Abstract
5-Lipoxygenase inhibitor and human recombinant erythropoietin might accelerate renal recovery in cisplatin-induced acute renal failure rats. Male Sprague-Dawley rats were randomized into four groups: 1) normal controls; 2) Cisplatin group-cisplatin induced acute renal failure (ARF) plus vehicle treatment; 3) Cisplatin+nordihydroguaiaretic acid (NDGA) group-cisplatin induced ARF plus 5-lipoxygenase inhibitor treatment; 4) Cisplatin+erythropoietin (EPO) group-cisplatin induced ARF plus erythropoietin treatment. On day 10 (after 7 daily injections of NDGA or EPO), urea nitrogen and serum Cr concentrations were significantly lower in the Cisplatin+NDGA and Cisplatin+EPO groups than in the Cisplatin group, and 24 hr urine Cr clearances were significantly higher in the Cisplatin+EPO group than in the Cisplatin group. Semi-quantitative assessments of histological lesions did not produce any significant differences between the three treatment groups. Numbers of PCNA(+) cells were significantly higher in Cisplatin, Cisplatin+NDGA, and Cisplatin+EPO groups than in normal controls. Those PCNA(+) cells were significantly increased in Cisplatin+NDGA group. These results suggest that EPO and also NDGA accelerate renal function recovery by stimulating tubular epithelial cell regeneration.
Acute renal failure (ARF) is characterized by a rapid decline in glomerular filtration rate (GFR) over hours to days, and the retentions of nitrogenous waste products. The mortality rate of patients with ARF has remained 25-70% despite the use of various pharmacologic agents (1). Therefore, a new therapeutic agent is required to promote renal function recovery and a noninvasive early biomarker is needed to determine the presence of early ARF.
The anticancer drug cisplatin is one of the most active drugs used to treat tumors (2, 3). Furthermore, cisplatin may induce injury to renal tubular epithelial cells and subsequent renal failure (4).
Bagnis et al. (5) reported that human recombinant erythropoietin (EPO) enhances recovery from cisplatin-induced ARF in the rats, which suggests that EPO acts directly on damaged tubular cells and stimulates their regeneration. EPO is a cytokine that is produced primarily in the kidneys (6), and which regulates the differentiation and proliferation of erythroid progenitor cells. However, its effects may not be limited to bone marrow progenitor cells, because in vitro, human recombinant EPO stimulates endothelial cell proliferation (7) and angiogenesis (8).
The 5-lipoxygenase (LOX) inhibitor, nordihydroguaiaretic acid (NDGA), is a simple lignin and is found in evergreen shrubs, such as the creosote bush (Larrea tridentata or Larrea divarita), which has a worldwide distribution. The creosote bush is used in traditional medicine to treat more than 50 illnesses, such as rheumatism, diabetes, sterility, gallbladder and kidney stones (9-15). Furthermore, LOX inhibitors have been reported to have protective effects on ileal motor disturbances induced by endotoxins (16), to promote adaptation following massive bowel resection (17), and to aid the correction of coronary spasms of immune origin (18).
Most studies reported previously, were designed to administer drugs before or at the same time of renal insult. However, most therapeutic agents are usually administered after the expression of clinical diseases. Therefore we hypothesized that EPO and NDGA might affect the course of tubular repair after the onset of cisplatin-induced ARF, and thus, accelerate renal recovery in the rats.
Cisplatin (Cisplan®, Dong-A Pharm., Seoul, Korea) was provided as a powder dissolved in saline (1 mg/mL). Human recombinant EPO (Epoetin beta; Recormon®, Roche, Switzerland) was provided as a 2,000 IU/mL solution. The 5-LOX inhibitor, NDGA (6-dimethylamino-9 [3' amino-3' deoxyribosyl] purine, Sigma Chemical Co., St. Louis, MO, U.S.A.) was provided as a powder dissolved in dimethyl sulfoxide (DMSO) solution.
Male Sprague-Dawley rats (n=40), initially weighing 200-250 g were kept in individual metabolic cages with free access to standard chow and water. The rats were divided into four groups: 1) the normal control group (n=10); 2) the Cisplatin group (n=10)-Cisplatin induced ARF plus vehicle; 3) the Cisplatin+NDGA group (n=10)-Cisplatin induced ARF plus NDGA; 4) the Cisplatin+EPO group (n=10)-Cisplatin induced ARF plus EPO. Animals were clinically investigated and subjected to standard laboratory tests for one week before being sacrificed for kidney removal. All experiments were performed in accordance with the National Institute's of Health 'Guiding Principles for the Care and Use of Laboratory Animals'.
