Abstract
The incidence of diastolic heart failure increases dramatically with age. We investigated the impact of long-term exercise training on age-related diastolic dysfunction. Old (25-month-old) male Fischer 344 rats were studied after 12 weeks of treadmill exercise training or sedentary cage life (N=7, in each group). We determined cardiac performance using a pressure-volume conductance catheter and magnetic resonance imaging. Collagen volume fraction (CVF) and myocardial collagen solubility by pepsin as an index of advanced glycation end products (AGEs) cross-linked collagen were measured. The maximal slope of systolic pressure increment (+dP/dt) and the slope of end-systolic pressure-volume relation were higher, and end diastolic volume (EDV), ΔEDV (the percentage of the EDV increment-to-baseline EDV) and the slope of end-diastolic pressure-volume relation were lower in training group. The maximal slope of diastolic pressure decrement (-dP/dt) and time constant of LV pressure decay (τ) had no difference. AGEs cross-linked collagen, not CVF was reduced by exercise training. Long-term exercise training appears to attenuate age-related deterioration in cardiac systolic function and myocardial stiffness and could be reduce in pathologic AGEs cross-linked collagen in myocardium.
The incidence of diastolic dysfunction is age-related, and the incidence of diastolic heart failure increases with age (1, 2). The normal aging process itself is known to depress cardiac performance during diastole which happens earlier than events in systole. One of the pathology of diastolic dysfunction with age is increase in myocardial stiffness resulting from changes in ventricular passive diastolic properties (3, 4).
Fibrillar collagen is a component of the extracellular matrix (ECM), which is postulated to be a determinant of myocardial stiffness. Furthermore, some have suggested that collagen cross-linkings, rather than total collagen or types of collagen, are even more important at determining chamber stiffness in the heart (5, 6). Collagen cross-linking may occur via two different pathways. One involves an enzymatically controlled cross-linking which occurs during development and physiologic maturation, and the other is a non-enzymatic form of cross-linking that occurs as a result of the spontaneous modification of proteins by glucose to form advanced glycation end products (AGEs) (7). This latter process follows tissue maturation and is the major cause of collagen tissue dysfunction during senescence (8). Other studies have shown that AGEs cross-link breakers improve cardiac diastolic function in aged animals and humans (6, 9).
Existing cross-sectional studies and limited training trials support the hypothesis that exercise may improve diastolic function (10, 11). It is uncertain whether the decline in diastolic function that occurs during aging is a feature of physiologic process or a pathologic state attributable of a sedentary life style in the aged population, and thus one reversible by exercise training. Moreover, the influences of long term exercise training on the AGEs cross-linked collagen and cardiac performance have not been tested.
Thus this study was designed to test whether exercise prevents the age-associated changes in non-enzymatic cross-linking of myocardial collagen, and thus improves cardiac performance in an animal model.
Young and old male Fischer 344 pathogen-free rats (Samtako Bio, Osan, Korea) were housed in a climate-controlled room (25℃, 12-hr light-dark cycle) and allowed standard food and water ad libitum. Old rats were weight-matched and randomly assigned to a sedentary control (OC) or an exercise-training group (OT). Young rats (YC) were assigned to a control group only to determine aging effects. The number of rats in each group was at least 7. After either 12 weeks of exercise or alternatively confinement in a cage, rats (young: 6 months and old: 25 months of age) were studied. The 6-month-old rats are mature animals which had passed the rapid growth phase and 25-month-old rats represent old animals with relatively little mortality (12).
Plasma glucose levels were assessed at baseline using the glucose oxidase technique (YSI 2300 stat plus glucose/L-lactate analyzer; YSI, Yellow Springs, OH, U.S.A.). This investigation conducted in accord with the guidelines issued by the Seoul National University Hospital Institutional Animal Care and Use Committee.
Before training, all rats were familiarized with a treadmill (1004RVI Exer-4R Open Treadmill; Columbus Instruments, Columbus, OH, U.S.A.) by walking or running for 10 min/day for 10-15 days. Animals in OT commenced training at 4 m/min, for 20-30 min, 5 days/week. Rates and times were progressively increased throughout the 12-week training program. Rates at termination were 15 m/min for 45-60 min/day, 5 days/week. This speed-grade combination has been shown to be slightly less than 70-75% of maximal oxygen consumption (VO2max) (11, 13). The YC and OC rats walked or ran on the treadmill for 10 min once a week to maintain familiarity with the treadmill for subsequent maximal exercise capacity testing. At the end of the 12-week period, maximal exercise capacities were measured twice in each rat, using a test involving walking at 10 m/min for 5 min followed by 2 m/min increases in speed every 2 min until the animal was exhausted (9).
