Abstract
The standard iron-chelator deferoxamine is known to prevent the growth of coagulase-negative staphylococci (CoNS) which are major pathogens in iron-overloaded patients. However, we found that deferoxamine rather promotes the growth of coagulase-positive Staphylococcus aureus. Accordingly, we tested whether deferiprone, a new clinically-available iron-chelator, can prevent the growth of S. aureus strains as well as CoNS. Deferiprone did not at least promote the growth of all S. aureus strains (n=26) and CoNS (n=27) at relatively low doses; moreover, it could significantly inhibit the growth of all staphylococci on non-transferrin-bound-iron and the growth of all CoNS on transferrin-bound iron at relatively high doses. At the same doses, it did not at least promote the growth of all S. aureus strains on transferrin-bound-iron. These findings indicate that deferiprone can be useful to prevent staphylococcal infections, as well as to improve iron overload, in iron-overloaded patients.
The human body is potentially so highly nutritious that it can well nourish some bacteria. However, it maintains an iron-restricted condition, as most iron is bound to iron-withholding glycoproteins such as transferrin and lactoferrin, or is sequestered within cells. This low iron-availability provides a non-specific defense mechanism that limits bacterial growth within the human body. Conversely, in order for bacteria to grow within the human body, they must be able to acquire iron effectively. For this purpose, most bacteria have developed their specific high-affinity iron-uptake systems. Among these systems, the siderophore-mediated iron-uptake system is basic and essential in most bacteria (1, 2). The recent increase in the incidence of infections caused by multidrug-resistant bacteria including staphylococci, underlines the importance of identifying novel preventive or therapeutic agents. Bacterial iron-uptake systems are attractive targets for the development of preventive or therapeutic vaccines, especially for multidrug-resistant bacteria. Similarly, iron-chelation therapies capable of preventing bacterial iron-uptake have also received attention as attractive novel preventive or therapeutic modalities (3-7).
In vivo iron-availability increases under some pathological conditions. In vivo bacterial growth can be stimulated by increased iron-availability, and conversely, bacterial growth can be suppressed by reducing iron-availability. Coagulase-negative staphylococci (CoNS), especially S. epidermidis, are known to be major causative agents of septicemia in patients who have received anticancer chemotherapy or stem cell transplantation, because serum iron levels are elevated in these patients (8-10). Recently, it was reported that S. epidermidis infections in such patients can be prevented by administering human apotransferrin, which is a major iron-withholding protein (11). Moreover, it was reported that the growth of coagulase-positive Staphylococcus aureus in human serum is suppressed by administrating lactoferrin, another iron-withholding protein (12). These findings suggest a possibility that chemical iron-chelating agents can also be used to prevent staphylococcal infections, as well as to improve iron overload in such patients.
Deferoxamine (C25H48N6O8·CH4O3S) is the best known hydroxamate siderophore derived from Streptomyces pilosus, and is currently being used as the standard parenteral iron-chelator for the treatment of iron-overload (13). In addition, deferoxamine is known to have an antimicrobial effect, mainly because it competes with bacteria for available iron (14). In vitro studies have demonstrated that deferoxamine has bacteriostatic activity against some pathogenic bacteria including S. epidermidis, especially in the presence of ascorbic acid (14-18). However, one of the drawbacks of deferoxamine is that some pathogenic bacteria, including Yersinia enterocolitica, Vibrio vulnificus and S. aureus, are able to utilize the drug for iron-uptake, in the same manner as they use their own siderophores via specific receptors (19-23). This cautions that deferoxamine therapy in iron-overloaded patients can facilitate fatal infections caused by Y. enterocolitica, V. vulnificus and S. aureus.