On day 1, ARF was provoked by a single intraperitoneal (i.p.) injection (6 mg/kg body weight) in the Cisplatin, Cisplatin+NDGA, and Cisplatin+EPO groups. Control rats received an identical volume of normal saline.
On day 4, serum and 24 hr urine samples were obtained to confirm the onset of ARF. After induction of ARF, rats were treated with vehicle, NDGA, or EPO from day 4 to 10 as follows; the 10 rats in the Cisplatin+NDGA group were injected daily with NDGA (10 mg/kg/day; s.c.), the 10 rats in the Cisplatin+EPO group with EPO (100 IU/kg/day; s.c.), the 10 rats in the Cisplatin group and the 10 rats in the control group were also injected s.c. with vehicle (volumes equivalent to the NDGA injections).
On days 1, 4, and 10, serum and 24 hr urine samples were collected to determine biochemical parameters. Serum samples were obtained from the retro-orbital plexus to measure hemoglobin (Hb), hematocrit (Hct), urea nitrogen (BUN), creatinine (SCr), sodium (Na), and potassium (K). Twenty four-hour urine samples were collected in metabolic cages to determine urine volumes, and urea nitrogen, Cr, Na, and K levels. Twenty four-hour urine Cr clearances (CCr) were viewed as surrogates of GFR.
On day 10, all rats were sacrificed by injecting ketamine (i.p.) under general anesthesia. Rats were perfused through left ventricles with saline and kidneys were removed immediately for histological examinations. Semi-quantitative analysis was performed on kidney sections in a blind manner. Tubular lesions were graded as follows (5): 0=no damage; 1+=areas of tubular epithelial cell swelling, vacuolization, necrosis, and desquamation <50%; 2+=lesion areas >50% with or without focal involvement of the S3 segment of medullary rays; 3+=lesion areas 100% with diffuse involvement of medullary rays. Immunostaining for proliferating cell nuclear antigen (PCNA) was performed using the ABC procedure (5). Kidneys were fixed in 15% formalin overnight, ethanol dehydrated, and paraffin embedded. Sections were then incubated for 3×5 min in a microwave oven for PCNA labeling, for 5 min with goat non-immune serum to reduce non-specific background staining, and for 1 hr at room temperature with specific antibody diluted in 10% goat serum. After a 10-min rinse in PBS, sections were incubated for 10 min at room temperature with a biotinylated goat anti-mouse IgG antibody, treated with avidin-biotin-peroxidase complex, and then with 3,3' diaminobezidine. The sections were counterstained with hematoxylin and mounted in Eukit. Each tissue sample stained for PCNA was viewed and scored by a pathologist blinded to the treatment received. Quantification of tubular cells was performed with an image analyzer computer system (Cambridge Instrument Q250 Rueil Malmaison, France) on 20 fields (area field: 0.109 mm2) outside the glomerular area. Renal tissue samples were divided into cortical and cortico-medullary area for quantification of staining (5).
On day 1, no significant differences were evident between the four groups in terms of body weight, serum Hb, BUN, SCr, 24 hr urine volume, Na and CCr (Table 1).
On day 4 (three days after injecting of cisplatin), rats in the Cisplatin, Cisplatin+NDGA, and Cisplatin+EPO groups lost weight (203.0±2.8 to 176.0±25.4, 206.0±5.5 to 168.0±5.7, and 203.0±2.7 to 169.0±6.5 g, respectively), whereas rats in the control group gained weight (203.0±2.7 to 226.0±6.5 g) (p<0.05). Mean serum BUN of the Cisplatin, Cisplatin+NDGA, and Cisplatin+EPO groups (the three treatment groups) were significantly higher (154.6±60.1, 209.6±35.0, and 155.8±43.7 mg/dL, respectively) than that of the control group (12.6±1.9 mg/dL) (p<0.05). Mean SCr of the three treatment groups were also significantly higher (2.0±0.7, 1.7±0.3, and 1.8±0.4 mg/dL, respectively) than that of the control group (0.4±0.1 mg/dL) (p<0.05). However, CCr were significantly lower in the three treatment groups (0.11±0.05, 0.12±0.10, and 0.21±0.07 mL/min, respectively) than in the control group (1.30±0.24 mL/min) (p<0.05), as were 24 hr urine Na concentrations (UNa) (37.4±17.5, 58.9±11.2, and 65.4±29.1 mL/min, respectively, vs. 135.8±5.2 mEq/L) (p<0.05). On the other hand, fractional excretions of Na (FENa) in the three treatment groups were significantly higher (3.3±3.8, 6.8±5.7, 3.7±1.5%, respectively) than in the control group (0.5±0.1%) (p<0.05), as were renal failure indices (RFI) (4.6±5.2, 9.3±7.6, and 5.1±2.1, respectively, vs. 0.7±0.1) (p<0.05) (Table 1).
