Abstract
Activated protein C (APC) is known to be beneficial on ischemia reperfusion injury in myocardium. However, the protection mechanism of APC is not fully understood. The purpose of this study was to investigate the effects and possible mechanisms of APC on myocardial ischemic damage. Artificially ventilated anaesthetized Sprague-Dawley rats were subjected to a 30 min of left anterior descending coronary artery occlusion followed by 2 hr of reperfusion. Rats were randomly divided into four groups; Sham, I/R, APC preconditioning and postconditioning group. Myocardial infarct size, apoptosis index, the phosphorylation of ERK1/2, Bcl-2, Bax and cytochrome c genes and proteins were assessed. In APC-administrated rat hearts, regardless of the timing of administration, infarct size was consistently reduced compared to ischemia/reperfusion (I/R) rats. APC improved the expression of ERK1/2 and anti-apoptotic protein Bcl-2 which were significantly reduced in the I/R rats. APC reduced the expression of pro-apoptotic genes, Bax and cytochrome c. These findings suggest that APC produces cardioprotective effect by preserving the expression of proteins and genes involved in anti-apoptotic pathways, regardless of the timing of administration.
Myocardial ischemia-reperfusion injury (MIRI) is a complex pathophysiological event, resulting in serious acute and chronic heart damage. Although the precise mechanism responsible for MIRI remains unclear, it is widely accepted that apoptotic cell death is involved in the development of ischemic heart damage. Activated protein C (APC) is an important physiological anticoagulant that is produced from protein C by the action of the thrombin-thrombomodulin complex on endothelial cells (1). APC plays a significant role in the regulation of anti-inflammatory processes by inhibiting cytokine production by monocytes and has been reported to attenuate tissue or organ injury in various pathological conditions (2-5). It has been well demonstrated that APC can reduce hypoxia-induced apoptosis in neuron and cerebral endothelial cells (2). In myocardial ischemia, APC has been reported to have beneficial effects in ischemia-reperfusion injury with its inhibitory effects on the myocardial apoptosis and inflammation (1).
Apoptosis is a crucial event in various physiologic processes, such as embryogenesis, organ development, and cell proliferation, as well as in pathologic processes, which contributes significantly to post-ischemic cardiomyocyte death (6). But there are no evidence regarding the effect of activated protein C on anti-apoptotic pathways in ischemia/reperfusion (I/R) injury. In mammalian cells, among three major mitogen activated protein kinases (MAPKs) signaling pathways, activation of extracellular signal related protein kinases (ERK1/2) exerts beneficial effect on post-ischemic myocardial apoptosis (7). Thus, we evaluated the effects of activated protein C against I/R injury on myocardial infarction in rat in terms of the expression of proteins involved in apoptosis signaling cascades.
Male Sprague-Dawley (SD) rats (SPF grade, 220-250 g) were purchased from Tongji Medical School, Huazhong University of Science and Technology (HUST), China. Animals were maintained under standard laboratory conditions at 25±2℃, relative humidity of 50±15%, and normal photoperiod (12 hr dark and 12 hr light). The procedures for experiments and animal care were approved by the institutional animal care and use committee of HUST (approval number: 2004-0007), and conformed to the guide for the care and use of laboratory animals by the National Institutes of Health (NIH Publication No. 80-23).
APC was purchased from Sigma-Aldrich (Oakvile, ON, Canada), and dissolved in distilled water on the day of administration. The 48 rats were randomly divided into four groups: the sham operation group (sham); I/R group (I/R); APC-preconditioning group (Pre-APC) and APC-postconditioning group (Post-APC). In the sham operation and I/R groups, 0.9 percent saline solution (2 mL/kg) was injected 5 min prior to the reperfusion protocol. In APC-preconditioning group, APC (2 mg/kg) was given also 5 min prior to the reperfusion protocol. In APC-postconditioning group, APC was administrated 5 min after reperfusion.
The rats were anesthetized with an intraperitoneal injection of pentobarbital sodium (30 mg/kg). After endotracheal intubation, ventilation was provided by a rodent respirator at a respiratory rate of 50/min with a tidal volume of 20 mL/kg body weight (8). A left parasternal incision was made through the third and fourth ribs, and the pericardium was gently opened to expose the heart. The lateral anterior descending artery (LAD) was ligated using a 6-0 silk suture. Additionally, a medical latex tube (socket, inner diameter, 1.5 mm) was placed between the ligature and the LAD. Myocardial ischemia was induced by compressing the LAD by tightening the ligature around the latex tube. The ECG was monitored for changes in the ST-T segment caused by tightening or loosing the ligation. After 30-min ischemia, the latex tube was removed to reperfuse the myocardium by restoring the coronary circulation. After 2-hr reperfusion, rats were killed, and parts of the anterior wall of the left ventricular myocardium near the cardiac apex were obtained for further analysis. The sham control group underwent the same procedures, with the exception of the induction of myocardial ischemia/reperfusion.