Recently, a new synthetic oral iron-chelator deferiprone (1,2-dimethyl-3-hydroxypyrid-4-one) became clinically available (24, 25). Deferiprone forms strong complexes with iron at physiological pH. Deferiprone captures iron in vitro from transferrin, lactoferrin, ferritin, and hemosiderin, and in vivo after its parenteral or intragastric administration, and the effect of deferiprone is similar to or greater than that of parenteral deferoxamine. Moreover, deferiprone has been found to inhibit the growths of Y. enterocolitica and V. vulnificus capable of utilizing deferoxamine because structurally it is completely unlike deferoxamine (20, 26). However, whether deferiprone can inhibit or facilitate the growth of staphylococci, especially S. aureus, has not been determined yet. Accordingly, in this study, we determined the effect of deferiprone on the in vitro growth of staphylococci.
S. aureus KCTC1927 (ACTC6538), S. epidermidis KCTC1917 (ACTC12228) and S. saprophyticus KCTC3345 (ACTC15305) strains were purchased from the Korean Collection for Type Cultures (http://kctc.kribb.re.kr/). CoNS (n=25) and S. aureus strains (n=25) isolated from various clinical samples were used in this study. Production of coagulase was tested using Staphaurex Plus kits (Murex Biotech Limited, Dartford, U.K.). CoPS included methicillin-susceptible strains (n=11) and methicillin-resistant strains (n=14). Brain Heart Infusion (BHI, BD, Sparks, MD, U.S.A.) agar was used to subculture all staphylococci. Staphylococcal siderophore detection (SSD) medium, which was recommended as a minimal medium containing low phosphate and citrate for studying staphylococcal iron-uptake systems (27), was used without deferration, because its iron concentration was less than 1 µg/dL (28). When necessary, 1 µM of ferric chloride (FC) as a non-transferrin-bound-iron source or 0.5 mg/mL of human holotransferrin (HT; 1,200-1,600 µg of iron per 1 g of protein) as a transferrin-bound-iron source was added to SSD agars or broths. Deferoxamine was purchased from Ciba-Geigy (Basel, Switzerland) and deferiprone from Apotex Inc. (Toronto, Canada). Unless otherwise noted, all reagents were purchased from Sigma (Sparks, MO, U.S.A.).
Staphylococci were preconditioned in BHI broth containing 200 µM dipyridyl, an iron chelator, at 37℃ overnight in order to adapt them to iron-restricted conditions and to reduce intracellular iron stores. In order to observe the effect of deferoxamine on staphylococcal growth, about 1×104 cfu of the preconditioned staphylococci were spread onto SSD agars supplemented with 1 µM FC (or 0.5 mg/mL HT). Paper discs containing 30 µL of deferoxamine solution (100 µM) or phosphate-buffered saline were then placed on agar surfaces. Because S. aureus strains generally grew more rapidly than CoNS on SSD agars, we observed S. aureus growths after 24 hr but CoNS growths after 48 hr. Response to deferoxamine was determined by the presence of a growth-stimulatory zone or a growth-inhibitory zone around discs containing deferoxamine, as shown in Fig. 1.
Staphylococci, preconditioned as above, were inoculated into SSD broths containing 1 µM FC (or 0.5 mg/mL HT) or into SSD broths containing 1 µM FC (or 0.5 mg/mL HT) plus 0.5-1.5 mM deferiprone at about 1×106 cfu/mL, and then cultured with vigorous shaking (220 rpm) for 6 hr or 24 hr at 37℃. During culture, aliquots were withdrawn at appropriate times, and bacterial growths were measured by optical densities at a wavelength of 600 nm (OD600). Response to deferiprone was determined by comparing the OD600 ratios of strains after culture for 6 hr in broths with/without deferiprone. Response was arbitrarily expressed using OD ratios, as; less than 0.8 (growth inhibition), 0.8-1.2 (no response) and more than 1.2 (growth stimulation). The responses of S. aureus KCTC1927, S. epidermidis KCTC1917, and S. saprophyticus KCTC3345 strains are presented as growth kinetics over 24 hr.
During culture in SSD broths containing HT, culture supernatants were obtained by centrifuging culture aliquots obtained at appropriate times at 10,000 rpm for 5 min. Equal volumes (20 µL) of culture supernatants were reacted with sample buffer containing 8 M urea, but not SDS or mercaptoethanol, at 37℃ for 30 min, and then electrophoresed on 5% stacking gel and 6% running gel containing 6 M urea (28). Proteins were visualized by staining with Coomassie brilliant blue R-250. On 6 M urea-gel, transferrin molecules are separated into four forms according to their iron-saturation levels, i.e., apoferric (AP), C-terminal monoferric (MC), N-terminal monoferric (MN), and diferric (DF) forms.
