Abstract
The myocardial protective effects of endothelin antagonist in ischemic cardiomyopathy (ICMP), doxorubicin-induced cardiomyopathy (DOX) and pressure-overload hypertrophy by transverse aortic constriction (TAC) models have been predicted to be different. The objective of this experiment, therefore, is to evaluate the myocardial protective effect of tezosentan, an endothelin receptor antagonist, in various experimental heart failure models. Sprague-Dawley rats (6-8 weeks old, 200-300 g) were randomized to three experimental groups (n=30 each): ICMP; DOX; and TAC group. Each of these groups was randomly assigned further to the following subgroups (n=10 each): sham-operated ischemia-reperfusion subgroup (SHAM); tezosentan treated ischemia-reperfusion subgroup (Tezo); and tezosentan non-treated ischemia-reperfusion subgroup (N-Tezo). Total circulatory arrest was induced for 1 hr, followed by 2 hr of reperfusion. The left ventricular developed pressure, peak positive and negative first derivatives, and coronary blood flow were significantly different (P<0.05) among the SHAM, Tezo, and N-Tezo subgroups of the ICMP group at 30 min of reperfusion, but there were no statistically significant differences among the subgroups of the DOX and TAC groups. In conclusion, tezosentan, an endothelin receptor antagonist, showed myocardial protection effects only on the ischemic cardiomyopathy rat model, but not in the non-ischemic heart failure rat models.
Endothelin has been identified as a neurohormone that is activated during heart failure, and also by renin-angiotensin-aldosterone, epinephrine-norepinephrine, and natriuretic peptide. In addition, a number of studies have shown that endothelin-1 and its receptors over ischemic myocardial tissue are increased during ischemia-reperfusion injury. The endothelin-1 is the principal isoform in disease states and also is the most potent vasoconstrictor. It induces myocardial injury during ischemia and reperfusion as a contributor (1).
There are numerous reports about the relationship between endothelin and congestive heart failures (CHF). Some authors showed that endothelin-1 is an independent, noninvasive predictor of functional and hemodynamic response to therapy in patients with CHF. The majority of heart failure is caused by cardiomyopathy, and the increased endothelin levels have also been observed in the cardiomyopathy heart such as cardiomyopathic Syrian hamsters (2) or in the experimental Chagas' cardiomyopathic hearts infected with Trypanosoma cruzi (3).
Tezosentan, the first intravenous endothelin A and B receptor antagonist, has been evaluated as the treatment for acute and chronic heart failure. There are two large randomized controlled studies about tezosentan on acute heart failure syndrome-RITZ (randomized intravenous tezosentan study) and VERITAS (value of endothelin receptor inhibition with tezosentan in acute heart failure studies) (4, 5). Although these studies demonstrated mixed results of tezosentan as the drug of treatment for decompensated heart failure, the roles of the myocardial protective effect of tezosentan in various heart failure models have not yet been defined. The objectives of this experiment are to evaluate the myocardial protective effects of tezosentan in various experimental heart failure models.
Total 90 Sprague-Dawley male rats (6-8 weeks old, 200-300 g) were housed at 20±2℃ and 55±20% humidity with 12-hr light/dark cycles, and free access to food and water in the Animal Care Facility at the Ansan Hospital, Korea University, Korea. This study was approved by the Committee of Animal care of Korea University and conforms with the Guide for the Care and Use of Laboratory Animals published by the US National Institutes of Health (NIH Publication No. 85-23, revised 1996).
Rats were anesthetized with ketamine (100 mg/kg) andxylazine (10 mg/kg), intraperitoneally. The endotracheal intubation was performed, and positive pressure ventilation was given using a mechanical ventilator (Harvard Apparatus, South Natick, MA, U.S.A.) with room air at a respiratory rate of 55-65 strokes/min and tidal volume of 10 mL/kg body weight to maintain normal PO2, PCO2 and pH of blood.
Under the right lateral decubitus position, the left anterior descending coronary artery was ligated with a 6-0 silk suture through left anterior thoracotomy. The lungs were then inflated and the chest incision was closed. After recovery of self respiration, extubation was performed.
Doxorubicin hydrochloride (D1515, Sigma Chemical Co, St. Louis, MO, U.S.A.) was administrated in 6 equal injections (each intraperitoneal injection containing 2.5 mg/kg in saline, for a total dose of 15 mg/kg) during 2 weeks. Rats were observed for their general appearance, behavior, and mortality for 4 weeks after the final injection.
Under sterile condition, the midline cervical incision was performed; 1-2 cm incision was made at the level of cricoid process and dissection down to the clavicle. After cutting of the right clavicle, the thymus was retracted gently, and the aortic arch was exposed. A constriction of the aortic arch was conducted between the both carotid arteries using a 16 gauze angiocatheter (internal diameter 0.6 mm).
We divided the experimental animals into three cardiomyopathy groups; ischemic dilated cardiomyopathy group (ICMP, n=30), doxorubicin-induced cardiomyopathy group (DOX, n=30) and pressure-overload hypertrophy group by transverse aortic constriction method (TAC, n=30). One month after the construction of experimental rat groups, each group was further randomly subdivided to a sham-operated ischemia-reperfusion subgroup (SHAM) operation (SHAM, n=10), tezosentan treatment ischemia-reperfusion subgroup (Tezo, n=10), or tezosentan non-treatment ischemia-reperfusion subgroup (N-Tezo, n=10) (Fig. 1A). In the SHAM subgroup, only open and closure procedures or injection of saline instead of doxorubicin were performed.
