Abstract
Dominant inflammatory cytokines might be different depending on the underlying causes of acute lung injury (ALI). The role of kertinocyte-derived chemokine (KC), a potent chemoattractant for neutrophils, has not been clearly established in hemorrhage-induced ALI. In this study, lung injury and cytokine expressison were evaluated in LPS- or hemorrhage-induced ALI models of BALB/c mice. The myeloperoxidase activities at 4 hr after hemorrhage and LPS-injection were 47.4±13.0 and 56.5±16.4 U/g, respectively. NF-κB activity peaked at 4 hr after hemorrhage, which was suppressed to the control level by anti-high mobility group B1 (HMGB1) antibody. Lung expressions of TNF-α, MIP-2, and IL-1β were increased by LPS injection. However, there was only a minimal increase in IL-1β and no expressions of TNF-α or MIP-2 in hemorrhage-induced ALI. In contrast, lung KC increased significantly at 4 hr after hemorrhage compared to control levels (83.1±12.3 vs. 14.2±1.6 pg/mL/mg by ELISA) (P<0.05). By immunohistochemical staining, lung neutrophils stained positive for KC. Increased KC was also observed in bronchoalveolar lavage fluid and plasma. KC plays an important role in hemorrhage-induced ALI.
Acute lung injury (ALI) is a clinical syndrome characterized by rapid-onset, bilateral pulmonary infiltrates and hypoxemia that are not attributed to left atrial hypertension (1, 2). While many medical and surgical conditions are associated with the development of ALI (3), most cases are caused by a relatively small number of clinical disorders (4). Sepsis is the most common cause leading to ALI/acute respiratory distress syndrome (ARDS). ARDS develops in almost half of sepsis patients, which usually occurs within 48 hr of the onset of sepsis (5). ALI is also frequently associated with trauma and blood loss with a loss of approximately 30% of the total blood volume is an important threshold for predicting the subsequent development of ALI and other organ system dysfunction (6).
Although ALI associated with blood loss may appear similar to sepsis-related ALI, there may be differences in the regulation of cytokines involved in the pathogenesis and progression of inflammatory process in each ALI type (7). These differences may lead to specific treatment that target specific agonists for each type of ALI.
Keratinocyte-derived chemokine (KC) is a mouse ortholog of human GRO-α, which is a potent chemoattractant for neutrophils (8, 9). KC expression is increased within the trachea and bronchoalveolar lavage fluid in lipopolysaccharide (LPS)-induced ALI (10). KC is also up-regulated after trauma or hemorrhage (11, 12). However, it's role and locations of expression in trauma/hemorrhage induced ALI have not been clearly delineated.
The objectives of this study are to compare cytokine expressions in the hemorrhage-induced ALI model with LPS-induced ALI in mice and to see what role KC has in this model of ALI.
Male BALB/c mice, 8-12 weeks of age, were purchased from Harlan Sprague-Dawley (Indianapolis, IN, U.S.A.). The mice were kept on a 12 hr light/dark cycle with free access to food and water. All experiments were conducted in accordance with institutional review board-approved protocol.
Bicinchoninic acid (BCA) protein assay reagent was purchased from Pierce (Rockford, IL, U.S.A.). Chicken polyclonal antibody to human high mobility group B1 (HMGB1) and chicken control IgY antibody were kindly donated by Dr. Akitoshi Ishizaka (Keio University, Tokyo, Japan). All other chemicals were obtained from Sigma (St. Louis, MO, U.S.A.).
Mice received an intra-peritoneal injection of LPS (E. coli 0111:B4) at a dose of 5 mg/kg in 0.2 mL phosphate-buffered saline (PBS). Mice were anesthetized 4 hr after LPS injection and chest was opened and flushed by infusing 10 mL of PBS into beating right ventricle. Then, lungs were removed and stored at -70℃ before being used for cytokines measurement and MPO assay.
Mice were anesthetized with inhaled isoflurane (Abbott Lab., Chicago, IL, U.S.A.). Hemorrhagic shock was induced by removing 30% of the calculated total blood volume (0.27 mL/10 g body weight) over 60 sec through cardiac puncture. Aspirated blood was re-infused into retro-orbital vein 1 hr later. The sham procedure involved cardiac puncture under isoflurane anesthesia, but no blood was removed.