Cardiac magnetic resonance imaging (MRI) was performed at baseline and 2 days after measuring maximal exercise capacity. Rats were anesthetized with pentobarbital sodium (Entobar; Hanlim; young rats 50 mg/kg, old rats 42.5 mg/kg) administered intraperitoneally (i.p.).
Rats were imaged with a 1.5-T clinical imaging unit (Gyroscan Intera, release 10; Philips Medical Systems, Best, Netherlands) equipped with a custom surface coil (4.7 cm in diameter). A prospectively electrocardiogram (ECG)-gated steady-state free-precession cine sequence (balanced fast field-echo 10/4.1 [repetition time msec/echo time msec], flip angle of 35°, matrix of 179×256, field of view of 80×80 mm, number of excitation:1) was applied in a true short-axis image orientation to encompass the entire heart with contiguous 2 mm sections. With ECG referencing, the temporal resolution was 10 time frames per cardiac cycle per study. A shimming procedure was performed for imaging areas to avoid magnetic field inhomogeneity. Most MR images were suitable for analysis.
MR images were analyzed by two independent readers, blinded to subjects and their results, using commercially available software on an off-line workstation (View Forum, release 3.2, Cardiac Package; Philips Medical Systems). The cine loops were viewed and end-diastolic and end-systolic frames were chosen as the images with the largest and smallest left ventricle (LV) cavities, respectively. Endocardial contours, including papillary muscles leading into the LV cavity, were traced using a semiautomated border technique. LV volumes were calculated consecutively from MR imaging data sets with software assistance.
Hemodynamic parameters were measured as previously described (14). Two days after MRI measurements, rats were anesthetized with pentobarbital sodium (young rats 50 mg/kg, old rats 42.5 mg/kg, i.p.) without endotracheal intubation while breathing room air. MPVS-300 Cardiovascular Micro-Tip multi-segment pressure-volume (P-V) transducer catheter (Millar Instruments, Huston, TX, U.S.A.) was inserted into the right carotid artery and advanced into the LV under pressure monitoring. Catheter was inserted into the right femoral artery for mean arterial pressure (MAP) measurement and into the right femoral vein for fluid administration. Heart rate (HR), maximal LV systolic pressure (LVSP), LV end-diastolic pressure (LVEDP), MAP, maximal slope of systolic pressure increment (+dP/dt) and diastolic pressure decrement (-dP/dt), time constant of LV pressure decay (tau, τ), ejection fraction (EF), stroke volume (SV), LV end-diastolic volume (EDV), cardiac output (CO), and stroke work (SW) were computed using a cardiac P-V analysis program (PVAN3.3; Millar Instruments). Isovolumic relaxation was quantified by calculating -dP/dt and τ. Tau (τ) describes the rate of fall of LV pressure during isovolumic relaxation. The area contained within the left ventricular pressure-volume loop is the SW. SV and CO were calculated and corrected according to in vitro and in vivo volume calibrations using PVAN3.3. CO and SW were normalized to body weight (cardiac index [CI] and SW index [SWI]).
LV pressure-volume relations were assessed by transiently compressing the inferior vena cava. The slope of end-systolic and end-diastolic pressure-volume relation (ESPVR and EDPVR) and +dP/dt-EDV relation were calculated using PVAN 3.3. ESPVR and +dP/dt-EDV represents a parameter of chamber contractility. EDPVR slope is an index of LV chamber stiffness. The volume calibration of the conductance system was performed. At the end of each experiment, 50 µL of 15% saline was injected intravenously, and from shifts in P-V relations parallel conductance volume (Vp) was calculated using PVAN 3.3 and used to correct cardiac mass volume.
After the hemodynamic measurements, animals were sacrificed by exsanguinations (aortas were punctured to obtain blood samples). Hearts and lungs were removed, and left and right ventricles were dissected and weighed. Hearts were snapfrozen in liquid nitrogen and stored at -80℃ for hydroxyproline assay or were fixed in 10% formalin and paraffin-embedded for histological assessment.
Interstitial collagen in LV mid-free wall was measured using Picrosirius red staining with a computerizing automated image analysis system (Image-pro plus, Silver Spring, MD, U.S.A.) (5). Collagen volume fraction (CVF) was defined as the sum of all stained interstitial collagen tissue areas divided by the whole tissue area. Collagen surrounding intramyocardial coronary arteries was excluded.
Hydroxyproline (a marker of collagen content) concentrations were determined using the colorimetric assay described by Woessner (15). Collagen concentrations were calculated by assuming that collagen weighs 7.25 times more that of hydroxyproline (collagen has a molecular weight of 300,000).