Four weeks after the construction of cardiomyopathy models, the rats were anesthetized with sodium pentobarbital (50 mg/kg, intraperitoneally). Hearts were rapidly excised, connected via the aorta to Langendorff apparatus (size 3, type 830, Hugo Sachs Elektronik, March-Hugstetten, Germany) and perfused retrogradely at a constant pressure of 80 mmHg with the perfusate (Krebs-Henseleit solution) of the following composition: NaCl, 118.1 mM; KCl, 4.6 mM; CaCl2, 2.5 mM; MgSO4, 1.2 mM; KH2PO4, 1.2 mM; NaHCO3, 24.8 mM; and glucose, 10 mM. The perfusate was continuously bubbled with a gas mixture of 95% O2/5% CO2 (pH 7.4), and temperature was maintained at 37℃ throughout the experiment. A latex balloon filled with water was inserted into the left ventricle through the left atrium and attached to a pressure transducer (DX-360; Nihon Kohden, Tokyo, Japan). After 30 min of stabilization period, total circulatory arrest was performed with cardioplegia and coldness about 1 hr. After then, reperfusion was continued for 2 hr. In Tezo subgroup, tezosentan 10-5 M/L was added to the cardioplegic solution and the perfusate (Fig. 1B), but not to N-Tezo subgroup.
Tezosentan (Ro 47-0203, Actelion Pharmaceuticals Ltd, Allschwil, Switzerland) is a non-selective specially formulated intravenous dual (endothelin-A and -B) endothelin receptor antagonist. Unlike its sister compound (bosentan), tezosentan is easily soluble in water and stable in solution at physiologic pH, therefore, usable clinically. Another drug, doxorubicin hydrochloride (D1515, Sigma Chemical Co) is well known for cardioselective toxicity and induces congestive heart failure.
The left ventricular pressure was monitored with a pressure transducer to obtain the peak positive and negative first derivatives (dP/dTmax and dP/dTmin), and the left ventricular developed pressure (LVDP) was calculated as the difference between the left ventricular (LV) systolic and diastolic pressures. These parameters were measured by an amplifier (AP601G; Nihon Kohden) and also recorded using PowerLab/4sp software (AD Instruments, Mountain View, CA, U.S.A.). Coronary flow was also determined by collecting the coronary effluent from the hearts.
LVDP of Tezo subgroup was significantly higher than that of N-Tezo subgroup in the ICMP group after 30 min of reperfusion (64.3±7.1 mmHg vs. 41.4±4.3 mmHg; P<0.05). In the DOX or TAC model, LVDPs were not significantly different between Tezo and N-Tezo subgroup during the entire reperfusion period (Fig. 2A, Table 1).
In the ICMP group, dP/dTmax was significantly different among the three subgroups at 30 min of reperfusion (SHAM, 3,491±21 mmHg/sec; Tezo, 2,821±13 mmHg/sec; N-Tezo, 2,282±182 mmHg/sec; P<0.05). In the DOX or TAC groups, there were no statistical differences among three subgroups (Fig. 2B, Table 1).
In the ICMP group, dP/dTmin was significantly different among the three subgroups at 30 min of reperfusion (SHAM, -2,382±114 mmHg/sec; Tezo, -1,638±130 mm Hg/sec; N-Tezo, -1,387±81; P<0.05), similar to the above dP/dTmax. In the DOX or TAC groups, there were no statistically significant differences among the three subgroups (Fig. 2C, Table 1).
The effect of endothelin-1, the principal isoform of endothelin, is mediated by 2 types of receptor, endothelin-A and endothelin-B. The endothelin-A receptor appears to mediate vasoconstriction and stimulate the secretion of atrial natriuretic peptide, while the endothelin-B receptor mediates endothelin-induced vasodilatation and activation of the renin-angiotensin-aldosterone system. Through these effects, endothelin has been demonstrated to be not only one of the most potent vasoconstrictors known, but also to mediate pathologic hypertrophy and fibrosis of both ventricular and vascular tissues, thus promoting progression of atherosclerosis, ventricular and vascular remodeling (6-8).
Tezosentan, a dual inhibitor of endothelin A and B receptors, is weakly acidic, highly soluble in water and stable in physiologic pH solution. These properties make it an ideal candidate for intravenous use, and are the reason of why we used tezosentan in our study.
Heart failure has been associated with elevated plasma levels of endothelin-1, and the magnitude of the elevation with the severity of disease, the incidence of arrhythmia, and a worse prognosis. Increased endothelin levels have been described in patients with acute and chronic CHF that are predictive of increased mortality risk (9). Furthermore, increased endothelin levels have been suggested to play an important role in the increased systemic vascular resistance seen in CHF (10). Therefore, endothelin seems to play a major role in the pathogenesis of experimental and clinical heart failure (11, 12).
Although the endothelin receptor antagonists may have the ability to improve the outcome of heart failure by reducing preload and afterload, increasing the contractility of failing myocardium, delaying myocyte hypertrophy, and reducing the incidence of arrhythmias, some studies demonstrated no benefit of the endothelin receptor antagonists for myocardial ischemia-reperfusion heart models (13-15). Therefore, we aimed to investigate the myocardial protective effects of tezosentan for the ischemia-reperfusion injury in various heart failure models.
Before analyzing our experimental data, it was necessary to confirm the in-depth of animal heart failure models. Therefore, one month after cardiomyopathic animal models had been constructed, they were verified with pathologic specimens during euthanasia of the experimental animals. In the ICMP models, staining of triphenyltetrazolium chloride with evans blue was used for visualizing ischemia-infarct area of myocardium. In the DOX models, dilated myocardium was confirmed with pathologic specimens and hypertrophied myocardium was also confirmed in the TAC models (Fig. 3).