Neutralizing chicken anti-human HMGB1 antibody (200 µg/mouse) was injected into the peritoneum 1 hr after the induction of hemorrhagic shock (immediately following the infusion of the aspirated blood). The therapeutic effects of the antibody against NF-κB activation was evaluated at 4 hr after the induction of hemorrhage by electrophoretic mobility shift assay (EMSA).
Lung tissues were homogenized in ice cold lysis buffer (50 mM HEPES, 150 mM NaCl, 10% glycerol, 1% Triton X-100, 1.5 mM MgCl2, 1 mM EGTA, 1 mM sodium vanadate, 10 mM sodium pyrophosphate, 10 mM NaF, 300 µM P-nitrophenyl phosphate, 1 mM PMSF, 10 µg/mL leupeptin, 10 µg/mL aprotinin, pH 7.3) containing 1 mM of protease inhibitor. Homogenates were centrifuged at 14,000 g for 15 min, and supernatants were collected.
Immunoreactive TNF-α, IL-1β, MIP-2 and KC were quantified in duplication using commercially available ELISA kits (R&D Systems, Minneapolis, MN, U.S.A.), according to the manufacturer's instruction. ELISA for HMGB1 in the plasma was performed with the use of monoclonal antibody to human HMGB1 antibody.
Lung tissue was homogenized in 1.0 mL of 50 mM potassium phosphate buffer (pH 6.0) containing a reducing agent, N-ethylmaleimide (10 mM) for 30 sec on ice. The homogenate was centrifuged at 12,000 g for 30 min at 4℃. The pellet was resuspended and sonicated on ice for 90 sec in 10 times volume of hexadecyltrimethylammonium bromide (HTAB) buffer (0.5% HTAB in 50 mM potassium phosphate, pH 6.0). Samples were incubated in a water bath (56℃) for 2 hr and then centrifuged at 12,000 g for 10 min. The supernatant was collected for assay of MPO activity as determined by measuring the H2O2-dependent oxidation of o-DA (3,3'-dimethoxybenzidine dihydrochloride) at 460 nm (12).
Lungs were homogenized in buffer A containing 1 mM DTT and 1 mM protease inhibitor. After storing homogenates on ice for 15 min, 10% Igepal CA630 solution was added to a final concentration of 0.6%. Then homogenates were centrifuged immediately at 4℃ for 1 min at 8,000 g. After removing the supernatant, the nuclear pellet was resuspended in 75 µL of extraction buffer (buffer C) containing 1 mM DTT and 1 mM protease inhibitor cocktail. The extract was centrifuged at 4℃ for 15 min at 18,000 g. The supernatant was collected and stored at -80℃ (12).
Nuclear extracts were prepared and assayed by EMSA as followings. For analysis of NF-κB, the B-DNA sequence of the Ig gene was used. Synthetic double-stranded sequences (with enhancer motifs underlined) were filled in and labeled with [-32P]dATP using Sequenase DNA polymerase as follows: B, 5'-TTTTCGAGCTCGGGA CTTTCCGAGC-3' and 3'-GCTCGAGCCCTGAAAGGCTCGTTTT-5'(Promega, Madison, WI, U.S.A.). Nuclear extracts (10 µg) were added to radiolabeled NF-κB oligonucleotide in binding buffer. DNA-protein complexes were resolved on 5% nondenaturing polyacrylamide gel in Tris-HCl (pH 7.5)-borate-EDTA buffer at 10 V/cm. Dried gels were exposed with Kodak Biomax MS film (Rochester, NY, U.S.A.) for 1-24 hr at -70℃. Quantification was performed by image analysis using densitometry (ChemDoc system, Bio-Rad, Hercules, CA, U.S.A.).
To evaluate the expression of cytokines in the bronchoalveolar lavage (BAL) fluid, the trachea was exposed and the lungs were lavaged three times with 1 mL of sterile saline per wash. The aliquots of BAL fluid were pooled and stored at -80℃ before use.
Each slice of lung (4-5 µm thickness) was fixed by acetone for 5 min. After then, it was washed with 50 mM Tris buffer solution (TBS, pH 7.5). Rabbit polyclonal GRO alpha (1:50, Abcam, Cambridge, U.K.) was used as a primary antibody and biotinated, anti-rabbit antibody (Zymed, South San Francisco, CA, U.S.A.) was applied as a secondary antibody.
Lung neutrophil accumulation peaked 4 hr after hemorrhage, and then returned to baseline levels within 48 hr after bleeding. MPO activities at 0, 4, 24, 48, and 72 hr after hemorrhage were 15.9±2.2, 47.4±13.0 (P<0.05 compared to control), 28.0±1.1, 36.5±3.8 and 34.7±2.0 U/g of lung protein, respectively (Fig. 1). MPO activity at 4 hr after intraperitoneal injection of LPS was 56.5±16.4 U/g of lung protein (Fig. 1).