On day 10 (after 7 daily injections of NDGA or EPO), controls gained weight (226.0±6.5 to 242.0±9.8 g), whereas Cisplatin group rats lost weight (176.0±25.4 to 172.0±10.9 g). Cisplatin+NDGA and Cisplatin+EPO groups were significantly heavier than Cisplatin group (217±12.0, and 188.0±7.6, respectively, vs. 172.0±10.9 g) (p<0.05).
On day 10, serum Hb levels in the Cisplatin+EPO group were significantly higher than in the Cisplatin and Cisplatin+NDGA groups (15.7±0.4 vs. 14.0±0.3 and 13.7±0.3 g/dL, respectively) (both p<0.05 vs. Cisplatin and Cisplatin+NDGA group).
On day 10, BUN concentrations in the Cisplatin+NDGA group were significantly decreased from day 4 (209.6±35.0 to 22.6±10.5 mg/dL) (p<0.05 vs. Cisplatin group). Similarly, BUN in the Cisplatin+EPO group were significantly decreased from day 4 (155.8±43.7 to 27.8±3.8 mg/dL) (p<0.05 vs. Cisplatin group) (Fig. 1A). SCr in the Cisplatin+NDGA and Cisplatin+EPO groups were also significantly decreased from day 4 (1.7±0.3 to 1.2±0.5, and 1.8±0.4 to 0.8±0.2 mg/dL, respectively) (both p<0.05 vs. Cisplatin group) (Fig. 1B). CCr in the Cisplatin+EPO group on day 10 recovered significantly from day 4 (0.21±0.07 to 0.94±0.49 mL/min) (p<0.05 vs. Cisplatin group). However, the Cisplatin+NDGA group showed only a tendency to recover (0.12±0.10 to 0.32±0.25 mL/min) (p>0.05) (Fig. 1C). UNa in the Cisplatin+NDGA group on day 10 were significantly increased from day 4 (58.9±11.2 to 78.7±10.6 mEq/L) (p<0.05 vs. Cisplatin group), but this was not the case in the Cisplatin+EPO group (Fig. 1D). FENa and RFI values in the Cisplatin+NDGA and Cisplatin+EPO groups showed a tendency to diminish from day 4 to day 10 (p>0.05).
All rats in the three treatment groups showed structural damage, although glomeruli and vessels were normal. Changes were limited to tubules, usually the proximal straight S3 portion in the outer medulla. Semi-quantitative assessments of histological lesions produced significantly higher scores for the three treatment groups than the normal controls at day 10. However, no significant differences were observed between the three treatment groups (Table 2, Fig. 2). Numbers of PCNA-positive cells were significantly higher in the cortical and cortico-medullary areas of kidneys in the Cisplatin, Cisplatin+NDGA and Cisplatin+EPO groups than in the normal controls. After treatments of NDGA, the number of PCNA-positive cells was significantly higher than in the Cisplatin group. However, no significant difference was observed in the Cisplatin+EPO group (Table 3, Fig. 3).
Cisplatin is widely known to induce ARF as a result of renal tubular epithelial cell injury. This cytotoxicity is considered to be due to several factors, e.g., the peroxidation of cell membranes, mitochondrial dysfunction, inhibition of protein synthesis, and DNA damage (19-22). Cisplatin accumulates in renal tubular cells to a concentration approximately five times higher than its extracellular concentration, and this uptake is most prominent in the S3 segment of proximal tubules. Experimental prevention of cisplatin-induced ARF has been achieved by intensive hydration (mainly with chloride containing salts) and by administering diuretics (23-25).
Recently, several researchers have suggested that human recombinant EPO might enhance recovery from cisplatin-induced ARF or ischemic injury in the rats. The data presented suggest that EPO accelerates renal recovery by inducing hemodynamic improvements and by stimulating tubular cell regeneration (5, 26). EPO is a cytokine that specifically regulates the differentiation and proliferation of erythroid progenitor cells, and the proliferating effect of EPO has also been demonstrated in other types of cells, like endothelial cells (7), which also possess an EPO receptor (27, 28). Furthermore, the expressions of specific high-affinity EPO binding sites have been demonstrated in rat and mouse megakaryocytes (29), which explain why platelet counts increase in patients on high dose EPO (30). Furthermore, tubular and mesangial cells also express EPO receptor mRNA (31), which suggests that EPO acts as a renotropic cytokine.
NDGA is a potent inhibitor of lipoxygenase activity and a strong antioxidant. It has been reported to reduce proteinuria and systolic blood pressure in diabetic rats, possibly via hemodynamic improvements induced, at least in part, by the maintenance of glutathione (GSH; an antioxidant enzyme) levels by NDGA within normal physiologic limits (32). In addition, NDGA is reported to protect ileal motor disturbances (16), to promote bowel adaptation (17), and to control coronary spasms of immune origin (18). Therefore we expected that NDGA might have regenerative and immunomodulating properties similar to EPO.