We first tested the responses of staphylococci to deferoxamine (Fig. 1, Table 1). The growth of S. aureus KCTC1927 was enhanced but those of S. epidermidis KCTC1917 and S. saprophyticus KCTC3345 were inhibited by deferoxamine. The growths of 15 S. aureus strains were enhanced around discs containing deferoxamine, but the other 10 strains did not respond. No S. aureus strain showed 'growth inhibition' by deferoxamine. In contrast, only two CoNS (S. epidermidis) strains showed 'growth enhancement' by deferoxamine, whereas the growths of the remaining 23 strains were inhibited. The response of one of the two CoNS strains to deferoxamine was shown in the Fig. 1. Similar results were observed when SSD broths containing HT plus deferoxamine were used (data not shown). Moreover, the responses of S. aureus strains to deferoxamine were not associated with their susceptibilities to methicillin (data not shown). As shown in the Fig. 1, some S. aureus and CoNS strains appeared to respond to phosphate-buffered saline alone, indicating that they can use phosphate for iron-uptake.
To determine whether deferiprone can inhibit the growths of staphylococci on non-transferrin-bound-iron, we cultured S. aureus KCTC1927, S. epidermidis KCTC1917 and S. saprophyticus KCTC3345 strains in SSD broths only, in SSD broths containing 1 µM FC, or in SSD broths containing 1 µM FC plus 0.5-1.5 mM deferiprone. The growths of the three strains were stimulated by the addition of FC (Fig. 2). S. aureus KCTC1927 grew more actively than S. epidermidis KCTC1917 or S. saprophyticus KCTC3345 in iron-limited SSD broths or in SSD broths containing FC. These growth stimulations by FC were inhibited dose-dependently by deferiprone. Deferiprone showed a greater growth-inhibitory effect against S. epidermidis KCTC1917 and S. saprophyticus KCTC3345 than against S. aureus KCTC1927. At less than 0.5 mM, deferiprone did not affect the growths of all the three staphylococci; that is to say, it did not facilitate the growths of all the three staphylococci in contrast with deferoxamine (data not shown).
To determine whether deferiprone inhibits the growths of S. aureus (n=25) and CoNS (n=25) strains on non-transferrin-bound-iron, we cultured all 50 staphylococci in SSD broths containing 1 µM FC or in SSD broths containing 1 µM FC plus 1.5 mM deferiprone at 37℃ for 6 hr. Deferiprone inhibited the growths of all S. aureus and CoNS strains on non-transferrin-bound-iron regardless of the ability to utilize deferoxamine (Table 2) and methicillin susceptibility (data not shown).
To determine whether deferiprone can also inhibit the growths of S. aureus KCTC1927, S. epidermidis KCTC1917 and S. saprophyticus KCTC3345 strains on transferrin-bound-iron, we cultured these strains in SSD broths only, in SSD broths containing 0.5 mg/mL HT or in SSD broths containing 0.5 mg/mL HT plus 0.5-1.5 mM deperiprone for 24 hr. The growths of all three strains were stimulated by the addition of HT (Fig. 3). S. aureus KCTC1927 grew more actively than S. epidermidis KCTC1917 or S. saprophyticus KCTC3345 in SSD broths containing HT (Fig. 3), and it also utilized iron from transferrin more efficiently than the other two staphylococci (Fig. 4). On 6 M urea-gel electrophoresis, transferrin bands were changed from DF to MN or MC to AP forms as the result of the removal of iron from transferrin molecules by staphylococci or deferiprone. The growth stimulations of S. epidermidis KCTC1917 and S. saprophyticus KCTC3345 by HT were inhibited dose-dependently by deferiprone. In contrast, the growth stimulation of S. aureus KCTC1927 by HT was not inhibited by deferiprone. As shown at 0 hr in Fig. 4, deferiprone evidently reduced the iron-saturation level of transferrin by removing iron from diferric transferrin. Nevertheless, S. aureus KCTC1927 still utilized iron from transferrin more efficiently than the other two staphylococci.
To determine whether deferiprone inhibits the growths of S. aureus (n=25) and CoNS (n=25) strains on transferrin-bound-iron, we cultured all 50 staphylococci in SSD broths containing 0.5 mg/mL HT or in SSD broths containing 0.5 mg/mL HT plus 1.5 mM deferiprone at 37℃ for 6 hr. The growths of all CoNS strains were inhibited by deferiprone. In contrast, the growths of all S. aureus strains were not inhibited by deferiprone.