Increased expression of nuclear NF-κB was noted in the lungs of mice after hemorrhage, which was treated by control chicken IgY antibody. However, in mice treated with anti-HMGB1 antibody, NF-κB activity in the lung was suppressed almost to the same levels as in control mice without hemorrhage (Fig. 2).
The mean expression of TNF-α in the lungs of control mice was 84±18 pg/mL/mg of lung protein. This increased to 192±11 pg/mL/mg of lung protein (P<0.05) at 4 hr after LPS injection (5 mg/kg). MIP-2 activities in the lungs of control and LPS-injected mice were 187±26 and 3,000±725 pg/mL/mg of lung protein (P<0.05), respectively. The expressions of IL-1β in the lungs of control and LPS-injected mice was 266±25 and 6,500±1,167 pg/mL/mg of lung protein (P<0.05), respectively (Fig. 3).
The expression of TNF-α in the lung at 0, 4, 24, 48, and 72 hr after hemorrhage were 13.5±0.9, 10.9±1.7, 10.0±1.0, 8±1.3, and 12.1±2.4 pg/mL/mg of lung protein (P>0.05), respectively. Lung expressions of MIP-2 at these time points were 33.6±4.0, 30.1±3.7, 25.5±1.9, 26.3±5.9, and 30.2±11.4 pg/mL/mg of lung protein (P>0.05), respectively. IL-1β, expressions were 32.0±11.0, 106.0±7.0 (P<0.05 compared to control), 35.2±1.4, 28.2±5.3, and 29.3±3.4 pg/mL/mg of lung protein (Fig. 4).
Lung activities for KC at 0, 4, 24, 48, and 72 hr after bleeding were 14.2±1.6, 86.5±23.9 (P<0.05), 26.3±4.7, 22.9±3.4, and 28.0±3.2 pg/mL/mg of lung protein, respectively (Fig. 5).
Using polyclonal rabbit anti-GROα/KC antibody, immunohistochemical staining was done for lung tissue. Compared to control mice, increased infiltrations of neutrophils were found in the lungs of mice at 4 hr after hemorrhage, many of which stained positive for anti-GROα/KC antibody (Fig. 6).
Mice were sacrificed at 4 or 24 hr after hemorrhage and bronchoalveolar lavage (BAL) was done. The mean expression of KC in BAL fluid of control mice was 5.3±3.1 pg/mL. This increased to 26.3±8.6 pg/mL (P<0.05) and 25.5±4.8 pg/mL (P<0.05) at 4 and 24 hr after hemorrhage, respectively (Fig. 7).
At 0, 4, 24, 48, and 72 hr after hemorrhage, blood was sampled by cardiac puncture. After harvesting plasma, KC was measured by ELISA. The plasma concentration of KC at these time points were 73±38, 606±176 (P<0.05), 63±22, 105±16, and 81±25 pg/mL, respectively (Fig. 8).
Among different animal ALI models, a LPS-induced septic model was adopted as the model for sepsis-induced ALI in the present study. Neutrophil recruitment and cytokine expressions in the lungs were measured only at 4 hr after LPS injection as in the previous studies (7, 13). A hemorrhage-reperfusion model was introduced to reproduce trauma-induced ALI. Because time-response profiles are rarely reported for hemorrhage models, physiologic data were recorded at 4, 24, 48, and 72 hr after hemorrhage.
Activated neutrophils cross over rapidly to the lung in acute lung injury, which appears to be a ubiquitous phenomenon regardless of ALI types. Accumulations of neutrophils in the lungs were observed as early as 1 hr after hemorrhage in a previous study (7). In the present study, large numbers of neutrophils, as demonstrated by pulmonary MPO levels, accumulated in the lungs of ALI mice, which peaked at 4 hr after hemorrhage (Fig. 1). Of note, the MPO level at 4 hr after hemorrhage (47.4±13.0 U/g of lung protein) was almost as high as the level after LPS stimulation (56.5±16.4 U/g of lung protein). This implies that hemorrhage could be as strong a stimulus as LPS injection (5 mg/kg) for attracting neutrophils into the lung.
It is interesting to note that similar to LPS stimulation (14-16), hemorrhage induced activation of a transcriptional factor, NF-κB for intracellular signaling pathways. In addition, hemorrhage-induced activation of NF-κB was suppressed almost to the same levels as in control mice when anti-HMGB1 antibody was administered 1 hr after hemorrhage.