We also believe that hemodynamic parameters such as LVDP, dP/dTmax, dP/dTmin and CBF are adequate in our present study to verify myocardial protective effect of tezosentan in various rat cardiomyopathy models. It should be mentioned that these parameters have been used for proving drug efficacy in Langendorff technique (16).
As a result, our present study did not reveal any significant effect of tezosentan on DOX and TAC models. There are some hypotheses and limitations on this result in our study. First, although heart failure has been associated with elevated plasma levels of endothelin-1, the density of endothelin and endothelin binding sites had been produced in the heart has not been uniform among different animal heart failure models (17). In myocardial infarct model, it is well established that the infarcted myocardium induces the myocardial necrosis, and increases endothelin-1 levels many fold not only in the post-acute myocardial infarct scar tissues but also in the healed infarct scars after 4 and 13 weeks (18-20). Therefore, endothelin-1, a recognized fibrogenic factor, plays a crucial role in the stabilization of the necrotic area and in the healing of the scar (20). However, the most prominent histological feature of DOX is not the myocyte necrosis, but the loss of myofibrils and cytoplasmic vacuolization caused by dilatation of the sarcoplasmic reticulum in the myocardial cells (21), and there is a report that high dose of endothelin antagonist is needed to block endothelin induced hypertension in the dilated cardiomyopathy rat models (22). In addition, sustained pressure overload stimulate pathological cardiac hypertrophy and dysfunction (23) and hypertrophy progresses to decompensated phase with cardiac dilatation and contractile impairment during chronic pressure overload (24, 25). Some authors showed that the production of endothelin-1 increased in the hypertrophied left ventricle (26), but it depends on the time of the disease progress. Ventricular endothelin-1 levels and the density of endothelin-1 binding sites were increased significantly, without affecting their binding affinity, on day 8 of pressure overload (27), after then, the production of endothelin-1 is decreased and endothelin receptors are down-regulated in hypertrophied ventricles 8 weeks after aortic coarctation (28). However, it is also reported that endothelin-1 levels in subjects with heart failure in whom they are found to be elevated in the late, decompensated phase of the disease (29). This time-dependant up- and down-regulations of endothelin and endothelin receptors are also another proof for our hypothesis. That is, because of the differences of pathophysiology among our study group, cardiac endothelin-1 and endothelin receptors might not uniformly be induced in various heart failure models.
Second, in addition to endothelin, renin-angiotensin-aldosterone, epinephrine-norepinephrine, and natriuretic peptide have also been identified as neurohormones to be activated in cardiomyopathy (30). It is well known that doxorubicin administration has been shown to activate the renin-angiotensin system (31, 32) and the atrial natriuretic peptide level has also been increased relative to the severity of the cardiac dysfunction in human (32). And, there is a study on angiotensin and angiotensin receptor antagonist in the hypertrophic cardiomyopathy (33), showing that stimulation of the angiotensin receptor has an important role in disease process during pressure overload. Therefore, these neurohormones might be more important disease mediators in the DOX or TAC models than in the ICMP model.
There was a limitation to our study. Endothelin is a stimulator of polymorphonuclear leukocyte (PMN) aggregation (34) and adhesion as well as a potent vasoconstrictor. Myocardial ischemia initiates an acute inflammatory response in which PMNs are of importance (35). Because we had used isolated heart perfusion using Langendorff technique, however, most of the PMNs were diluted in the buffer perfused isolated heart. In other words, the effect of PMNs might be restricted and it can affect the results in our study.
In conclusion, our result reveals that tezosentan has myocardial protective effects for the ischemia-reperfusion injury in the ischemic cardiomyopathy model, but not in the doxorubicin-induced cardiomyopathy and pressure-overload hypertrophic heart failure models.
Figures and Tables
Fig. 1
(A) Experimental designs: there are three cardiomyopathy rat groups needed and each group was divided into three sub-groups. As mentioned previously, three groups, including ischemic dilated cardiomyopathy group (ICMP, n=30), doxorubicin-induced non-ischemic dilated cardiomyopathy group (DOX, n=30) and hypertrophic cardiomyopathy group by Transverse Aortic Constriction method (TAC, n=30) are prepared. After 1 month following constructions of cardiomyopathic rat groups, each group was then randomly assigned to a SHAM operation (SHAM; control group, n=10) subgroup or tezosentan treatment ischemia-reperfusion subgroup (Tezo, n=10) or tezosentan non-treatment ischemia-reperfusion subgroup (N-Tezo, n=10). (B) Ischemia-reperfusion injury experimental protocol (isolated heart perfusion via Langendorff technique): after the stabilization period of 20-30 min, total circulatory arrest for ischemia about 1 hr was performed. After then, reperfusion was performed during 2 hr. In Tezo subgroup, the tezosentan, each 10-5 M/L concentrations, is added to the cardioplegic solution and the perfusate.