Hartzen et al. reported that deferoxamine can inhibit the growths of staphylococci, especially in the presence of ascorbic acid (16-18). However, according to our results, the responses of staphylococci to deferoxamine appear to vary among staphylococcal species and strains. Deferoxamine was found to stimulate the growths of a few CoNS strains as well as of most S. aureus strains (Table 1). Our results are supported by recent reports, in which S. aureus was found to utilize deferoxamine for iron-acquisition and growth via a specific ATP-binding cassette (ABC) transporter system (23, 29). One iron-regulated ABC transporter has also been reported in S. epidermidis (30). However, it is not determined whether this transporter system is involved in the uptake of deferoxamine-iron complex by S. epidermidis. Overall, it appears that deferoxamine therapy in patients with iron-overload can facilitate staphylococcal infections, especially S. aureus infections. Moreover, deferoxamine is known to stimulate the growths of other pathogenic bacteria including Y. enterocolitica and V. vulnificus (19-22, 26). These problems severely limit the use of deferoxamine for the treatment of iron-overload, although the drug has been used for some time as a standard iron-chelator for the treatment of iron-overload (13).
S. aureus appear to possess more efficient iron-uptake systems than CoNS. In our previous studies (31-34), S. aureus was found to acquire iron efficiently from partially iron-saturated transferrin as well as highly iron-saturated transferrin, whereas S. epidermidis and S. saprophyticus were able to acquire iron only from highly iron-saturated transferrin, but less efficiently than S. aureus. Lindsay et al. found that S. aureus is able to actively grow and produce siderophores in severely iron-limited SSD medium, whereas CoNS grew poorly and did not produce siderophores (27). Trivier and Courcol reported that S. aureus could grow in severely iron-limited media containing 0.04 µM Fe whereas other bacteria could not (35). Martinaho et al. also found that the growth initiation of S. epidermidis is dependent on the presence of readily-available iron, i.e., non-transferrin-bound-iron (10). According to the results of the present study, S. aureus grew more actively in SSD broths containing FC or HT and utilized iron from transferrin more efficiently than S. epidermidis or S. saprophyticus (Fig. 2-4). Similar results were observed for all S. aureus and CoNS strains examined. In addition, deferiprone was found to inhibit the growths of all CoNS strains, but not of S. aureus strains, on transferrin-bound-iron. Overall, all these findings indicate that S. aureus possess more efficient iron-uptake systems that enable them to grow more actively in severely iron-limited conditions and to utilize transferrin-bound-iron more efficiently than CoNS.
CoNS, but not S. aureus, are known to be major causative agents of septicemia in iron-overloaded patients who have received anticancer chemotherapy or stem cell transplantation. CoNS growth proved to be stimulated by elevated non-transferrin-bound-iron or transferrin-bound iron in such iron-overloaded patients (8, 9). According to our results, deferiprone can inhibit significantly the growths of staphylococci, especially CoNS, by reducing iron-availability at high doses; moreover, at least it does not promote the growths of staphylococci, especially S. aureus, at low doses and on transferrin-bound-iron. As shown in Fig. 4, the ability of deferiprone to remove iron from transferrin in vitro and in vivo has been confirmed independently by others under similar conditions (36-38). Accordingly, deferiprone can be useful for the prevention of CoNS infections along with the treatment of iron-overload by reducing both the level of non-transferrin-bound-iron and the iron-saturation level of transferrin.
It has been reported that apotransferrin can prevent S. epidermidis growth in patients with iron-overload (11) and that lactoferrin or apotransferrin can prevent S. aureus growth in human serum or human peritoneal dialysate (12, 32). However, our results show that S. aureus can still efficiently acquire iron from low iron-saturated transferrin (Fig. 3, 4). In addition, apotransferrin and lactoferrin are inevitably destroyed in vivo and must be administered intravenously because of the proteineous natures. In contrast, deperiprone is a simple chemical oral agent and thus is free of these limitations of apotransferrin and lactoferrin (24, 25).