HMGB1 protein, originally identified as a DNA binding protein. has potent proinflammatory properties (17). Administration of HMGB1 to normal animals causes inflammatory responses, including fever, weight loss and anorexia, acute lung injury, epithelial barrier dysfunction, arthritis, and death (18). Treatment with anti-HMGB1 antibodies for mice exposed to intratracheal LPS significantly decreased lung edema and neutrophil accumulation in the lung (19). Elevated, circulating levels of HMGB1 have also been described in human hemorrhagic shock without evidence of infection (20). In the present study, we have chosen anti-HMGB1 as a suppressor for NF-κB activation because it is one of rare antagonists active against both hemorrhage- and LPS-induced translocation of this ubiquitous transcriptional factor.
As expected, hemorrhage-induced lung injury is regulated by a ubiquitous transcriptional factor, NF-κB and is suppressed by anti-HMGB1 treatment. However, lung inflammatory cytokines were not similar between two ALI models. LPS stimulation induced high expression of TNF-α, MIP-2, and IL-1β (Fig. 3) in the mice lungs.
Because KC has been reported to play an important role in ALI induced by hemorrhage/cecal ligation and puncture (CLP), we decided to evaluate its expression in our animal model, which simulates pure form of hemorrhage-induced ALI (21). The lung KC level, which was evaluated by ELISA, increased more than 9-fold at 4 hr after hemorrhage compared to the control mice (Fig. 5). By immunohistochemical staining with anti-GRO alpha/KC antibody, neutrophil infiltration was detected in the lungs at 4 hr after hemorrhage and the infiltrated neutrophils stained positive for KC (Fig. 6). Overall, KC appears to play an important role in hemorrhage-induced ALI.
In this study, KC expression was increased not only in lung tissue but also at other organs of experimental hemorrhage mice. In the bronchoalveolar lavage fluid of experimental mice, KC expression was increased compared to control mice (Fig. 7). Expression of KC was increased at 4 and 24 hr after the hemorrhage. Although increased expression of KC in BAL fluid has been reported in LPS-induced ALI, this is the first report to find it in hemorrhage-induced ALI.
The high level of KC continued up to 24 hr in bronchoalveolar lavage fluid, while KC expression in the lung peaked at 4 hr and then returned to the control level by 24 hr. This can be explained by the closed space effect of the bronchoalveolar tree, which may have prevented washing out of KC by the circulation. Of note, plasma KC increased at 4 hr after hemorrhage, and then returned to the control level at 24 hr (Fig. 8). It is evident that hemorrhage induces not only lung injury but also systemic inflammation.
KC is increased not only in hemorrhage-induced ALI, but also tissues of LPS-induced ALI. Because KC seems more important in hemorrhage-induced ALI than other cytokines, KC could be a target when an antagonist is to be developed against lung inflammation after blood loss.
Our study has several limitations. We showed that expressions of KC are increased in the lung, bronchoalveolar lavage fluid and plasma after hemorrhage. However, it will be necessary to perform studies using anti-KC antibody or KC knockout mice to substantiate a dominant role for KC in hemorrhage-induced ALI. In addition, the mechanisms for the discrepancy in lung inflammatory cytokines between LPS- and hemorrhage-induced ALI have not been evaluated. These differences must be elaborated in the future.
In summary, hemorrhage resulted in neutrophil infiltration, and increased activity of NF-κB, which was suppressed by anti-HMGB1 treatment. Enhanced expressions of KC were found in bronchoalveolar lavage fluid and plasma as well as in lung tissue in hemorrhage-induced ALI. KC plays an important role in hemorrhage-induced ALI.
Figures and Tables
Fig. 1
Lung neutrophil accumulation as assessed by MPO activity after hemorrhage- or endotoxemia-induced acute lung injury. Neutrophil accumulation in the lung was evaluated with MPO assay at 0, 4 hr, 24 hr, 48 hr and 72 hr after hemorrhage and 4 hr after lipopolysaccharide (LPS) injection (5 mg/kg, i.p.). Data are mean±SEM of at least 5 mice per group. *P<0.05.