In the present study, we assessed the effects of NDGA and EPO on rapid renal recovery of cisplatin-induced ARF. Both EPO and NDGA were found to have a renoprotective effect and to accelerate the recovery of damaged renal tubular epithelial cells. EPO was found to accelerate renal recovery, which is in accordance with the results of previous studies. However, these indices may also have been affected by hemodynamic improvements due to the correction of anemia. NDGA also decreased BUN and SCr, and increased UNa significantly. In addition, NDGA also enhanced renal function without affecting Hb levels. PCNA is an auxiliary protein to DNA polymerase δ, and it is expressed in nucleus strongly in late G1 and S phases of the cell cycle. Therefore PCNA antibodies are used as tools for detecting proliferating cells. The present study showed more increased PCNA-positive cells in the Cisplatin+NDGA group than in the Cisplatin group, and also showed a tendency to increase in the Cisplatin+EPO group. These results suggest that NDGA might act directly via stimulation of tubular cell regeneration even in the setting of post-treatment.
The management of acute tubular necrosis still hinges on the prevention and treatment of uremic complications until renal function spontaneously recovers. A number of agents have been investigated to determine whether they can attenuate injury or hasten recovery in ischemic and nephrotoxic acute tubular necrosis, but unfortunately, no agent has been found to reduce the incidence of acute tubular necrosis or to reduce dialysis requirements or mortality.
The present study suggests that EPO and also NDGA may accelerate renal function recovery by stimulating tubular epithelial cell regeneration even after clinical onset of ARF. Furthermore, our findings indicate that NDGA has therapeutic potential, which we consider warrants further investigation in man.
Figures and Tables
Fig. 1
Changes of mean serum and 24 hr urine parameters in the four groups. (A) Significant reductions in BUN levels were observed in the Cisplatin+NDGA and Cisplatin+EPO groups between Day 4 and Day 10. (B) Significant serum creatinine decreases were observed in the Cisplatin+NDGA and Cisplatin+EPO groups between Day-4 and Day-10. (C) Significant creatinine clearance increases were observed in the Cisplatin+EPO group between Day-4 and Day-10. (D) Significant Na concentration increases in urine were observed in the Cisplatin+NDGA group between Day-4 and Day-10. *p<0.05 vs. Cisplatin group. Normal controls (dashed line,○), Cisplatin (□), Cisplatin+NDGA (•), Cisplatin+EPO (♦).
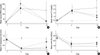
Fig. 2
Histological aspects of the rat corticomedullary junction 10 days after cisplatin administration showing tubular epithelial cell swelling, vacuolization, necrosis, and desquamation affecting primarily the proximal tubule. NDGA and EPO did not modify the effects of cisplatin on renal histology (×400, PAS).
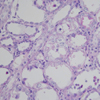
Fig. 3
PCNA-positive cells in Cisplatin+NDGA group. PCNA staining showing large nuclei of regenerating cells on day 10 (×400).
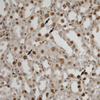
Table 1
Basal parameters before and after induction of acute renal failure in the four experimental groups
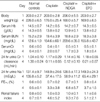
Table 2
Semi-quantitative evaluation of historical lesions at day 10
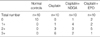
0=no damage; 1+=area of tubular epithelial cell swelling, vacuolization, necrosis, desquamation of <50%; 2+=lesion areas >50% with or without focal involvement of the S3 segment in the medullary rays; 3+=lesion areas 100% with diffuse involvement of medullary rays.
NDGA, nordihydroguaiaretic acid; EPO, erythropoietin; BUN, blood urea nitrogen.
References
2. Loehrer PJ, Einhorn LH. Drugs five years later. Cisplatin. Ann Intern Med. 1984. 100:704–713.
3. Dillman RO, Seagren SL, Propert KJ, Guerra J, Eaton WL, Perry MC, Carey RW, Frei EF 3rd, Green MR. A randomized trial of induction chemotherapy plus high dose radiation versus radiation alone in stage III non-small cell lung cancer. N Engl J Med. 1990. 323:940–945.
4. Blachley JD, Hill JB. Renal and electrolyte disturbances associated with cisplatin. Ann Intern Med. 1981. 95:628–632.