It was reported that deferiprone can also inhibit the growth of Y. enterocolitica by decreasing iron-availability (20). In addition, we recently found that deferiprone can also inhibit the growth of V. vulnificus, which causes fatal septicemia with an associated mortality rate of over 50% (26). Moreover, no one has yet found a bacterium capable of utilizing deferiprone for iron uptake. According to our results, deferiprone did not promote the growths of staphylococci at less than 0.5 mM in contrast with deferoxamine (data not shown). Relatively higher doses of deferiprone (>0.5 mM) were required to inhibit the growths of staphylococci than those were required to inhibit the growth of Y. enterocolitica or V. vulnificus (<0.2 mM). This is attributed to the more efficient iron-uptake systems of staphylococci, which enable them to more actively grow under severely iron-limited conditions than Y. enterocolitica or V. vulnificus; the latter two are considered archetypal ferrophilic or iron-sensitive bacteria that require higher levels of available iron for growth than other bacteria (26, 39). Ascorbic acid is known to enhance the efficacy of deferiprone as it does for deferoxamine (14-18, 40). Accordingly, the deferiprone doses required to inhibit the growths of staphylococci may be reduced by administering it in combination with ascorbic acid. Also, more potent iron-chelators capable of efficiently inhibiting the growth of staphylococci, especially S. aureus, are required (40).
Overall, the present study indicates that, in contrast with deferoxamine, deferiprone can inhibit the growths of staphylococci, especially CoNS, by reducing iron-availability at relatively high doses; moreover, at least it does not promote the growths of staphylococci, especially S. aureus, at relatively low doses. Accordingly, it is thought that deferiprone can be a useful iron-chelator to prevent staphylococcal infections, as well as to improve iron overload, in iron-overloaded patients.
Figures and Tables
Fig. 1
Examples of responses of staphylococci to deferoxamine. Staphylococcus aureus KCTC1927, Staphylococcus epidermidis KCTC1917, Staphylococcus saprophyticus KCTC3345 and a coagulase-negative staphylococcus (CoNS: S. epidermidis) strain were preconditioned by culturing in BHI broth containing 200 µM of dipyridyl at 37℃ overnight to adapt to iron-restricted conditions and to reduce intracellular iron stores. About 103-4 cfu of these three strains were spread onto the surface of SSD agar containing 1 µM FeCl3, and then discs containing 30 µL of phosphate-buffered saline (PBS) or 100 µM deferoxamine were placed on the agar surface. S. aureus KCTC1927 was incubated at 37℃ for 24 hr, and S. epidermidis KCTC1917, S. saprophyticus KCTC3345 and the CoNS strain for 48 hr.
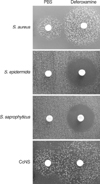
Fig. 2
Effect of deferiprone on the growths of staphylococci on non-transferrin-bound iron. The preconditioned s KCTC1927, Staphylococcus epidermidis KCTC1917 and Staphylococcus saprophyticus KCTC3345 strains were inoculated into SSD broth containing 1 µM FC or in SSD broth containing 1 µM FC plus 0.5-1.5 mM deferiprone (DFP) at ca. 1×106 cfu/mL, and then cultured with vigorous shaking (220 rpm) at 37℃ for 24 hr. During culture, aliquots were withdrawn at appropriate times, and bacterial growths were monitored by measuring OD600 values. Results are expressed as means±standard errors.
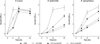
Fig. 3
Effect of deferiprone on the growths of staphylococci on transferrin-bound iron. The preconditioned Staphylococcus aureus KCTC1927, Staphylococcus epidermidis KCTC1917 and Staphylococcus saprophyticus KCTC3345 were inoculated into SSD broth containing 0.5 mg/mL HT or in SSD broth containing 0.5 mg/mL HT plus 0.5-1.5 mM deferiprone (DFP) at ca. 1×106 cfu/mL, and then cultured with vigorous shaking (220 rpm) at 37℃ for 24 hr. The other abbreviations and symbols are as in Fig. 2.
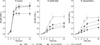
Fig. 4
Utilization of transferrin-bound iron by staphylococci in the absence or presence of deferiprone. During culture in SSD broth containing holotransferrin (HT) or in SSD broth containing HT plus deferiprone (DFP), as shown in Fig. 3, culture supernatants were obtained by centrifuging culture aliquots at 10,000 rpm for 5 min at appropriate times. Equal volumes (20 µL) of culture supernatants were electrophoresed on 6 M urea-polyacrylamide gels, and stained with Coomassie blue. AP, MC, MN, and DF indicate apoferric, C-terminal monoferric, N-terminal monoferric and diferric transferrins, respectively.
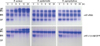
Table 1
Responses of staphylococci to deferoxamine
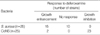
Details were described in the section of Materials and Methods. Response to deferoxamine was determined by the presence of a growth-enhancing zone or a growth-inhibitory zone around a disc containing deferoxamine as shown in Fig. 1. The experiment was repeated twice and results were consistent.
CoNS, coagulase-negative staphylococci.
References
1. Ratledge C, Dover LG. Iron metabolism in pathogenic bacteria. Ann Rev Microbiol. 2000. 54:881–941.