Fig. 2
Anti-HMGB1 antibody treatment decreases the NF-κB activation in the lung. One hour after the induction of hemorrhage, mice were injected with either control chicken IgY antibody (Hem+Cont Ab, 200 µg/mice) or anti-HMGB1 antibody (Hem+anti-HMGB1, 200 µg/mice). Four hours after the induction of hemorrhage, mice were sacrificed and NF-κB activity was determined in the lung with EMSA. The data shown are representative of three independent experiments.

Fig. 3
LPS induces an increase in the expression of TNF-α, MIP-2 and IL-1β in the lung. The expressions of TNF-α, MIP-2 and IL-1β of lung tissue were determined by ELISA at 4 hr after injection of LPS (5 mg/kg, i.p.). Data are mean±SEM of at least 5 mice per group. *P<0.05.

Fig. 4
Hemorrhage induces minimal increase of IL-1β and no increase of TNF-α and MIP-2 expression in the lung. The expressions of TNF-α, MIP-2 and IL-1β of lung tissue were determined by ELISA at 0, 4 hr, 24 hr, 48 hr, and 72 hr after hemorrhage. Data are mean±SEM of at least 5 mice per group. *P<0.05.

Fig. 5
Hemorrhage induces an increase in the expression of KC in the lung. The expression of KC of lung tissue was determined by ELISA at 0, 4 hr, 24 hr, 48 hr, and 72 hr after hemorrhage. Data are mean±SEM of at least 5 mice per group. *P<0.05.
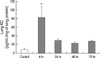
Fig. 6
Increased expression of KC in the lung at 4 hr after hemorrhage was confirmed by immunohistochemical staining. The expression of KC of lung tissue was determined by immunohistochemical (IHC) staining at 4 hr after hemorrhage. Rabbit polyclonal GRO alpha (1:50, Abcam, Cambridge, U.K.) was used as a primary antibody. Biotinated, anti-rabbit antibody (1:300, Zymed, South San Francisco, CA, U.S.A.) was applied as a secondary antibody. For background staining, 3-amino-9-ethyl carbazole (AEC) was used (×400).
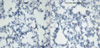
References
1. Bernard GR, Artigas A, Brigham KL, Carlet J, Falke K, Hudson L, Lamy M, Legall JR, Morris A, Spragg R. The American-European consensus conference on ARDS. Definition, mechanisms, relevant outcomes, and clinical trial coordination. Am J Respir Crit Care Med. 1994. 149:818–824.
2. Ashbaugh DG, Bigelow DB, Petty TL, Levine BE. Acute respiratory distress in adults. Lancet. 1967. 2:319–323.