5. Bagnis C, Beaufils H, Jacquiaud C, Adabra Y, Jouanneau C, Le Nahour G, Jaudon MC, Bourbouze R, Jacobs C, Deray G. Erythropoietin enhances recovery after cisplatin-induced acute renal failure in the rat. Nephrol Dial Transplant. 2001. 16:932–938.


6. Jacobson LO, Goldwasser E, Fried W, Plzak L. Role of the kidney in erythropoiesis. Nature. 1957. 179:633–634.


7. Nagai T, Akizawa T, Nakashima Y, Kohjiro S, Nabeshima K, Kanamori N, Takayama K, Kinugasa E, Koshikawa S. Effects of rHuEpo on cellular proliferation and endothelin-1 production in cultured endothelial cells. Nephrol Dial Transplant. 1995. 10:1814–1819.
8. Carlini RG, Reyes AA, Rothstein M. Recombinant human erythropoietin stimulates angiogenesis in vitro. Kidney Int. 1995. 47:740–745.


9. Mabry TJ, Hunzinker JH, DiFeo DR. Creosote bush. Biology and chemistry of Larrea in new world deserts. 1979. Stroudsburg, Pa: Dowden, Hutchinson & Ross.
10. Brinker F. Larrea tridentata (DC) Coville (chaparral or creosote bush). Br J Phytotherapy. 1993. 3:10–31.
11. Argueta A, Gallardo Vazquez MC. Atlas of the Traditional Mexican Medicinal Plants (vol II). 1994. Mexico: National Indigenous Institute;669–670.
12. Foster S, Tyler VE. Tyler's Honest Herbal: a sensible guide to the use of herbs and related remedies. 1999. New York: Haworth Herbal Press;109–111.
13. Winkelman M. Ethnobotanical treatments of diabetes in Baja California Norte. Med Anthropol. 1989. 11:255–268.