We assessed cardiac hemodynamic function in vivo. The right common carotid artery of rat was isolated and cannulated with a 2-Fr micromanometer. Left ventricular (LV) pressure and LV end-diastolic pressure (LVEDP) were measured by the catheter advanced into the LV cavity, and data were recorded and analyzed. These parameters as well as LV maximum positive change in pressure over time (+dP/dtmax), as a measure of cardiac contractility, and LV minimum negative change in pressure over time (-dP/dtmin), as a measure of relaxation, were recorded at baseline and after administration of APC.
At the end of the perfusion protocols, the coronary artery was reoccluded and 4 mL of 0.1% Evans blue dye was injected into the aorta to delineate the zone at risk. Stained hearts were frozen, sliced, and incubated at 37℃ in 1% triphenyltetrazolium chloride to delineate the infarcted tissue. Slices were then fixed and quantified by planimetry using the computer-based image analyzer SigmaScan Pro 5.0 (SPSS Science, Chicago, IL, USA). Infarct size was expressed as a percentage of the area at risk zone. The area at risk was calculated as a percentage of the total ventricular area (9).
LV specimens were pulverized and dissolved in lysis buffer. The solution was vigorously homogenized and centrifuged at 12,000 g for 10 min at 4℃. The supernatant was separated in a 12% SDS-polyacrylamide gel and transferred to PVDF membrane. After blocking the membrane with Tris-buffered saline-Tween 20 (TBS-T, 0.1% Tween 20) containing 5% non-fat dried milk for 1 hr at room temperature, membranes were washed twice with TBS-T and incubated with primary antibodies for 1 hr at room temperature or for overnight at 4℃. The following primary antibodies were used: rabbit anti-extracellular signal-regulated kinases (ERK1/2), mouse anti-phospho ERK1/2 and mouse anti-β actin antibodies (Sigma, St. Louis, MO, USA). The membranes were washed three times with TBS-T for 10 min, and then incubated for 1 hr at room temperature with horseradish peroxidase (HRP)-conjugated secondary antibodies. After extensive washing, the bands were visualized by an enhanced chemiluminescence system (10).
The total RNA from the cardiac muscle samples was extracted and purified using the Trizol reagent kit (Invitrogen, CA, USA). Total RNA was reversely transcribed into complementary DNA (cDNA) using the cDNA synthesis kit (Takara Bionic, Otsu, Japan) according to the manufacturer's protocol. The opticon-2 real-time PCR reactor (MJ Research, Fremont, CA, USA) and real-time PCR kit (SYBR Premix Ex TaqTM, TaKaRa, Japan) were employed based on the manufacturer's instruction. A 20 µL reverse transcription reaction mixture was incubated at 42℃ for 15 min, heated to 95℃ for 2 min. The PCR condition was at 95℃ for 10 sec, then 40 cycles of 95℃ for 5 sec, and 60℃ for 30 sec and 72℃ for 1 min. GAPDH and β-actin were used as the housekeeping genes. The mRNA levels were calculated based on the method of 2-ΔΔCt(11,12). The primers were as follows: Bax, sense primer 5'-AGACACCTGAGCTGACCTTGGAG-3', antisense primer 5'-GTTGAAGTTGCCATCAGCAAACA-3'. Bcl-2, sense primer 5'-TGAACCGGCATCTGCACAC-3', antisense primer 5'-CGTCTTCAGAGACAGCCAGGAG-3'. cytochrome c, sense primer 5'-CATAAGACTGGACCAAACC-3', antisense primer 5'-TCTGCCCTTTCTCCCT-3'. β-actin, sense primer 5'-GTCCCTCACCCTCCCAAAAG-3', antisense primer 5'-GCTGCCTCAACACCTCAACCC-3'. GAPDH, sense primer 5'-ACCACAGTCCATGCCATCAC-3' and antisense primer 5'-TCCACCACCCTGTTGCTGTA-3'.
The myocardial tissues were fixed with 10% neutral-buffered formalin, dehydrated in increasing concentrations of ethanol, embedded in paraffin, and sectioned into 4-µm slices. After deparaffinization, the sections were immersed in 3% hydrogen peroxide in methanol for 30 min, placed in a 500-W microwave for 5 min and incubated with normal goat serum for 10 min. The primary antibody was diluted appropriately and incubated with the sections overnight at 4℃. Biotinylated secondary antibody was added followed by the addition of HRP-Streptavidin complex, and then the sections were stained with DAB solution and counterstained with hematoxylin. Brown immunoprecipitates occurred in positive cytoplasm after staining. In immunohistochemical analysis of quantity, five random microscopic fields from each section were examined at ×400 magnification with a micro-image collecting system. The average grey values were analyzed using LEICA Q55OIW image analysis software.