2. Brown JS, Holden DW. Iron acquisition by Gram-positive bacterial pathogens. Microbes Infect. 2002. 4:1149–1156.


3. Kontoghiorghes GJ, Weinberg ED. Iron: mammalian defense mechanisms, mechanisms of disease, and chelation therapy approaches. Blood Rev. 1995. 9:33–45.
4. von Bonsdorff L, Tolo H, Lindeberg E, Nyman T, Harju A, Parkkinen J. Development of a pharmaceutical apotransferrin product for iron binding therapy. Biologicals. 2001. 29:27–37.


5. Brown JS, Ogunniyi AD, Woodrow MC, Holden DW, Paton JC. Immunization with components of two iron-uptake ABC transporters protects mice against systemic infection with Streptococcus pneumoniae. Infect Immun. 2001. 69:6702–6706.
6. Etz H, Minh DB, Henics T, Dryla A, Winkler B, Triska C, Boyd AP, Sollner J, Schmidt W, von Ahsen U, Buschle M, Gill SR, Kolonay J, Khalak H, Fraser CM, von Gabain A, Nagy E, Meinke A. Identification of in vivo expressed vaccine candidate antigens from Staphylococcus aureus. Proc Natl Acad Sci USA. 2002. 99:6573–6578.


7. Marx JJ. Iron and infection: competition between host and microbes for a precious element. Best Pract Res Clin Haematol. 2002. 15:411–426.


8. Bradley SJ, Gosriwitana I, Srichairatanakool S, Hider RC, Porter JB. Non-transferrin-bound iron induced by myeloablative chemotherapy. Br J Haematol. 1997. 99:337–343.


9. Sahlstedt L, Ebeling F, von Bonsdorff L, Parkkinen J, Ruutu T. Non-transferrin-bound iron during allogeneic stem cell transplantation. Br J Haematol. 2001. 113:836–838.


10. Matinaho S, von Bonsdorff L, Rouhiainen A, Lönnroth M, Parkkinen J. Dependence of Staphylococcus epidermidis on non-transferrin-bound iron for growth. FEMS Microbiol Lett. 2003. 196:177–182.
11. von Bonsdorff L, Sahlstedt L, Ebeling F, Ruutu T, Parkkinen J. Apotransferrin administration prevents growth of Staphylococcus epidermidis in serum of stem cell transplant patients by binding of free iron. FEMS Immunol Med Microbiol. 2003. 37:45–51.
12. Aguila A, Herrera AG, Morrison D, Cosgrove B, Perojo A, Montesinos I, Perez J, Sierra G, Gemmell CG, Brock JH. Bacteriostatic activity of human lactoferrin against Staphylococcus aureus is a function of iron-binding properties and is not influenced by antibiotic resistance. FEMS Immunol Med Microbiol. 2001. 31:145–152.
13. Olivieri NF, Brittenham GM. Iron-chelating therapy and the treatment of thalassemia. Blood. 1997. 89:739–761.