Fig. 2
The results of cardiac performance. (A) LV developed pressure (LVDP) during ischemia-reperfusion injury. (B) dP/dTmax during ischemia-reperfusion injury. (C) dP/dTmin during ischemia-reperfusion injury. (D) Coronary blood flow (CBF) during ischemia-reperfusion injury.
*P<0.05 at 30 min of reperfusion among SHAM, Tezo and N-Tezo subgroups.
NS, there is no statistical significance among subgroups; ICMP, ischemic cardiomyopathy rat group; DOX, doxorubicin treated non-ischemic dilated cardiomyopathy rat group; TAC, hypertrophic cardiomyopathy rat group by transverse aortic constriction; SHAM, Sham operated ischemia-reperfusion subgroup; Tezo, tezosentan treated ischemia-reperfusion subgroup; N-Tezo, tezosentan non-treatment ischemia-reperfusion subgroup.
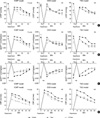
Fig. 3
(A) In the ICMP models, staining of triphenyltetrazolium chloride with evans blue was used for visualizing ischemia-infarct area of myocardium (black dash). (B) In the DOX models, dilated myocardium was confirmed with pathologic specimens (white arrow-enlarged left ventricle). (C) In the TAC models, hypertrophied myocardium was confirmed with pathologic specimens (white arrow).
*The scale of the ruler in the photo is millimeter (mm).
ICMP, ischemic cardiomyopathy rat group; DOX, doxorubicin treated non-ischemic dilated cardiomyopathy rat group; TAC, hypertrophic cardiomyopathy rat group by transverse aortic constriction.