Myocardial tissue samples were obtained at the end of the reperfusion, fixed in 10% paraformaldehyde, paraffin-embedded, and sectioned. TUNEL staining was performed in deparaffinized and rehydrated sections according to the demands of TUNEL assay kit. After TUNEL staining, the sections were counterstained with hematoxylin. Total cardiomyocytes and TUNEL positive cells in the specimens were counted under light microscopic analysis. Five high-power fields (×400) of each section were randomly selected from the marginal and the central parts of the ischemic zone. The ratio of TUNEL-positive cells to total cardiomyocytes was apoptosis index (AI).
Quantitative data were expressed as mean±SD. For experiments with three or more groups of animals, one-way analysis of variance (ANOVA) was performed, and Student-Newman-Keuls (SNK)-qtest was used for selected groups. A P value of less than 0.05 was considered as statistically significant. Analysis was carried out using Statistical Product and Service Solutions (SPSS 11.0).
Student-Newman-Keuls (SNK)-qtest is a kind of method to made multiple comparison.
Statistical Product and Service Solutions (SPSS11.0) is Statistical software.
Parameters were studied both at baseline and in response to APC administration and included LVDP, LVEDP, and LV+dP/dtmax and LV-dP/dtmin as measures of ventricular contractility and relaxation, respectively. Rats undergoing I/R had significantly impaired LV contractile function. Treatment with APC significantly preserved impaired LV contractility and relaxation. Obviously improvements in LV +dP/dtmax and LV -dP/dtmin were also seen in animals treated with APC. No differences existed in LVEDP, LVDP, +dP/dt, and -dP/dt between the APC groups during ischemia and reperfusion (Fig. 1).
Mean infarct size of the AAR in the I/R group was 58.7±3.1%. In the groups administered with APC, infarct sizes were significantly reduced to 31.4±2.6% and 28.4±2.8% in the Pre-APC and Post-APC group, respectively (all P<0.01). No significant differences were observed between the APC-administrated groups (Fig. 2).
Western blot analysis of ERK1/2 is shown in Fig. 3. I/R injury obviously increased P-ERK1/2 expression (3.4±0.21; respectively) compared to the sham as measured densitometrically (fold relative to sham). In contrast to the I/R group, APC treatment significantly increased P-ERK1/2 expression (7.45±0.34 vs 3.4±0.21, P<0.01 and 6.98±0.31 vs 3.4±0.21, P<0.01). Phosphorylations of ERK1/2 were similar between the APC-administrated groups (Fig. 3).
Expression levels of pro-apoptotic proteins, Bax and cytochrome c, significantly increased and expression level of anti-apoptotic protein, Bcl-2 significantly decreased in the I/R group than in the sham group. In the APC-administrated groups, the expression of Bax and cytochrome c significantly decreased and the expression level of Bcl-2 significantly increased than in the I/R group. The expression levels of Bax, cytochrome c and Bcl-2 were similar among the APC-administrated groups (Fig. 4, Table 1).
A number of TUNEL-positive cells were observed in the myocardium in the I/R group. Compared with I/R group, the apoptosis indeces were decreased significantly in APC-administrated groups (all P<0.01). No significant differences were observed between the APC-administrated groups (Fig. 5).
The current results confirm that APC significantly reduces myocardial infarct size without reference to the timing of administration in I/R rat heart, which is in conjunction with the preserved phosphorylations of ERK1/2 and anti-apoptotic proteins, Bcl-2 and attenuated pro-apoptotic proteins, Bax and cytochrome c resulting from I/R.
Activated protein C (APC) is a serine protease with systemic anticoagulant activity, which is mediated by irreversible proteolytic inactivation of factors Va and VIIIa with contributions by various cofactors (13). Independent of its anticoagulant activity, APC exerts direct cellular effects that are mediated by the protein C cellular pathway resulting in the following: 1) cytoprotective alteration of gene expression profiles, 2) antiinflammatory activities, 3) antiapoptotic activity, and 4) protection of endothelial barriers (14-16). In myocardial ischemia, APC has been reported to have beneficial effects in ischemia-reperfusion injury with its inhibitory effects on the myocardial apoptosis and inflammation (1). However, little has been studied regarding mechanisms of APC induced cardioprotection and we could observe that APC acted through proteins involved in anti-apoptoic pathways of survival regardless of timing of administration. Apoptosis has been linked with reperfusion-induced myocardial injury after reversible coronary occlusion and suggested as one of the key mechanisms in the development of infarction in rat cardiac myocytes (17, 18).
