Abstract
Epithelial membrane protein 3 (EMP3) is a trans-membrane signaling molecule with important roles in the regulation of apoptosis, differentiation and invasion of cancer cells, but the detailed is largely still unknown. We analyzed the mRNA levels and methylation statuses of EMP3 in 63 primary breast carcinomas and assessed their correlations with clinicopathologic variables. The expression of EMP3 mRNA in primary breast carcinomas was significantly higher than the expression of 20 normal breast tissues (p<10-7). EMP3 overexpression in breast carcinomas was significantly related to histological grade III (p=3.9×10-7), lymph node metastasis (p=0.003), and strong Her-2 expression (p=3.3×10-6). Hypermethylation frequencies of EMP3 were detected in 36.5% of breast carcinomas by methylation-specific polymerase chain reaction. However, no significant correlations were found between methylation status of EMP3 and mRNA expression levels as well as other clinical parameters. In conclusion, EMP3 may be a novel marker of tumor aggressiveness. Overexpression of EMP3 in primary breast carcinoma is not associated with DNA methylation.
Primary breast cancer is one of the most common cancer for women. The dissemination of metastasis is the leading cause of breast cancer fatality. The metastatic process of malignant cells involves cell proliferation and cell-cell interactions, in which epithelial membrane protein 3 (EMP3) is thought to be involved (1).
The myelin-related gene EMP3 belongs to the peripheral myelin protein 22-kDa (PMP22) gene family of small hydrophobic membrane glycoproteins, which include four closely related members, PMP22, EMP1 (TMP), EMP2 (XMP), and EMP3 (YMP), as well as an additional and more distant, member, MP20 (2). The EMP3 gene is located on the 19q13.3 band, which encodes a protein similar to PMP22, EMP1 and EMP2 with approximately 40% of amino acids (1). EMP3 has high transcriptional expression in peripheral blood leukocytes, ovary, intestine and various embryonic tissues (1). The function of the EMP3 protein is largely unknown. But it may be involved in regulating cell proliferation and cell-cell interactions, based on the available data for PMP22 and EMP1 which are the best-characterized members of the family (3). Recent reports showed that overexpression of EMP3 positively associated with the invasive status of human mammary carcinoma cell lines (4-6).
It has emerged that epigenetic events can lead to gene inactivation that contributes to human neoplasia. The most important mechanism in mammalian cells is DNA methylation, the covalent addition of a methyl group to the 5'position of cytosine, predominantly within the CpG dinucleotide (7). An online search for CpG islands (http://ccnt.hsc.usc.edu/cpgislands/) in the 5' region of the EMP3 gene (GenBank accession number, NM001425) revealed a typical CpG island on the start site of the transcripton. It indicated that hypermethylation of the promoter regions of EMP3 likely plays an important role in the regulation of their mRNA levels in malignant tumors. Alaminos et al. (8) showed that the hypermethylation of the promoter region of the EMP3 gene was present in 24% of 116 neuroblastic primary tumors and 39% of 41 gliomas. On the contrary, Li et al. (9) recently reported that methylation alone does not mediate transcriptional down-regulation of EMP3 in oligodendroglial tumors, because their study with 29 oligodendroglial tumors showed no correlation between transcript level and methylation status. The methylation status of the EMP3 gene is still unknown in other human tumors. In the present study, we tried to analyze the methylation status of the EMP3 gene as well as its correlation with mRNA levels in human primary breast carcinoma.
Primary breast carcinoma samples (n=63) and normal breast tissues (n=20) were obtained from patients who were diagnosed with T1-T3, N0-N2 (stage I-IIIA) breast carcinoma and had been treated with curative resectional surgery between June 2004 and March 2006, in Shandong Cancer Hospital and Institute (Jinan, China). Among them, 9 patients had stage I, 25 patients had stage IIA, 13 patients had stage IIB, and 16 patients had stage IIIA disease. The median age of the patients was 51 yr (range, 32-80). There were 34 node-negative and 29 node-positive patients (12 with one to three positive nodes, and 17 with more than three positive nodes). Histological subtypes included 43 invasive ductal, 6 invasive lobular, 6 mixed type (ductal/lobular), 7 mucinous carcinomas and 1 ductal carcinoma in situ, which were classified according to the WHO classification (10). All the breast tissue samples were collected from patients, who had neither chemotherapy nor radiotherapy before operation. They were collected with the informed consent of all patients. The study was approved by the ethics committees of Shandong Cancer Hospital. The collected specimens were snap-frozen in liquid nitrogen and kept at -80℃.
Standard immunohistochemical detection, with minor modifications, was performed with archival paraffin embedded breast tumor tissues and corresponding nonmalignant breast tissues. In brief, 4 µm thick histological sections were mounted on 0.1% poly-L-lysine treated slides, dried overnight at 37℃, and subsequently deparaffinized and rehydrated. Protein expressions in malignant and nonmalignant breast tissues were detected with specific antibodies against the estrogen receptor (ER) (Zymed, San Francisco, CA, U.S.A.; prediluted monoclonal mouse anti-human estrogen receptor clone 1D5, incubated for 18 hr at 4℃), the progesterone receptor (PR) (Zymed; prediluted monoclonal mouse anti-human progesterone receptor clone PR-2C5, incubated for 18 hr at 4℃), Her-2 (Zymed; prediluted monoclonal mouse anti-human Her-2 clone TAB250, incubated for 30 min at 37℃), p53 (Zymed; prediluted monoclonal mouse anti-human p53 protein clone BP53.12, incubated for one hour at 37℃), and Ki-67 (Zymed; prediluted monoclonal mouse anti-human Ki-67 antigen clone 7B11, incubated for one hour at 37℃). In addition, positive and negative controls for each marker were routinely performed during experiments.