Unmodified heart collagen was significantly solubilized by pepsin after 2 hr and greater than 98% of heart collagen was solubilized after 24 hr. The susceptibility of collagen to digestion by pepsin provides an index of AGEs cross-linked collagen (16, 17). Aliquots of LV were excised and mixed with 3 mL of 200 µg/mL pepsin in 0.5 M/L acetic acid at 37℃. After 2 and 24 hr of digestion, 1 mL of the supernatant was removed and hydroxyproline concentrations were measured as described above.
The results presented reflect means±SE per group. Interobserver agreement of the MRI results was determined by the Pearson correlation coefficient. An unpaired two-sided Student's t-test was used to compare old vs. young rats, and old controls vs. old trained groups. p values <0.05 were considered statistically significant, and SPSS Ver 12.0 software was used throughout (SPSS Inc., Chicago, IL, U.S.A.).
At baseline, there were no significant differences in body weight (OC 409±8 g, OT 410±9 g; p=0.877) and plasma glucose levels (OC 150±10 mg/dL, OT 157±12 mg/dL; p=0.564) in old rats. At the end of the 12-week period, LV weight and the ratio of LV to body weight were significantly higher in OC rats compared with YC rats without notable differences in body weight (aging effect). In addition, wet lung weights to body weight, an index of pulmonary congestion followed a similar pattern, indicating marked pulmonary congestion in old rats secondary to cardiac decompensation. Exercise-training significantly reduced body weight in OT rats. LV weight and the ratio of LV weight to body weight (an index of LV hypertrophy) were not different between OC and OT groups after 12 week of exercise training. Exercise training had no effect on LV weight, the ratio of LV to body weight, or the ratio of lung to body weight in old rats (Table 1).
Maximal exercise capacity was measured twice with high reproducibility (r=0.823 [p<0.001], and r2=0.677 by Pearson's rank-order correlation analysis). Twelve weeks of exercise training significantly increased maximal exercise capacity, defined as time to exhaustion using a standardized treadmill running protocol, as was expected (7.7±1.0 min in OC, 20.8±1.7 min in OT; p<0.001). Exercise training generated the OT rats with a maximal exercise capacity matched that of the YC rats (17.2±1.6 min in YC, 20.8±1.7 min in OT; p>0.05).
Typical P-V loops obtained from young and old animals shows in Fig. 1. The shift of P-V loops to the right and decreased +dP/dt in aging rats indicated a decrease in cardiac contractility. Aging was associated with significant decrease in HR, MAP, LVSP, +dP/dt, -dP/dt. In contrast, LVEDP, EDV, ESV, τ(a classic parameter of active relaxation), and EDPVR slope (a parameter of LV chamber stiffness) significantly increased in aging, indicating diastolic dysfunction (Table 2). In old rats, +dP/dt was significantly decreased but EF, SV, CI, and SWI were not different from the parameters of young rats, which resembles findings in diastolic heart failure in men. During preload manipulation, ESPVR slope was steeper in young than in old rats, suggesting decreased systolic performance with aging. In contrast, EDPVR slope was increased (with a higher EDPVR slope) in aging rats, indicating increased end-diastolic stiffness. ESPVR slope and +dP/dt were significantly increased in OT vs. OC rats. Fig. 2 shows that the slope of +dP/dt-EDV relation and SW and EDV were steeper in OT vs. OC rats, indicating increased contractility in OT rats. ESPVR was a load insensitive index of contractility but also by changes in chamber geometry and other diastolic factors. +dP/dt is a classic parameter that is sensitive to changes in contractility but dependent on changes in preload. +dP/dt-EDV represents a sensitive but less load-dependent parameter of chamber contractility. Preload recruitable SW (PRSW), the slope of the relation between SW and EDV, is sensitive to chamber contractile function, which is independent of chamber size and mass.
Exercise was also associated with significant decreases in EDV and EDPVR slope. Tau (τ) and -dP/dt were not different between OC and OT rats (Table 2). The long-term exercise training prominently improved myocardial contractility and stiffness.
At the baseline ESV and EDV measured by MRI were not different among OC and OT rats. After 12 weeks of exercise training, ESV and EDV increased in old rats, but, post-ESV and EDV had no difference in OC and OT groups. However, ΔEDV (%), the percentage of the EDV increment-to-baseline EDV in each rats was 50.5±12.7% in OC rats and 20.2±7.6% in OT rats (p=0.060) (Table 3). Interobserver measurement of MR volumes were closely correlated (R, 0.96, 95% CI, 0.55 to 1.00, p<0.05).
Total myocardial collagen amount (% dry weight) and CVF were significantly higher in OC vs. YC rats (percent collagen: 6.67±0.91% vs. 2.08±0.79%, respectively; p=0.013). Exercise training did not influence total myocardial collagen amount of LV in the old rats (OC 6.67±0.91%, OT 6.61±0.61%; p=0.962) (Fig. 3). Myocardial collagen solubility as an index of AGEs cross-linked collagen was significantly lower in old rats (YC 43.57±1.13%, OC 38.11±1.27%; p=0.006), but significantly increased in OT rats (OC 38.11±1.27%, OT 43.94±2.11%; p=0.032) (Fig. 4).
The cause and pathology diastolic dysfunction with age is largely unknown, but it is most likely that age-related decrease in the rate of LV relaxation results from changes in the passive diastolic stiffness of LV (3, 4).
