, frame size=898×660 µm2).
All results were analyzed with SPSS 17.0 (SPSS, Chicago, IL, USA). Differences between groups were analyzed by one-way analysis of variance (ANOVA), followed by a Tukey posttest in which all groups were compared for CRF expression and number of p-ERK and c-Fos positive-neurons. Significance was set at P<0.05.
An immunoreaction for CRF was observed in the neuronal perikarya and neuropil throughout the rostrocaudal extent of the CeA. After CRD, the CRF-immunopositive area had increased significantly in both conscious (72.75±3.48 µm2) and unconscious (23.10±5.24 µm2) rats when compared to the control animals. However, the CRF-positive area in the rats with CRD under general anesthesia was significantly lower than that of the conscious animals (Fig. 1).
p-ERK was expressed in neurons in the CeA. After CRD, the number of pERK-positive neurons significantly increased compared to control animals. In parallel with the changes in CRF expression, the number of pERK-positive neurons was significantly reduced in anesthetized (95.17±22.47 µm2) vs conscious (264.50±65.98 µm2) rats with CRD (Fig. 2).
The number of c-Fos positive nuclei in the dorsal horn was significantly increased by CRD over the control animals (308.0±15.95 µm2), but the difference in expression between anesthetized (567.67±129.35 µm2) and conscious (505.67±50.70 µm2) rats was insignificant (Fig. 3).
The main result of this study is that the expression of CRF and pERK in the CeA was significantly increased by CRD-induced visceral pain, and that this effect was attenuated by general anesthesia, whereas the expression of c-Fos in the spinal cord was unaffected by general anesthesia.
Few studies have investigated the differences between conscious and unconscious animals in regional brain activity induced by visceral pain. Functional magnetic resonance imaging in anesthetized rats revealed that the amygdala, thalamus, cerebellum, and hippocampus were significantly activated during CRD-induced visceral pain (19). Similarly, CRD elicited a significant increase in regional blood flow in the cerebral cortex and amygdala in conscious rats by the autoradiographic cerebral perfusion method (23). The greatest increase in evoked cortical activity induced by somatic pain was observed in the contralateral somatosensory cortex and that was significantly lower in anesthetized rats than in conscious rats (18). Our results demonstrate that the level of consciousness may affect the expression of CRF and pERK in the CeA induced by visceral pain.
Polymodal sensory information of thalamic and cortical origin is related to the CeA through connections with the lateral and basolateral amygdaloid nuclei (24, 25). The amygdala also receives nociceptive information via the spinoparabrachial and spinohypothalamic tracts (20). We speculate that the decreased CRF expression in the CeA of anesthetized rats is related to the reduction of cortical activity caused by general anesthesia, which disrupts the relay of pain-related information from the neocortex to the amygdala.
In our results, CRD caused immediate neuronal activation in the CeA as indicated by the increased expression of p-ERK. Activation of ERK and the related signaling pathways in the cerebral cortex and the amygdala plays an important role in the enhancement of memory-related neuronal activity (26) and in the development of anxiety (27, 28).
Our results also indicate a sustained increased expression of CRF in the amygdala at 72 hr after CRD. Previous animal studies have suggested that exposures to psychological and physiological stresses immediately increase CRF expression in the CeA (29, 30). However, to our knowledge, there has been no study examining prolonged effect of stresses on amygdala CRF system in the rat. Since PTSD and major depression occur frequently following traumatic events, and there is usually a temporal gap between traumatic events and their related symptoms later in life, to understand the prolonged effect of stress at a specific period on the neuroendocrine system and its related symptoms is clinically important.
In conclusion, suppression of cortical activation by general anesthesia during visceral pain attenuated the expression of CRF in the CeA, suggesting that the conscious recognition of pain at a cortical level is an important determinant of the involvement of CRF in the mechanisms of subcortical pain control.
Figures and Tables
Fig. 1
Immunostaining for CRF in the central nucleus of the amygdala (CeA) in rats of the control group (A, CON), the colorectal distension group (B, CRD), and the CRD under general anesthesia group (C, Anesthesia+CRD). The immunostaining for CRF was higher in rats with CRD than in controls three days (72 hr) after CRD. However, the CRF-positive area in rats with CRD under general anesthesia was significantly lower than that of conscious animals (D, area [mean±SEM] in the 5 brain sections 400 µm apart, *P<0.005 vs CON, †P<0.005 vs CRD, one-way ANOVA). Scale bar: 200 µm.
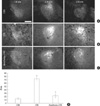
Fig. 2
Immunostaining for phospho-ERK (pERK) in the central nucleus of the amygdala (CeA) in rats of the control group (A, CON), the colorectal distension group (B, CRD), and the CRD under general anesthesia group (C, Anesthesia+CRD). The number of pERK-positive neurons was higher in rats with CRD than in controls 30 min after CRD. The increase of pERK-positive neurons was significantly lower in rats with general anesthesia (D, number of neurons [mean±SEM] in the 5 brain sections 400 µm apart, *P<0.005 vs CON, †P<0.005 vs CRD, one-way ANOVA). Scale bar: A-C, 50 µm.
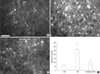
Fig. 3
Immunostaining for c-Fos in the spinal dorsal horn in rats of the control group (A, CON), the colorectal distension group (B, CRD), and the CRD under general anesthesia group (C, Anesthesia+CRD). 30 min after CRD, the number of c-Fos-positive nuclei was higher in rats with CRD than in controls. The difference in expression of c-Fos between conscious and anesthetized rats was not significant (D, number of nuclei [mean±SEM] in the 5 brain sections 300 µm apart, *P<0.05 vs CON, one-way ANOVA). Scale bar: A-C, 100 µm.
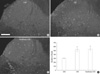
ACKNOWLEDGMENTS
We thank Dr. Juli Valtschanoff of the University of North Carolina for his careful reading and invaluable suggestions for this manuscript.
References
1. Ji G, Neugebauer V. Pro- and anti-nociceptive effects of corticotropin-releasing factor (CRF) in central amygdala neurons are mediated through different receptors. J Neurophysiol. 2008. 99:1201–1212.