Sections were then processed using the Histostain-SP kits according to the manufacturer's recommendations (Zymed). Interpretation of the staining was carried out according to the usual criteria (11) by two independent blinded observers. If any discrepancies between classifications of samples arose, they were reviewed and the final results reached by consensus. In brief, ER, PR, and p53 were scored for the immunohistochemical signal as follows: weak (1+), moderate (2+), and strong (3+) staining in >10% of the tumor cells or absent (0). Levels of Her-2 protein expression were evaluated semi-quantitatively using Zymed evaluation guidelines, as follows: no staining or membrane staining in less than 10% of the tumor cells (0), weak, incomplete membrane staining in more than 10% of the tumor cells (1+), weak or moderate complete membrane staining in more than 10% of the tumor cells (2+), and strong, complete membrane staining in more than 10% of the tumor cells (3+). Further, for Ki-67 expression we evaluated the percentage of cells stained.
Total RNA was extracted from frozen tissues using the Trizol reagent (Invitrogen, Carlsbad, CA, U.S.A.) following the manufacturer's instructions. RNA was treated with DNase and purified using the RNeasy mini kit (Qiagen, Hilden, Germany). Purified RNA was eluted in 30 µL of the RNA storage solution (Ambion, Austin, TX, U.S.A.) and stored at -80℃. The total RNA was quantified by UV-spectroscopy (U-3000 Spectrophotometer, Hitachi, Tokyo, Japan). cDNA synthesis was conducted using the high capacity cDNA archive kit (Applied Biosystems, Foster City, CA, U.S.A.) according to the manufacturer's instructions. The cDNA samples were used for reverse transcriptase-polymerase chain reaction (RT-PCR) analysis.
The primers of EMP3 gene were designed by the software of Primer 5 according to the Gene Bank sequence (NM 001425). The upward and downward primers were designed to span two exons to avoid any amplification of genomic DNA. The primer pairs of EMP3 were 5'-CGA GAA TGG CTG GCT GAA G-3', 5'-GCC ACG CTG GTG CAA AG-3', (156 bp). Glyceraldehyde-3-phosphate dehydrogenase (GAPDH) mRNA was also amplified in the same PCR reactions as an internal control using the following primers: 5'-GAA GGT GAA GGT CGG AGT C-3', 5'-GAA GAT GGT GAT GGG ATT TC-3', (226 bp, designed by Applied Biosystems). For semi-quantitative RT-PCR analysis, 1 µL of cDNA was added in a tube containing 10×Reaction Buffer 1 µL, 4×10 mM dNTP 0.4 µL, 10 µM of each primer 0.5 µL, 5 U/µL Taq DNA polymerase 0.1 µL (Promega, Madison, WI, U.S.A.) in a final volume of 10 µL (12). Cycling conditions were denaturation at 94℃ for 5 min, then 35 cycles of PCR (94℃, 30 sec; 60℃, 30 sec; and 72℃, 30 sec), followed by a final extension of 72℃ for 10 min. The amplification products were resolved by 3% agarose gel electrophoresis and visualized by ethidium bromide staining.
Quantitative real-time PCR was carried out using the specific primers as mentioned above. GAPDH mRNA was also amplified as an internal control. Briefly, 2 µL of cDNA was used to determine gene expression levels by the ABI PRISM® 7000HT Sequence Detection Systems (Applied Biosystems). It was amplified in the 25 µL reaction including 2×SYBR Premix Ex Taq™ 12.5 µL (Takara, Shiga, Japan), 10 µM of each primer 0.5 µL, 50×ROX Reference Dye 0.5 µL and H2O 9 µL. Cycling conditions included a hot start (15 sec, 95℃), followed by 40 cycles of 95℃, 10 sec and 60℃, 40 sec. For analysis, a threshold was set for the change in fluorescence at a point in the linear PCR amplification phase. Melt curve analysis was performed to ensure a single product species. All experiments were done in duplicate for both target gene and internal control and repeated three independent times. For the ΔΔCT calculation to be valid, the amplification efficiencies of the target and reference must be approximately equal. The standard curves used to determine relative expression were obtained by running real-time PCR for a diluted sample, for example, to 1:1, 1:10, 1:100, and 1:1,000. After control for equal PCR efficiency of target genes and internal controls, relative gene expressions were presented with the comparative Ct method of quantification (13). Briefly, this technique uses the formula 2-ΔΔCT to calculate the relative expression of target genes normalized to a calibrator. The threshold cycle (CT) indicates the cycle number at which the amount of amplified target reaches a fixed threshold. The level of genes was calculated by comparing ΔCT values of the sample (ΔCT=CT [target gene]-CT [GAPDH gene]) to that of the calibrator; that is ΔΔCT=ΔCT (tumor sample)-ΔCT (calibrator).
Genomic DNA from tissue specimens was isolated according to a standard proteinase K digestion and phenol/chloroform extraction protocol with some modifications (14). In brief, the materials were grounded to a fine powder under liquid nitrogen. Frozen pulverized powders of the specimens were incubated overnight at 52℃, in 500 µL lytic solution (10 mM Tris-HCl pH 8.0, 0.1 M EDTA, 0.5% SDS) containing proteinase K (Roche, Indianapolis, IN, U.S.A.). These were followed by extraction with equal volumes of phenol: chloroform:isoamyl alcohol (25:24:1). DNA was ethanol precipitated at -20℃, washed with 70% ethanol, dried and resuspended in TE buffer. The DNA concentrations were calculated according to the OD 260 nm readings.