We investigated the impact of long-term exercise training on age-related diastolic dysfunction. In this study, exercise attenuated the age-associated diastolic deterioration in myocardial stiffness and was related to changes in the biochemical characteristics of collagen in myocardium. AGEs cross-linked collagen in LV was lower in old rats in exercise-training group than in control group.
The normal aging process in human and other long-lived species is characterized by the gradual decrease in the elasticity of the cardiovascular system. These structural and mechanical changes in the heart lead to increased myocardial stiffness, and consequently to diastolic dysfunction, a significant contributing factor in heart failure in human. In vivo, chamber stiffness can be quantified by examining the relationship between diastolic pressure and volume (18), and EDPVR fundamentally characterizes intrinsic passive diastolic ventricular properties (19).
Our data demonstrated reduced diastolic performance accompanied by delayed relaxation and increased stiffness in aging rats. However, EF, CI, SV, SW, and SWI in OC rats were not different from those of YC rats, even though +dP/dt and ESPVR which are more sensitive indexes of cardiac contractility were significantly decreased. Therefore, the findings of the present study are similar to those of isolated diastolic dysfunction with normal LV systolic function in human as evaluated using conventional parameters by noninvasive study. In the present study, long-term exercise training improved myocardial stiffness. LV dilation and hypertrophy were the most common forms of LV remodeling which were found to occur with increasing age. Exercise reversed structural remodeling of LV in old rats, as evidenced by attenuation of LV EDV increment that had occurred in aging rat.
Diastolic dysfunction is composed of an active and a passive component. Isovolumic relaxation as a part of active component can be quantified by calculating -dP/dt and τ(19). Tau is influenced by loading condition and is not straightforwardly associated with elevated left atrial pressure and heart failure (19). Even though the abnormality of active relaxation is an early sign of diastolic dysfunction in cardiovascular diseases, it is far more common in the elderly (20). Therefore, tau may not be significantly improved by training with aging effect itself. But, several authors reported that exercise training in older people and in old animals reverses the reductions in early to late filling phases (21). It is possible that an exercise-induced decrease in myocardial end-diastolic stiffness follows changes in enhanced myocardial relaxation possibly as a result that training reverses the age-associated decline in myocardial calcium uptake (22). Our data showed regular exercise training attenuated myocardial stiffness, not relaxation in old rats. Exercise was associated with borderline-significant increase in -dP/dt (p=0.07) in old rats. Passive stiffness is not precisely separated from active relaxation. So, these changes may reflect LV stiffness.
Diastolic dysfunction integrates abnormalities emanating from sarcomere, extracellular coupling apparatus, myocardial matrix, and cardiac structure and coupling with the vascular system: at the level of the myofilament, modifications of proteins within the contractile thick and thin filaments, myosin-binding protein C, and the linkage protein titin; at the myocyte level, calcium signaling and interaction with myofilaments; at the level of integration, coupling with an ECM and forms bundles among muscle fibers; post-translational cross-linked collagen (including generation of AGEs cross-links) from protein/glucose interaction (23).
Many studies have suggested the importance of myocardial fibrosis to diastolic dysfunction. Connective tissue accumulations are considered a contributor to tissue stiffness. Reduced chamber diastolic stiffness is reported with modifications of collagen (20, 23). Collagen cross-linking as well as collagen type I and total collagen content was found to change in parallel with myocardial stiffness (20). AGEs are commonly found in long-lived proteins in the diabetic and aged populations. Compared with non-diabetic individuals, patients with diabetes manifest premature development of arterial stiffness and diminished LV compliance, which are associated with increase AGEs cross-linked collagen (24).
Collagen becomes increasingly insoluble and resistant to enzymatic and chemical digestion with aging, and this process is accelerated by diabetes and closely correlated with nonenzymatic glycosylation (16). Although accumulations of AGEs are usually associated with prolonged exposure to hyperglycemic conditions, such as, diabetes or aging, other studies have observed AGEs change stimulated by altered hemodynamic loading in euglycemic conditions (25). The locations of glycation cross-links are unknown, but almost certainly involve interfibrillar cross-links to explain the increases in mechanical strength, temperature stability and insolubility (7).