2. Nemeroff CB. Psychopharmacology of affective disorders in the 21st century. Biol Psychiatry. 1998. 44:517–525.
3. Nemeroff CB, Widerlov E, Bissette G, Walleus H, Karlsson I, Eklund K, Kilts CD, Loosen PT, Vale W. Elevated concentrations of CSF corticotropin-releasing factor-like immunoreactivity in depressed patients. Science. 1984. 226:1342–1344.


4. Baker DG, West SA, Nicholson WE, Ekhator NN, Kasckow JW, Hill KK, Bruce AB, Orth DN, Geracioti TD Jr. Serial CSF corticotropin-releasing hormone levels and adrenocortical activity in combat veterans with posttraumatic stress disorder. Am J Psychiatry. 1999. 156:585–588.
5. Bremner JD, Licinio J, Darnell A, Krystal JH, Owens MJ, Southwick SM, Nemeroff CB, Charney DS. Elevated CSF corticotropin-releasing factor concentrations in posttraumatic stress disorder. Am J Psychiatry. 1997. 154:624–629.
6. Sautter FJ, Bissette G, Wiley J, Manguno-Mire G, Schoenbachler B, Myers L, Johnson JE, Cerbone A, Malaspina D. Corticotropin-releasing factor in posttraumatic stress disorder (PTSD) with secondary psychotic symptoms, nonpsychotic PTSD, and healthy control subjects. Biol Psychiatry. 2003. 54:1382–1388.


7. Golianu B, Bhandari R, Shaw JR, Borse GW, Gaeta R, Spiegel D. Yudofsky CS, Hales ER, editors. Neuropsychiatric aspects of pain management. The American psychiatric publishing textbook of neuropsychiatry and behavioral neurosciences. 2008. 5th ed. Arlington, VA: American Psychiatric Publishing;368–369.
9. Neugebauer V, Li W, Bird GC, Han JS. The amygdala and persistent pain. Neuroscientist. 2004. 10:221–234.


10. Traub RJ, Silva E, Gebhart GF, Solodkin A. Noxious colorectal distention induced-c-Fos protein in limbic brain structures in the rat. Neurosci Lett. 1996. 215:165–168.


11. Wang L, Martinez V, Larauche M, Tache Y. Proximal colon distension induces Fos expression in oxytocin-, vasopressin-, CRF- and catecholamines-containing neurons in rat brain. Brain Res. 2009. 1247:79–91.


12. Zhang XJ, Li Z, Chung EK, Zhang HQ, Xu HX, Sung JJ, Bian ZX. Activation of extracellular signal-regulated protein kinase is associated with colorectal distension-induced spinal and supraspinal neuronal response and neonatal maternal separation-induced visceral hyperalgesia in rats. J Mol Neurosci. 2009. 37:274–287.