In the current study, APC increased the phosphorylation of ERK1/2 and anti-apoptotic proteins in I/R rat heart. ERK is activated in response to I/R, oxidative stress, and hypoxia, and is an established player in the anti-apoptotic defense network (7). As our results indicate, APC preserved the phosphorylation of ERK1/2 which were significantly reduced following I/R leading to improved viability of the rat heart exposed to I/R. In response to an apoptotic stimulus such as I/R, the pro-apoptotic protein, Bax, undergoes a conformational change that allows it to translocate to the mitochondria, where it induces cytochrome c release. Once released, cytochrome c leads to the formation of the apoptosome, a complex comprised of apoptotic protease-activating factor-1 (Apaf-1), procaspase-9 and ATP, which permits the autoactivation of procaspase-9 that in turn facilitates caspase-3 activation. Active caspase-3 activates the caspase activated DNase, leading to oligonucleosomal DNA fragmentation (19). Phosphorylation of ERK1/2 inhibits the conformational change in Bax protein and cytochrome c induced caspase activation, thereby preventing apoptosis (20). Bcl-2 normally resides in mitochondrial membranes and the cytoplasm, whose overexpression is a potential mechanism for apoptotic resistance (21). The anti-apoptotic protein Bcl-2 attenuates cellular injury by inhibiting cytochrome c translocation and inhibits Bax translocation (22, 23). In this study, we could observe that improved phosphorylations of ERK1/2, and associated recovery of expression of Bcl-2 and mitigated expression of Bax and mitochondrial cytochrome c release against I/R induced injury, which all indicate APC induced a cardioprotective effect via anti-apoptosis pathways of survival in I/R rat heart. In addition, we have investigated the cardioprotective effects of APC with different administration protocols, and we observed similar protective effect between APC pre- and post-conditioning groups. These results suggest that cardioprotective signaling pathways during the APC pre- and post-conditioning might be similar.
In conclusion, APC confers myocardial protection against injury in I/R rat hearts without reference to the timing of administration, which is conducted with preserved phosphorylations of ERK1/2 and of signal transduction proteins against apoptotic cell death.
Figures and Tables
Fig. 1
The changes of the hemodynamic parameters before and during myocardial I/R. (A) Left ventricular end-diastolic pressure (LVEDP) during ischemia-reperfusion injury. (B) Left ventricular developed pressure (LVDP). (C) Contractility (+dP/dt) during ischemia-reperfusion injury. dP/dtmin during ischemia-reperfusion injury. (D) Compliance (-dP/dt) during ischemia-reperfusion injury. All values are means±SE (n=6).
*P<0.05 for Pre-APC and Post-APC vs the I/R group.
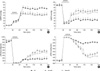
Fig. 2
The effect of APC on infarct size. Infarct size is expressed as a percentage of the area at risk in rats, which was reduced by APC administration. Mean±SE, n=6.
*P<0.01 vs sham group; †P<0.01 vs I/R group.
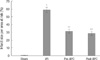
Fig. 3
Effect of APC treatment on p-ERK protein in rat heart after 30 min ischemia and 2 h reperfusion. The phosphorylation was quantified by densitometry using image analysis program. Mean±SE, n=6.
*P<0.01 vs sham; †P<0.01 vs I/R.
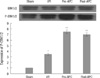
Fig. 4
The effect of APC on expressions of Bax, Bcl-2 and cytochrome C mRNA. All values are means±SE (n=6).
*P<0.01 vs sham group; †P<0.05 vs I/R group.
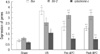
Fig. 5
Effect of APC on apoptosis in ischemic reperfusion-induced myocardial injury. Detection of apoptosis was done at 2 hr after reperfusion. (A) Sham group, (B) I/R group, (C) Pre-APC group, (D) Post-APC group. (E) Mean apoptotic index was counted in each group (A-D). TUNEL stain ×400 (n=6 animals per group). Arrow indicates TUNEL positive cells.
*P<0.05 vs sham group, †P<0.05 vs I/R group.
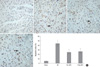
ACKNOWLEDGMENTS
We thank Professors C. Zhang and L. Han of the Faculty of the Microbiology Department, Professor Y. Hu and Dr. B. Gao of the Department of Pathology of the First College of Clinical Medical Sciences, and the China Three Gorges University for their helpful discussions and suggestions on this work.
References
1. Loubele ST, Spek CA, Leenders P, van Oerle R, Aberson HL, Hamulyák K, Ferrell G, Esmon CT, Spronk HM, ten Cate H. Activated protein C protects against myocardial ischemia/reperfusion injury via inhibition of apoptosis and inflammation. Arterioscler Thromb Vasc Biol. 2009. 29:1087–1092.
2. Shibata M, Kumar SR, Amar A, Fernandez JA, Hofman F, Griffin JH, Zlokovic BV. Anti-inflammatory, antithrombotic, and neuroprotective effects of activated protein c in a murine model of focal ischemic stroke. Circulation. 2001. 103:1799–1805.