In present study, myocardial collagen solubility decreased in old rats versus young rats, which is consistent with increased collagen cross-liking. They may be associated with increased stiffness observed in old rats. This age related decrease in solubility by pepsin was reversed by exercise training. These results reflect changes in the biochemical characteristics of collagen with aging, which was prevented by exercise training in old rats. Exercise training could potentially alter collagen characteristics even if total collagen concentrations remain unchanged, which concurs with previous results (13). It was suggested that exercise may change pressure, volume, and the neurohormonal state in the myocardium, which may involve changes in the rate of collagen biosynthesis (13, 26), collagen turnover, collagen cross-linking and cardiac remodeling. AGEs are chemically stable, and form irreversible cross-links, which accumulate and remain on the protein until it is degraded (7). Therefore, the prevention of excessive glycation is an important issue.
The relative proportion of two major collagen types I/III are also associated to LV end-diastolic stiffness. But, many studies demonstrate that myocardial collagen concentrations remain unchanged following exercise programs and that exercise training are unable to alter myocardial collage type I/III (13, 27). There are some studies that exercise training may be associated with alterations in collagen metabolism. Thomas et al. (13) reported that percent collagen in rat LV was unaffected by exercise training but, exercise training modulated the effects of aging on collagen gene mRNAs of LV free wall in rats. Training attenuated the decline in LV free wall mRNAs and the increase in ECM collagen cross-linking without affecting the overall increase in collagen concentration. However, there may be positive or negative relationships between variations in gene expression and cross-linking according to pathophysiologic conditions.
Further research should concentrate on elucidating the molecular effects and mechanisms involved, and that ultimately, therapeutics developed to inhibit these deleterious changes. The mechanism responsible for decreasing AGEs cross-linking within collagen in OT rats may be the result of altered AGEs collagen formation and/or collagen degradation. Chelators, sulfhydryl compounds, and antioxidants have also been suggested to act as inhibitors of AGEs-formation by uncoupling glycation from subsequent glycation and cross-linking reactions (16, 28). Thus, it may be suggested that exercise induced anti-oxidative effects could inhibit AGEs-formation.
Unfortunately, the detection of individual AGEs is difficult (29). In the present study, AGE cross-linking within collagen was determined indirectly by measuring pepsin digestibility.
In this experiment, we did not perform afterload manipulation. Therefore, we can not clearly differentiate the component of passive stiffness from the effect of relaxation. Carotid approach provides a merit to evaluate the heart function in physiologic status but have a limitation to manipulate afterload.
In conclusion, long-term exercise training appears to attenuate age-related deteriorations in cardiac contractility and myocardial stiffness and was also related to decreases in myocardial pathologic collagen cross-linking (AGEs cross-linked collagen) in old rats.
Figures and Tables
![]() | Fig. 1Representative pressure-volume (P-V) loops obtained with the Millar P-V conductance catheter system from young and old rats. |
![]() | Fig. 2Relationship between +dP/dt and EDV for young (YC) and old controls (OC), and old trained rats (OT). Note that for the relationship, slope values are higher in OT than OC rats, suggesting that systolic performance is in increased in OT rats. |
![]() | Fig. 3Percent collagen in LV from young (YC) and old controls (OC), and from old trained rats (OT).
*p=0.013 YC vs. OC.
|
![]() | Fig. 4Collagen solubility in LV myocardium from young (YC) and old controls (OC) and from old trained rats (OT).
*p=0.006 YC vs. OC; †p=0.032 OC vs. OT.
|
Table 1
Effects of age and exercise on heart, lung, and body weights, and on collagen volume fraction (CVF) in young control (YC), old control (OC), and old exercise-trained (OT) rats
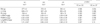
Table 2
Hemodynamic parameters in young control (YC), old control (OC), and old exercise-trained rats (OT) measured using the Millar pressure-volume conductance catheter system
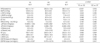
Values are mean±SE. HR, heart rate; MAP, mean arterial pressure; LVSP, left ventricular (LV) systolic pressure; LVEDP, LV end-diastolic pressure; CI, cardiac index; EF, ejection fraction; SWI, stroke work index; +dP/dt and -dP/dt, maximal slope of the systolic pressure increment and diastolic pressure decrement, respectively; τ, time constant of LV pressure decay; SV, stroke volume; LVEDV, LV end-diastolic volume; LVESV, LV end-systolic volume; EDPVR, end-diastolic pressure-volume relation; ESPVR, end-systolic pressure-volume relation.
References
1. Davie AP, Francis CM, Caruana L, Sutherland GR, McMurray JJ. The prevalence of left ventricular diastolic filling abnormalities in patients with suspected heart failure. Eur Heart J. 1997. 18:981–984.