For DNA methylation analysis, sodium bisulfite modification of genomic DNA was performed, as described previously with minor modifications (15). Briefly, 1 µg of genomic DNA was denatured at 42℃ for 30 min, in a total volume of 50 µL containing NaOH (final concentration, 0.2 M). After the addition of 30 µL freshly prepared 10 mM hydroquinone (Sigma, St. Louis, MO, U.S.A.) and 520 µL of 3 M sodium bisulfite (Sigma) at pH 5.0, the samples were incubated 16 hr at 55℃ in the dark. Afterward, modified DNA was purified using Wizard DNA clean-up resin (Promega A7280), and DNA was desulfonated in 0.3 M NaOH at 37℃ for 15 min. Treatment of genomic DNA with sodium bisulfite converts unmethylated cytosines (but not methylated cytosines) to uracil, which are then converted to thymidine during the subsequent PCR step, giving sequence differences between methylated and unmethylated DNA. The converted DNA was precipitated at -20℃, overnight in ice-cold 100% ethanol containing ammonium acetate (final concentration, 3 M) and 10 µg of glycogen. DNA was resuspended in deionized water, and used immediately or stored at -20℃.
Bisulfite-modified DNA was amplified with primers specific for methylated or unmethylated sequences. The methylated-specific and unmethylated-specific primers (8) covered the transcription start site of EMP3. The methylated DNA of EMP3 (144 bp) was amplified using M set primers, 5'-GAC GTA GAA GGA GAG CGA GC-3'(sense), 5'-CCT CGC TCG AAC CTC CGT A-3'(antisense), and the unmethylated DNA of EMP3 (149 bp) was amplified using U set primers, 5'-GAA GAG ATG TAG AAG GAG AGT GAG T-3'(sense), 5'-CTT ATC CCT CAC TCA AAC CTC CAT A-3'(antisense). The PCR mixture contained 2×GC buffer 5 µL (Takara), 4×10 mM dNTP 0.4 µL, 10 µM of each primer 0.5 µL, 5 U/µL Takara LA Taq DNA polymerase 0.1 µL (Takara), and 2 µL bisulfite-modified DNA. Amplification was carried out as follows: after initial denaturation at 95℃ for 5 min, 40 cycles of amplification at 95℃ for 30 sec, 58℃ (methylated or unmethylated) for 30 sec, and 72℃ for 30 sec, followed by a final extension of 72℃ for 10 min. DNA from peripheral blood lymphocytes of healthy individuals was used as a positive control for unmethylated alleles. DNA from peripheral normal blood lymphocytes treated in vitro with SssI methyltransferase (New England Biolabs, Ipswich, MA, U.S.A.) was used as a positive control for methylated alleles. Ten µL of each PCR reaction were loaded and run onto 3% agarose gels stained with ethidium bromide and visualized under UV illumination. The PCR for all samples demonstrating methylation status of the EMP3 gene was repeated to confirm these results.
Descriptive statistics, such as mean, standard deviation, and percentage were used to summarize a patient's data and gene expression or methylation status. The immunohistological markers were defined into dichotomous variables on the basis of the staining index such as negative/reduced expression levels (scores 0, 1, and 2) and positive/maximum staining (score 3) for ER, PR, Her-2 proteins. To p53 protein, the expression level was categorized as negative (scores 0 and 1) or positive (scores 2 and 3). The comparison of EMP3 mRNA levels among various subgroups such as tumor sizes, histologic grade, and lymph node status was calculated using the Kruskal-Wallis test. The EMP3 mRNA expression levels between different sex, immunohistological, and methylation status teams were evaluated by using the Mann-Whitney test. The correlations of the methylation statuses of EMP3 with clinicopathologic parameters were analyzed by chi-square test. Statistical significance was assumed for p<0.05. The statistical tests were performed using the statistical software package SPSS, version 12.0 (LEAD Technologies, Athens, GA, U.S.A.).
The mRNA levels of EMP3 were analyzed in all the 63 primary breast carcinomas and the 20 normal breast tissues by conventional RT-PCR (Fig. 1A). The results showed that the mRNA of EMP3 was over-expressed in breast carcinomas as compared with in normal breast tissues. EMP3 expression status determined by real-time PCR assay is shown according to the various clinical, pathological, and biological parameters in Table 1. The expression of EMP3 mRNA in primary breast carcinomas was significantly higher than the expression of 20 normal breast tissues (p<10-7). Furthermore, EMP3 overexpression in primary breast carcinomas was significantly related to histological grade III (p=3.9×10-7), lymph node metastasis (p=0.003), and strong Her-2 expression (p=3.3×10-6). Other parameters such as age, menopausal status, histological subtype and macroscopic tumor size did not show significant correlations with EMP3 mRNA levels.
The frequencies of methylation of EMP3 were determined in the 63 resected primary breast carcinomas and in the 20 corresponding nonmalignant breast tissues by methylation-specific PCR (Fig. 1B). Twenty-three of 63 (36.5%) tumors showed EMP3 methylation. In the normal breast tissues, methylation of EMP3 was not detected. The incomplete methylation form of EMP3 was detected in 82.6% (19/23) of methylated samples in breast carcinomas. Statistical analysis showed that there were no significant links between methylation frequencies and clinical, pathological or biological parameters (Table 2).
Of the 23 breast carcinomas exhibiting hypermethylation at the promoter region of EMP3, 1 (4.3%) showed low expression level, and 22 (95.7%) exhibited high EMP3 expression levels when compared to normal breast tissues. Of the 40 breast carcinomas which the promoter region of EMP3 was unmethylated, 3 (7.5%) showed expression levels similar to the normal samples, and 37 (92.5%) showed high expression levels of EMP3 compared with the control samples where EMP3 was also unmethylated. The Mann-Whitney test showed no significant difference (p=0.418) of EMP3 expression levels between CXCL12 methylated (2.59±2.30) and unmethylated breast carcinomas (2.13±1.91) (Fig. 2).