Table 1
Variable hemodynamic parameters at the reperfusion 30 min

*Hemodynamic variables are significantly different among SHAM, Tezo and N-Tezo subgroups at 30 min of reperfusion (P<0.05).
ICMP, ischemic cardiomyopathy rat group; DOX, doxorubicin treated non-ischemic dilated cardiomyopathy rat group; TAC, hypertrophic cardiomyopathy rat group by transverse aortic constriction; SHAM, Sham operated ischemia-reperfusion subgroup; Tezo, tezosentan treated ischemia-reperfusion subgroup; N-Tezo, tezosentan non-treatment ischemia-reperfusion subgroup; LVDP, left ventricular developed pressure; CBF, coronary blood flow.
References
1. Parker JD, Thiessen JJ, Reilly R, Tong JH, Stewart DJ, Pandey AS. Human endothelin-1 clearance kinetics revealed by a radiotracer technique. J Pharmacol Exp Ther. 1999. 289:261–265.
2. Inada T, Fujiwara H, Hasegawa K, Araki M, Yamauchi-Kohno R, Yabana H, Fujiwara T, Tanaka M, Sasayama S. Upregulated expression of cardiac endothelin-1 participates in myocardial cell growth in Bio14.6 Syrian cardiomyopathic hamsters. J Am Coll Cardiol. 1999. 33:565–571.


3. Wittner M, Christ GJ, Huang H, Weiss LM, Hatcher VB, Morris SA, Orr GA, Berman JW, Zeballos GA, Douglas SA, Tanowitz HB. Trypanosoma cruzi induces endothelin release from endothelial cells. J Infect Dis. 1995. 171:493–497.