14. Lara F, Marquez C. Medicinal plants from Mexico: composition, uses and biological activity. 1996. Mexico: UNAM;9–61.
16. Ezberci F, Tekin E, Onuk E. Protective effect of a lipoxygenase inhibitor, nordihydroguaiaretic acid, on the ileal motor disturbances induced by Serratia marcescens endotoxin in rats. Surg Today. 2001. 31:497–501.
17. Kollman-Bauerly KA, Thomas DL, Adrian TE, Lien EL, Vanderhoof JA. The role of eicosanoids in the process of adaptation following massive bowel resection in the rat. JPEN J Parenter Enteral Nutr. 2001. 25:275–281.


18. Marchenko HI, Kotsiuruba VM, Butovych IA, Sorochynskyi AE, Zrazhevska VK, Tumanovska LV. The correction of disorders in arachidonic acid metabolism in coronary spasm of an immune origin. Fiziol Zh. 1994. 40:81–87.
19. Sugiyama S, Hayakawa M, Kato T, Hanaki Y, Shimizu K, Ozawa T. Adverse effects of anti-cancer drug, cisplatin on rat kidney mitochondria: disturbances in glutathione peroxidase activity. Biochem Biophys Res Commun. 1989. 159:1121–1127.
20. Brady HR, Kone BC, Stromski ME, Zeidel ML, Giebisch G, Gullans SR. Mitochondrial injury: an early event in cisplatin toxicity to renal proximal tubules. Am J Physiol. 1990. 258:F1181–F1187.


21. Leibbrandt ME, Wolfgang GH, Metz AL, Ozobia AA, Haskins JR. Critical subcellular targets of cisplatin and related platinum analogs in renal proximal tubule cells. Kidney Int. 1995. 48:761–770.
22. Kharbanda S, Ren R, Pandey P, Shafman TD, Feller SM, Weichselbaum RR, Kufe DW. Activation of the c-Abl tyrosine kinase in the stress response to DNA-damaging agents. Nature. 1995. 376:785–788.
23. Heidemann TH, Gerkens JF, Jackson EK, Branch RA. Attenuation of cisplatinum-induced nephrotoxicity in the rat by high salt diet, furosemide and acetazolamide. Naunyn Schmiedebergs Arch Pharmacol. 1985. 329:201–205.


24. Daley-Yates PT, McBrien DC. A study of the protective effect of chloride salts on cisplatin nephrotoxicity. Biochem Pharmacol. 1985. 34:2363–2369.


25. Cvitkovic E, Spaulding J, Bethune V, Martin J, Whitmore WF. Improvement of Cis-dichlorodiamineplatinum (NCS 119875): therapeutic index in an animal model. Cancer. 1977. 39:1357–1361.
26. Nemoto T, Yokota N, Keane WF, Rabb H. Recombinant erythropoietin rapidly treats anemia in ischemic acute renal failure. Kidney Int. 2001. 59:246–251.


27. Anagnostou A, Lee ES, Kessimian N, Levinson R, Steiner M. Erythropoietin has a mitogenic effect and a positive chemotactic effect on endothelial cells. Proc Natl Acad Sci USA. 1990. 87:5978–5982.
28. Anagnostou A, Liu Z, Steiner M, Chin K, Lee ES, Kessimian N, Noguchi CT. Erythropoietin receptor mRNA expression in human endothelial cells. Proc Natl Acad Sci USA. 1994. 91:3974–3978.


29. Fraser JK, Tan AS, Lin FK, Berridge MV. Expression of specific high-affinity binding sites for erythropoietin on rat and mouse megakaryocytes. Exp Hematol. 1989. 17:10–16.
30. Kitagawa S, Masaki S, Miura Y. Recombinant human erythropoietin at high doses stimulates thrombopoiesis: treatment for protracted severe myelosuppression complicating interferon-alpha and busulfan therapy for chronic myelogenous leukemia. Eur J Haematol. 1995. 55:285–286.
31. Westenfelder C. Tubular and mesangial cells of human, rat and mouse kidney express authentic erythropoietin receptor mRNA. Nephrology. 1997. S22.