3. Downes TR, Nomeir AM, Smith KM, Stewart KP, Little WC. Mechanism of altered pattern of left ventricular filling with aging in subjects without cardiac disease. Am J Cardiol. 1989. 64:523–527.


4. Lakatta EG, Mitchell JH, Pomerance A, Rowe GG. Human aging: changes in structure and function. J Am Coll Cardiol. 1987. 10:42A–47A.


5. Jyothirmayi GN, Soni BJ, Masurekar M, Lyons M, Regan TJ. Effects of metformin on collagen glycation and diastolic dysfunction in diabetic myocardium. J Cardiovasc Pharmacol Ther. 1998. 3:319–326.


6. Norton GR, Tsotetsi J, Trifunovic B, Hartford C, Candy GP, Woodiwiss AJ. Myocardial stiffness is attributed to alterations in cross-linked collagen rather than total collagen or phenotypes in spontaneously hypertensive rats. Circulation. 1997. 96:1991–1998.


7. Avery NC, Bailey AJ. Enzymic and non-enzymic cross-linking mechanisms in relation to turnover of collagen: relevance to aging and exercise. Scand J Med Sci Sports. 2005. 15:231–240.


8. Bailey AJ, Paul RG, Knott L. Mechanisms of maturation and ageing of collagen. Mech Ageing Dev. 1998. 106:1–56.


9. Asif M, Egan J, Vasan S, Jyothirmayi GN, Masurekar MR, Lopez S, Williams C, Torres RL, Wagle D, Ulrich P, Cerami A, Brines M, Regan TJ. An advanced glycation endproduct cross-link breaker can reverse age-related increases in myocardial stiffness. Proc Natl Acad Sci U S A. 2000. 97:2809–2813.


10. Arbab-Zadeh A, Dijk E, Prasad A, Fu Q, Torres P, Zhang R, Thomas JD, Palmer D, Levine BD. Effect of aging and physical activity on left ventricular compliance. Circulation. 2004. 110:1799–1805.