Recently, the EMP3 gene was reported to have reduced transcriptional expression in primary neuroblastoma, an extracranial malignant solid tumor of childhood (8). Reintroduction of EMP3 in deficient neuroblastoma cell lines induced lower colony formation density and tumor growth in nude mice (8). Thus, Alaminos et al. proposed that the EMP3 gene, located on chromosome 19q13, is a candidate tumor suppressor gene in neuroblastomas (8). In the present study, we used real-time quantitative RT-PCR assays to determine the mRNA expression levels of EMP3 in tumors different from neuroblastoma in tissue origin and age of onset: primary breast carcinoma. Meanwhile, we sought relationships between EMP3 expression status and clinical, pathological, and biological parameters. However, we found that the expression of EMP3 mRNA in primary breast carcinomas was significantly higher than the expression of 20 normal breast tissues (p<10-7). EMP3 overexpression in primary breast carcinomas was significantly related to histological grade III, lymph node metastasis and strong Her-2 expression. These data are consistent with reports indicating that overexpressions of EMP3 are significantly associated with the invasive status of human mammary carcinoma cell lines (3-6). The EMP3 gene was found to be significantly up-regulated (>2.0-fold) in the Her-2-overexpressing breast cancer cell line as compared with in the normal mammary luminal epithelial cell line (4). Taken together with previous studies about EMP3 expression status, we hypothesized that EMP3 may display different expression pattern in different histopathological tumors.
Until now, the studies on the EMP3 gene are rarely reported and its function is largely still unknown. Recent evidence indicates that EMP3 may participate in the process of cell apoptosis (16). EMP3 as well as the other members of the epithelial membrane protein family (EMP-1, EMP-2, and PMP-22) was found to be expressed in HEK-293 cells, which also express P2X7 receptors. The constitutive overexpression of any of the EMPs in HEK-293 cells, through its interaction with P2X7 receptor, led to cell blebbing, annexin V binding, and cell death by a caspase-dependent pathway (16). Nevertheless, EMP1, PMP-22, and EMP3 were found to be highly expressed in invasive mammary carcinoma cells (3, 6, 17). Thus, the role of EMP3, whether as a candidate tumor suppressor gene or an indicator of tumor progression, remains to be established. Absence of the unitary conception of EMP3 behavior requires a comprehensive assessment of EMP3 expression and functional mechanism in different histopathological tumors.
We sought to define a possible mechanism regulating the expression of EMP3. Epigenetic events such as DNA methylation are thought to be a major early event in tumor development (18). A main target of the regional hypermethylation is normally CpG islands located in gene promoter regions. The 5' region of the EMP3 gene revealed a typical CpG island on the start site of the transcripton. We subsequently analyzed the methylation status of EMP3 and its relationship with mRNA expression in primary breast carcinoma. We found that EMP3 was hypermethylated 36.5% in 63 primary breast carcinomas. But we did not find significant statistical correlation between methylation status of EMP3 and EMP3 expression level as well as histologic subtype, tumor grade, age and gender. Consistent with our findings, Li et al. (9) reported that no correlation was found between transcript level and methylation status in oligodendroglial tumors, though all oligodendroglial tumors except 3 showed aberrant methylation of EMP3. Taken together, links between EMP3 methylation status and the mode of its expression in different tumors need further study.
In addition to the role of DNA methylation, other regulatory mechanisms may be involved in the regulation of EMP3. Burmester et al. (19) tested candidate SNPs from genomic regions that show linkage to prostate cancer susceptibility and/or aggressiveness. The polymorphism in EMP3 changes amino acid 125 from Val to Ile (19). Significant difference (p<0.0001) in allele frequencies of SNP was observed for EMP3 between prostate cancer sibships and controls (19). And the association remained significant when cases and controls were matched for age (19). In addition, Li et al. (9) reported that EMP3 overexpression was associated with oligodendroglial tumors retaining chromosome arms 1p and 19q, whereas decreased EMP3 expression was associated with deletion of chromosome 1p, not 19q. However, this notion is at variance with the study from Kunitz et al. (20). They found that low EMP3 expression was significantly associated with 19q deletion in oligodendroglial tumors, but not in astrocytic tumors (20). Obviously, the real regulatory mechanism of EMP3 expression remains to be elucidated. Given the variable expression patterns of EMP3 in different malignant tumors, the pathway leading to this diversity may also be quite different, which needs to be further investigated by additional genetic or biochemical studies.
Figures and Tables
Fig. 1
Expression and methylation analyses of EMP3 in primary breast carcinomas. (A) Semi-quantitative RT-PCR analysis showing EMP3 mRNA is over-expressed in primary breast carcinoma (TB) as compared with their corresponding nonmalignant tissues (NB). (B) Methylation analysis of EMP3 in primary breast carcinomas (TB) by methylation-specific PCR.
Lane U, unmethylated; Lane M, methylated. Data in (A, B) are representative of two independent experiments.
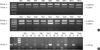
Fig. 2
EMP3 mRNA levels were assayed by real-time PCR and compared between tumors with hypermethylation (M) and without hypermethylation (UM).
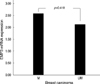
ACKNOWLEDGMENTS
We thank the staff of the Breast Cancer Center, Shandong Cancer Hospital and Institute for assistance in patient care and specimen collection. We also thank Xiaotian Chang, of Shandong Academy of Medical Sciences, for writing assistance.
References
1. Taylor V, Suter U. Epithelial membrane protein-2 and epithelial membrane protein-3: two novel members of the peripheral myelin protein 22 gene family. Gene. 1996. 175:115–120.