3. Mizutani A, Okajima K, Uchiba M, Noguchi T. Activated protein C reduces ischemia/reperfusion-induced renal injury in rats by inhibiting leukocyte activation. Blood. 2000. 95:3781–3787.


4. Grey S, Hau H, Salem HH, Hancock WW. Selective effects of protein C on activation of human monocytes by lipopolysaccharide, interferon-gamma, or PMA: modulation of effects on CD11b and CD14 but not CD25 or CD54 induction. Transplant Proc. 1993. 25:2913–2914.
5. Murakami K, Okajima K, Uchiba M, Johno M, Nakagaki T, Okabe H, Takatsuki K. Activated protein C prevents LPS-induced pulmonary vascular injury by inhibiting cytokine production. Am J Physiol. 1997. 272:L197–L202.


6. Gottlieb RA, Engler RL. Apoptosis in myocardial ischemia-reperfusion. Ann N Y Acad Sci. 1999. 874:412–426.


7. Yue TL, Wang C, Gu JL, Ma XL, Kumar S, Lee JC, Feuerstein GZ, Thomas H, Maleeff B, Ohlstein EH. Inhibition of extracellular signal-regulated kinase enhances ischemia/Reoxygenation-induced apoptosis in cultured cardiac myocytes and exaggerates reperfusion injury in isolated perfused heart. Circ Res. 2000. 86:692–699.