11. Takemoto KA, Bernstein L, Lopez JF, Marshak D, Rahimtoola SH, Chandraratna PA. Abnormalities of diastolic filing of the left ventricle associated with aging are less pronounced in exercise-trained individuals. Am Heart J. 1992. 124:143–148.
12. Turturro A, Witt WW, Lewis S, Hass BS, Lipman RD, Hart RW. Growth curves and survival characteristics of the animals used in the biomarkers of aging program. J Gerontol A Biol Sci Med Sci. 1999. 54:B492–B501.


13. Thomas DP, Zimmerman SD, Hansen TR, Martin DT, McCormick RJ. Collagen gene expression in rat left ventricle: interactive effect of age and exercise training. J Appl Physiol. 2000. 89:1462–1468.


14. Pacher P, Mabley JG, Liaudet L, Evgenov OV, Marton A, Haskó G, Kollai M, Szabó C. Left ventricular pressure-volume relationship in a rat model of advanced aging-associated heart failure. Am J Physiol Heart Circ Physiol. 2004. 287:H2132–H2137.


15. Woessner JF. The determination of hydroxyproline in tissue and protein samples containing proportions of this amino acid. Arch Biochem Biophys. 1961. 93:440–447.
16. Kochakian M, Manjula BN, Egan JJ. Chronic dosing with aminoguanidine and novel advanced glycosylation end product-formation inhibitors ameliorates cross-linking of tail tendon collagen in STZ-induced diabetic rats. Diabetes. 1996. 45:1694–1700.


17. Candido R, Forbes JM, Thomas MC, Thallas V, Dean RG, Burns WC, Tikellis C, Ritchie RH, Twigg SM, Cooper ME, Burrell LM. A breaker of advanced glycation end products attenuates diabetes-induced myocardial structural changes. Circ Res. 2003. 92:785–792.


18. Zile MR, Brutsaert DL. New concepts in diastolic dysfunction and diastolic heart failure: Part I: diagnosis, prognosis, and measurements of diastolic function. Circulation. 2002. 105:1387–1393.
19. Maurer MS, Spevack D, Burkhoff D, Kronzon I. Diastolic dysfunction: can it be diagnosed by Doppler echocardiography? J Am Coll Cardiol. 2004. 44:1543–1549.
20. Yamamoto K, Masuyama T, Sakata Y, Nishikawa N, Mano T, Yoshida J, Miwa T, Sugawara M, Yamaguchi Y, Ookawara T, Suzuki K, Hori M. Myocardial stiffness is determined by ventricular fibrosis, but not by compensatory or excessive hypertrophy in hypertensive heart. Cardiovasc Res. 2002. 55:76–82.


21. Levy WC, Cerqueira MD, Abrass IB, Schwartz RS, Stratton JR. Endurance exercise training augments diastolic filling at rest and during exercise in healthy young and older men. Circulation. 1993. 88:116–126.


22. Brenner DA, Apstein CS, Saupe KW. Exercise training attenuates age-associated diastolic dysfunction in rats. Circulation. 2001. 104:221–226.


23. Kass DA, Bronzwaer JG, Paulus WJ. What mechanisms underlie diastolic dysfunction in heart failure? Circ Res. 2004. 94:1533–1542.


24. Aronson D. Cross-linking of glycated collagen in the pathogenesis of arterial and myocardial stiffening of aging and diabetes. J Hypertens. 2003. 21:3–12.


25. Herrmann KL, McCulloch AD, Omens JH. Glycated collagen cross-linking alters cardiac mechanics in volume-overload hypertrophy. Am J Physiol Heart Circ Physiol. 2003. 284:H1277–H1284.
26. Takala TE, Rämö P, Kiviluoma K, Vihko V, Kainulainen H, Kettunen R. Effects of training and anabolic steroids on collagen synthesis in dog heart. Eur J Appl Physiol Occup Physiol. 1991. 62:1–6.


27. Woodiwiss AJ, Oosthuyse T, Norton GR. Reduced cardiac stiffness following exercise is associated with preserved myocardial collagen characteristics in the rat. Eur J Appl Physiol Occup Physiol. 1998. 78:148–154.


28. Fu MX, Wells-Knecht KJ, Blackledge JA, Lyons TJ, Thorpe SR, Baynes JW. Glycation, glycoxydation, and cross-linking of collagen by glucose. Kinetics, mechanisms, and inhibition of late stages of the Maillard reaction. Diabetes. 1994. 43:676–683.