2. Ben-Porath I, Kozak CA, Benvenisty N. Chromosomal mapping of Tmp (Emp1), Xmp (Emp2), and Ymp (Emp3), genes encoding membrane proteins related to Pmp22. Genomics. 1998. 49:443–447.
3. Ben-Porath I, Benvenisty N. Characterization of a tumor-associated gene, a member of a novel family of genes encoding membrane glycoproteins. Gene. 1996. 183:69–75.


4. Mackay A, Jones C, Dexter T, Silva RL, Bulmer K, Jones A, Simpson P, Harris RA, Jat PS, Neville AM, Reis LF, Lakhani SR, O'Hare MJ. cDNA microarray analysis of genes associated with ERBB2 (HER2/neu) overexpression in human mammary luminal epithelial cells. Oncogene. 2003. 22:2680–2688.


5. Evtimova V, Zeillinger R, Weidle UH. Identification of genes associated with the invasive status of human mammary carcinoma cell lines by transcriptional profiling. Tumour Biol. 2003. 24:189–198.


6. Schiemann S, Ruckels M, Engelholm LH, Schwirzke M, Brunner N, Weidle UH. Differential gene expression in human mammary carcinoma cells: identification of a new member of a receptor family. Anticancer Res. 1997. 17:13–20.
8. Alaminos M, Davalos V, Ropero S, Setien F, Paz MF, Herranz M, Fraga MF, Mora J, Cheung NK, Gerald WL, Esteller M. EMP3, a myelin-related gene located in the critical 19q13.3 region, is epigenetically silenced and exhibits features of a candidate tumor suppressor in glioma and neuroblastoma. Cancer Res. 2005. 65:2565–2571.