3. Rubenfeld GD, Caldwell E, Peabody E, Weaver J, Martin DP, Neff M, Stern EJ, Hudson LD. Incidence and outcomes of acute lung injury. N Engl J Med. 2005. 353:1685–1693.


5. Sevransky JE, Levy MM, Marini JJ. Mechanical ventilation in sepsis-induced acute lung injury/acute respiratory distress syndrome: an evidence-based review. Crit Care Med. 2004. 32(11):Suppl. S548–S553.


6. Hudson LD, Milberg JA, Anardi D, Maunder RJ. Clinical risks for development of the acute respiratory distress syndrome. Am J Respir Crit Care Med. 1995. 151:293–301.


7. Abraham E, Carmody A, Shenkar R, Arcaroli J. Neutrophils as early immunologic effectors in hemorrhage- or endotoxemia-induced acute lung injury. Am J Physiol Lung Cell Mol Physiol. 2000. 279:L1137–L1145.


8. Molls RR, Savransky V, Liu M, Bevans S, Mehta T, Tuder RM, King LS, Rabb H. Keratinocyte-derived chemokine is an early biomarker of ischemic acute kidney injury. Am J Physiol Renal Physiol. 2006. 290:1187–1193.


9. Schober A. Chemokines in vascular dysfunction and remodeling. Arterioscler Thromb Vasc Biol. 2008. 28:1950–1959.


10. Huang S, Paulauskis JD, Godleski JJ, Kobzik L. Expression of macrophage inflammatory protein-2 and KC mRNA in pulmonary inflammation. Am J Pathol. 1992. 141:981–988.
11. Frink M, Hsieh YC, Hsieh CH, Pape HC, Choudhry MA, Schwacha MG, Chaudry IH. Keratinocyte-derived chemokine plays a critical role in the induction of systemic inflammation and tissue damage after trauma-hemorrhage. Shock. 2007. 28:576–581.


12. Kim JY, Park JS, Strassheim D, Douglas I, Diaz del Valle F, Asehnoune K, Mitra S, Kwak SH, Yamada S, Maruyama I, Ishizaka A, Abraham E. HMGB1 contributes to the development of acute lung injury after hemorrhage. Am J Physiol Lung Cell Mol Physiol. 2005. 288:L958–L965.


13. Choi JC, Jung JW, Kwak HW, Song JH, Jeon EJ, Shin JW, Park IW, Choi BW, Kim JY. Granulocyte macrophage-colony stimulating factor (GM-CSF) augments acute lung injury via its neutrophil priming effects. J Korean Med Sci. 2008. 23:288–295.


14. Schell MT, Spitzer AL, Johnson JA, Lee D, Harris HW. Heat shock inhibits NF-kB activation in a dose- and time-dependent manner. J Surg Res. 2005. 129:90–93.


15. Barsness KA, Arcaroli J, Harken AH, Abraham E, Banerjee A, Reznikov L, McIntyre RC. Hemorrhage-induced acute lung injury is TLR-4 dependent. Am J Physiol Regul Integr Comp Physiol. 2004. 287:R592–R599.


16. Brundage SI, Schreiber MA, Holcomb JB, Zautke N, Mastrangelo MA, Xq X, Macaitis J, Tweardy DJ. Amplification of the proinflammatory transcription factor cascade increases with severity of uncontrolled hemorrhage in swine. J Surg Res. 2003. 113:74–80.


17. Andersson U, Erlandsson-Harris H, Yang H, Tracey KJ. HMGB1 as a DNA-binding cytokine. J Leukoc Biol. 2002. 72:1084–1091.
18. Yang H, Wang H, Czura CJ, Tracey KJ. The cytokine activity of HMGB1. J Leukoc Biol. 2005. 78:1–8.
19. Abraham E, Arcaroli J, Carmody A, Wang H, Tracey KJ. HMG-1 as a mediator of acute lung inflammation. J Immunol. 2000. 165:2950–2954.
20. Ombrellino M, Wang H, Ajemian MS, Talhouk A, Scher IA, Friedman SG, Tracey KJ. Increased serum concentration of high-mobility-group protein 1 in hemorrhagic shock. Lancet. 1999. 354:1446–1447.