4. Cheng JW. Tezosentan in the management of decompensated heart failure. Cardiol Rev. 2005. 13:28–34.


5. Teerlink JR, McMurray JJ, Bourge RC, Cleland JG, Cotter G, Jondeau G, Krum H, Metra M, O'Connor CM, Parker JD, Torre-Amione G, Van Veldhuisen DJ, Frey A, Rainisio M, Kobrin I. Tezosentan in patients with acute heart failure: design of the Value of Endothelin Receptor Inhibition with Tezosentan in Acute heart failure Study (VERITAS). Am Heart J. 2005. 150:46–53.


6. Battistini B, Chailler P, D'Orleans-Juste P, Briere N, Sirois P. Growth regulatory properties of endothelins. Peptides. 1993. 14:385–399.


7. Kaddoura S, Firth JD, Boheler KR, Sugden PH, Poole-Wilson PA. Endothelin-1 is involved in norepinephrine-induced ventricular hypertrophy in vivo. Acute effects of bosentan, an orally active, mixed endothelin ETA and ETB receptor antagonist. Circulation. 1996. 93:2068–2079.
8. Teerlink JR. The development of new medical treatments for acute decompensated heart failure. Heart Fail Monit. 2002. 2:129–137.
9. Fishman WH, Kaur S, Singh I, Tamirisa P. Frishman WH, Sonnenblick EH, Sica DA, editors. Endothelin as a therapeutic target in the treatment of cardiovascular disease. Cardiovascular pharmacotherapeutics. 2003. 2nd ed. New York: McGraw-Hill, Medical Pub. Division;527–543.
10. Webb DJ. Evidence for endothelin-1-mediated vasoconstriction in severe chronic heart failure. Endothelin antagonism in heart failure. Circulation. 1995. 92:3372.
11. Margulies KB, Hildebrand FL Jr, Lerman A, Perrella MA, Burnett JC Jr. Increased endothelin in experimental heart failure. Circulation. 1990. 82:2226–2230.


12. Omland T, Lie RT, Aakvaag A, Aarsland T, Dickstein K. Plasma endothelin determination as a prognostic indicator of 1-year mortality after acute myocardial infarction. Circulation. 1994. 89:1573–1579.


13. Duchman SM, Thohan V, Kalra D, Torre-Amione G. Endothelin-1: a new target of therapeutic intervention for the treatment of heart failure. Curr Opin Cardiol. 2000. 15:136–140.


14. McMurdo L, Thiemermann C, Vane JR. The effects of the endothelin ETA receptor antagonist, FR 139317, on infarct size in a rabbit model of acute myocardial ischaemia and reperfusion. Br J Pharmacol. 1994. 112:75–80.


15. Richard V, Kaeffer N, Hogie M, Tron C, Blanc T, Thuillez C. Role of endogenous endothelin in myocardial and coronary endothelial injury after ischaemia and reperfusion in rats: studies with bosentan, a mixed ETA-ETB antagonist. Br J Pharmacol. 1994. 113:869–876.


16. Xia Z, Kuo KH, McNeill JH, Ansley DM. Endothelin A and B receptor antagonist bosentan reduces postischemic myocardial injury in the rat: critical timing of administration. Can J Physiol Pharmacol. 2005. 83:259–266.


17. Sakai S, Yorikane R, Miyauchi T, Sakurai T, Kasuya Y, Yamaguchi I, Sugishita Y, Goto K. Altered production of endothelin-1 in the hypertrophied rat heart. J Cardiovasc Pharmacol. 1995. 26:Suppl 3. S452–S455.


18. Cernacek P, Stewart DJ, Monge JC, Rouleau JL. The endothelin system and its role in acute myocardial infarction. Can J Physiol Pharmacol. 2003. 81:598–606.


19. Nguyen QT, Cernacek P, Calderoni A, Stewart DJ, Picard P, Sirois P, White M, Rouleau JL. Endothelin A receptor blockade causes adverse left ventricular remodeling but improves pulmonary artery pressure after infarction in the rat. Circulation. 1998. 98:2323–2330.