9. Li KK, Pang JC, Chung NY, Ng YL, Chan NH, Zhou L, Poon WS, Ng HK. EMP3 overexpression is associated with oligodendroglial tumors retaining chromosome arms 1p and 19q. Int J Cancer. 2007. 120:947–950.


10. World Health Organisation. International Histological Classification of Tumours No. 2. Histological typing of breast tumours. 1981. 2nd ed. Geneva: World Health Organisation.
11. Caldeira JR, Prando EC, Quevedo FC, Neto FA, Rainho CA, Rogatto SR. CDH1 promoter hypermethylation and E-cadherin protein expression in infiltrating breast cancer. BMC Cancer. 2006. 6:48.


12. Hwang KT, Han W, Bae JY, Hwang SE, Shin HJ, Lee JE, Kim SW, Min HJ, Noh DY. Downregulation of the RUNX3 gene by promoter hypermethylation and hemizygous deletion in breast cancer. J Korean Med Sci. 2007. 22:Suppl. S24–S31.


13. Livak KJ, Schmittgen TD. Analysis of relative gene expression data using real-time quantitative PCR and the 2(-Delta Delta C(T)) Method. Methods. 2001. 25:402–408.
14. Goelz SE, Hamilton SR, Vogelstein B. Purification of DNA from formaldehyde fixed and paraffin embedded human tissue. Biochem Biophys Res Commun. 1985. 130:118–126.


15. Grunau C, Clark SJ, Rosenthal A. Bisulfite genomic sequencing: systematic investigation of critical experimental parameters. Nucleic Acids Res. 2001. 29:E65–E65.


16. Wilson HL, Wilson SA, Surprenant A, North RA. Epithelial membrane proteins induce membrane blebbing and interact with the P2X7 receptor C terminus. J Biol Chem. 2002. 277:34017–34023.


17. Huhne K, Park O, Liehr T, Rautenstrauss B. Expression analysis of thö PMP22 gene in glioma and osteogenic sarcoma cell lines. J Neurosci Res. 1999. 58:624–631.
18. Baylin SB, Herman JG, Graff JR, Vertino PM, Issa JP. Alterations in DNA methylation: a fundamental aspect of neoplasia. Adv Cancer Res. 1998. 72:141–196.