13. Martinez V, Wang L, Tache Y. Proximal colon distension induces Fos expression in the brain and inhibits gastric emptying through capsaicin-sensitive pathways in conscious rats. Brain Res. 2006. 1086:168–180.
14. Saito-Nakaya K, Hasegawa R, Nagura Y, Ito H, Fukudo S. Corticotropin-releasing hormone receptor 1 antagonist blocks colonic hypersensitivity induced by a combination of inflammation and repetitive colorectal distension. Neurogastroenterol Motil. 2008. 20:1147–1156.


15. Trimble N, Johnson AC, Foster A, Greenwood-van Meerveld B. Corticotropin-releasing factor receptor 1-deficient mice show decreased anxiety and colonic sensitivity. Neurogastroenterol Motil. 2007. 19:754–760.


16. Saito K, Kasai T, Nagura Y, Ito H, Kanazawa M, Fukudo S. Corticotropin-releasing hormone receptor 1 antagonist blocks brain-gut activation induced by colonic distention in rats. Gastroenterology. 2005. 129:1533–1543.


17. Greenwood-Van Meerveld B, Johnson AC, Cochrane S, Schulkin J, Myers DA. Corticotropin-releasing factor 1 receptor-mediated mechanisms inhibit colonic hypersensitivity in rats. Neurogastroenterol Motil. 2005. 17:415–422.


18. Lahti KM, Ferris CF, Li F, Sotak CH, King JA. Comparison of evoked cortical activity in conscious and propofol-anesthetized rats using functional MRI. Magn Reson Med. 1999. 41:412–416.


19. Lazovic J, Wrzos HF, Yang QX, Collins CM, Smith MB, Norgren R, Matyas K, Ouyang A. Regional activation in the rat brain during visceral stimulation detected by c-fos expression and fMRI. Neurogastroenterol Motil. 2005. 17:548–556.


20. Almeida TF, Roizenblatt S, Tufik S. Afferent pain pathways: a neuroanatomical review. Brain Res. 2004. 1000:40–56.


21. Ness TJ, Gebhart GF. Colorectal distension as a noxious visceral stimulus: physiologic and pharmacologic characterization of pseudaffective reflexes in the rat. Brain Res. 1988. 450:153–169.


22. Eitner F, Bucher E, van Roeyen C, Kunter U, Rong S, Seikrit C, Villa L, Boor P, Fredriksson L, Backstrom G, Eriksson U, Ostman A, Floege J, Ostendorf T. PDGF-C is a proinflammatory cytokine that mediates renal interstitial fibrosis. J Am Soc Nephrol. 2008. 19:281–289.


23. Wang Z, Bradesi S, Maarek JM, Lee K, Winchester WJ, Mayer EA, Holschneider DP. Regional brain activation in conscious, nonrestrained rats in response to noxious visceral stimulation. Pain. 2008. 138:233–243.


24. Pitkanen A, Savander V, LeDoux JE. Organization of intra-amygdaloid circuitries in the rat: an emerging framework for understanding functions of the amygdala. Trends Neurosci. 1997. 20:517–523.
26. Cohen-Matsliah SI, Brosh I, Rosenblum K, Barkai E. A novel role for extracellular signal-regulated kinase in maintaining long-term memory-relevant excitability changes. J Neurosci. 2007. 27:12584–12589.


27. Di Benedetto B, Kallnik M, Weisenhorn DM, Falls WA, Wurst W, Holter SM. Activation of ERK/MAPK in the lateral amygdala of the mouse is required for acquisition of a fear-potentiated startle response. Neuropsychopharmacology. 2009. 34:356–366.


28. Schafe GE, Atkins CM, Swank MW, Bauer EP, Sweatt JD, LeDoux JE. Activation of ERK/MAP kinase in the amygdala is required for memory consolidation of pavlovian fear conditioning. J Neurosci. 2000. 20:8177–8187.


29. Merlo Pich E, Lorang M, Yeganeh M, Rodriguez de Fonseca F, Raber J, Koob GF, Weiss F. Increase of extracellular corticotropin-releasing factor-like immunoreactivity levels in the amygdala of awake rats during restraint stress and ethanol withdrawal as measured by microdialysis. J Neurosci. 1995. 15:5439–5447.