20. Nguyen QT, Cernacek P, Sirois MG, Calderone A, Lapointe N, Stewart DJ, Rouleau JL. Long-term effects of nonselective endothelin A and B receptor antagonism in postinfarction rat: importance of timing. Circulation. 2001. 104:2075–2081.
21. Arola OJ, Saraste A, Pulkki K, Kallajoki M, Parvinen M, Voipio-Pulkki LM. Acute doxorubicin cardiotoxicity involves cardiomyocyte apoptosis. Cancer Res. 2000. 60:1789–1792.
22. Watanabe K, Ohta Y, Kouda T, Sekiguchi T, Sato S, Nakazawa M, Hasegawa G, Naito M, Fuse K, Ito M, Hirono S, Tanabe N, Hanawa H, Kato K, Kodama M, Aizawa Y. Acute effects of endothelin-1 and TAK-044 (ET(A) and ET(B) receptor antagonist) in rats with dilated cardiomyopathy. J Cardiovasc Pharmacol. 2000. 36:Suppl 2. S49–S54.


23. Heineke J, Molkentin JD. Regulation of cardiac hypertrophy by intracellular signalling pathways. Nat Rev Mol Cell Biol. 2006. 7:589–600.


24. Houser SR, Margulies KB. Is depressed myocyte contractility centrally involved in heart failure? Circ Res. 2003. 92:350–358.


26. Yorikane R, Sakai S, Miyauchi T, Sakurai T, Sugishita Y, Goto K. Increased production of endothelin-1 in the hypertrophied rat heart due to pressure overload. FEBS Lett. 1993. 332:31–34.


27. Arai M, Yoguchi A, Iso T, Takahashi T, Imai S, Murata K, Suzuki T. Endothelin-1 and its binding sites are upregulated in pressure overload cardiac hypertrophy. Am J Physiol. 1995. 268:H2084–H2091.


28. Sirvio ML, Uhlenius N, Stewen P, Metsarinne K, Fyhrquist F. The effect of aortic coarctation on expression of endothelin-1 and endothelin receptors in heart and lungs. Blood Press. 1995. 4:320–323.
29. Wei CM, Lerman A, Rodeheffer RJ, McGregor CG, Brandt RR, Wright S, Heublein DM, Kao PC, Edwards WD, Burnett JC Jr. Endothelin in human congestive heart failure. Circulation. 1994. 89:1580–1586.


30. Hayashi M, Tsutamoto T, Wada A, Maeda K, Mabuchi N, Tsutsui T, Horie H, Ohnishi M, Kinoshita M. Intravenous atrial natriuretic peptide prevents left ventricular remodeling in patients with first anterior acute myocardial infarction. J Am Coll Cardiol. 2001. 37:1820–1826.


31. Arnolda L, McGrath B, Cocks M, Sumithran E, Johnston C. Adriamycin cardiomyopathy in the rabbit: an animal model of low output cardiac failure with activation of vasoconstrictor mechanisms. Cardiovasc Res. 1985. 19:378–382.


32. Bauch M, Ester A, Kimura B, Victorica BE, Kedar A, Phillips MI. Atrial natriuretic peptide as a marker for doxorubicin-induced cardiotoxic effects. Cancer. 1992. 69:1492–1497.


33. Lako-Futo Z, Szokodi I, Sarman B, Foldes G, Tokola H, Ilves M, Leskinen H, Vuolteenaho O, Skoumal R, deChatel R, Ruskoaho H, Toth M. Evidence for a functional role of angiotensin II type 2 receptor in the cardiac hypertrophic process in vivo in the rat heart. Circulation. 2003. 108:2414–2422.


34. Gomez-Garre D, Guerra M, Gonzalez E, Lopez-Farre A, Riesco A, Caramelo C, Escanero J, Egido J. Aggregation of human polymorphonuclear leukocytes by endothelin: role of platelet-activating factor. Eur J Pharmacol. 1992. 224:167–172.