8. Maulik N, Engelman RM, Rousou JA. Ischemic preconditioning reduces apoptosis by upregulating anti-death gene Bcl-2. Circulation. 1999. 100:19 Suppl. II369–II375.


9. Song DK, Jang Y, Kim JH, Chun KJ, Lee D, Xu Z. Polyphenol (-)-epigallocatechin gallate during ischemia limits infarct size via mitochondrial KATP channel activation in isolated rat hearts. J Korean Med Sci. 2010. 25:380–386.


10. Yang J, Jiang H, Yang J, Ding JW, Chen LH, Li S, Zhang XD. Valsartan preconditioning protects against myocardial ischemia-reperfusion injury through TLR4/NF-kappaB signaling pathway. Mol Cell Biochem. 2009. 330:39–46.
11. Marino JH, Cook P, Miller KS. Accurate and statistically verified quantification of relative mRNA abundances using SYBR green I and real-time RT-PCR. J Immunol Methods. 2003. 283:291–306.


12. Vandesompele J, De Preter K, Pattyn F, Poppe B, Van RN, De Paepe A, Speleman F. Accurate normalization of real-time quantitative RT-PCR data by geometric averaging of multiple internal control genes. Genome Biol. 2002. 3:research0034. 1–research0034. 11.
13. Mosnier LO, Zlokovic BV, Griffin JH. The cytoprotective protein C pathway. Blood. 2007. 109:3161–3172.


14. Joyce DE, Gelbert L, Ciaccia A, DeHoff B, Grinnell BW. Gene expression profile of antithrombotic protein c defines new mechanisms modulating inflammation and apoptosis. J Biol Chem. 2001. 276:11199–11203.


15. Finigan JH, Dudek SM, Singleton PA, Chiang ET, Jacobson JR, Camp SM, Ye SQ, Garcia JG. Activated protein C mediates novel lung endothelial barrier enhancement: role of sphingosine 1-phosphate receptor transactivation. J Biol Chem. 2005. 280:17286–17293.
16. Pirat B, Muderrisoglu H, Unal MT, Ozdemir H, Yildirir A, Yucel M, Turkoglu S. Recombinant human-activated protein C inhibits cardiomyocyte apoptosis in a rat model of myocardial ischemia-reperfusion. Coron Artery Dis. 2007. 18:61–66.


17. Haunstetter A, Izumo S. Toward antiapoptosis as a new treatment modality. Circ Res. 2000. 86:371–376.


18. Buja LM, Entman ML. Modes of myocardial cell injury and cell death in ischemic heart disease. Circulation. 1998. 98:1355–1357.


19. Yoo KH, Yim HE, Jang GY, Bae IS, Choi BM, Hong YS, Lee JW. Endothelin A receptor blockade influences apoptosis and cellular proliferation in the developing rat kidney. J Korean Med Sci. 2009. 24:138–145.


20. Tsuruta F, Masuyama N, Gotoh Y. The phosphatidylinositol 3-kinase (PI3K)-Akt pathway suppresses Bax translocation to mitochondria. J Biol Chem. 2002. 277:14040–14047.


21. Myong NH. Tissue microarray analysis of fas and fasL expressions in human non-small cell lung carcinomas; with reference to the p53 and bcl-2 overexpressions. J Korean Med Sci. 2005. 20:770–776.


22. Zhu L, Yu Y, Chua BH, Ho YS, Kuo TH. Regulation of sodium-calcium exchange and mitochondrial energetics by Bcl-2 in the heart of transgenic mice. J Mol Cell Cardiol. 2001. 33:2135–2144.


23. Lazou A, Iliodromitis EK, Cieslak D, Voskarides K, Mousikos S, Bofilis E, Kremastinos DT. Ischemic but not mechanical preconditioning attenuates ischemia/reperfusion induced myocardial apoptosis in anaesthetized rabbits: the role of Bcl-2 family proteins and ERK1/2. Apoptosis. 2006. 11:2195–2204.