14. van Asbeck BS, Marcelis JH, Struyvenberg A, van Kats JH, Verhoef J. Inhibition of bacterial multiplication by the iron chelator deferoxamine; potentiating effect of ascorbic acid. Eur J Clin Microbiol. 1983. 2:426–431.


15. van Asbeck BS, Marcelis JH, van Kats JH, Jaarsma EY, Verhoef J. Synergy between the iron chelator deferoxamine and the antimicrobial agents gentamicin, chloramphenicol, cefalothin, cefotiam and cefsulodin. Eur J Clin Microbiol. 1983. 2:432–438.


16. Hartzen SH, Frimodt-Moller N, Thomsen VF. The antibacterial activity of a siderophore. 3. The activity of deferoxamine in vitro and its influence on the effect of antibiotics against Escherichia coli, Proteus mirabilis and coagulase-negative staphylococci. APMIS. 1994. 102:219–226.
17. Hartzen SH, Frimodt-Moller N, Thomsen VF. The antibacterial activity of a siderophore. 2. The influence of deferoxamine alone and combined with ascorbic acid on the activity of antibiotics against Staphylococcus aureus. APMIS. 1991. 99:879–886.
18. Hartzen SH, Frimodt-Moller N, Frolund Thomsen V. The antibacterial activity of a siderophore. 1. In vitro activity of deferoxamine alone and in combination with ascorbic acid on Staphylococcus aureus. APMIS. 1989. 97:419–424.
19. Baumler AJ, Hantke K. Ferrioxamine uptake in Yersinia enterocolitica; characterization of the receptor protein FoxA. Mol Microbiol. 1992. 6:1309–1321.


20. Lesic B, Foulon J, Carniel E. Comparison of the effects of deferiprone versus deferoxamine on growth and virulence of Yersinia enterocolitica. Antimicrob Agents Chemother. 2002. 46:1741–1745.


21. Tanabe T, Takata N, Naka A, Moon YH, Nakao H, Inoue Y, Narimatsu S, Yamamoto S. Identification of an AraC-like regulator required for induction of the 78-kDa ferrioxamine B receptor in Vibrio vulnificus. FEMS Microbiol Lett. 2005. 249:309–314.
22. Aso H, Miyoshi S, Nakao H, Okamoto K, Yamamoto S. Induction of an outer membrane protein of 78 kDa in Vibrio vulnificus in the presence of desferrioxamine B under iron-limiting conditions. FEMS Microbiol Lett. 2002. 212:65–70.
23. Sebulsky MT, Hohnstein D, Hunter MD, Heinrichs DE. Identification and characterization of a membrane permease involved in iron-hydroxamate transport in Staphylococcus aureus. J Bacteriol. 2000. 182:4394–4400.
24. Hoffbrand AV, Al-Refaie F, Davis B, Siritanakatkul N, Jackson BF, Cochrane J, Prescott E, Wonke B. Long-term trial of deferiprone in 51 transfusion-dependent iron overloaded patients. Blood. 1998. 91:295–300.


25. Barman Balfour JA, Foster RH. Deferiprone: a review of its clinical potential in iron overload in beta-thalassemia major and other transfusion-dependent diseases. Drugs. 1999. 58:553–558.
26. Kim CM, Park RY, Choi MH, Sun HY, Shin SH. Ferrophilic characteristics of Vibrio vulnificus and potential usefulness of iron chelating therapy. J Infect Dis. 2007. 195:90–98.
27. Lindsay JA, Riley TV, Mee BJ. Staphylococcus aureus but not Staphylococcus epidermidis can acquire iron from transferrin. Microbiology. 1995. 141:197–203.


28. Makey DG, Seal US. The detection of four molecular forms of human transferrin during the iron binding process. Biochem Biophys Acta. 1976. 453:250–256.


29. Sebulsky MT, Heinrichs DE. Identification and characterization of fhuD1 and fhuD2, two genes involved in iron-hydroxamate uptake in Staphylococcus aureus. J Bacteriol. 2001. 183:4994–5000.


30. Cockayne A, Hill PJ, Powell NB, Bishop K, Sims VK, Williams P. Molecular cloning of a 32-kilodalton lipoprotein component of a novel iron-regulated Staphylococcus epidermidis ABC transporter. Infect Immun. 1998. 66:3767–3774.


31. Park RY, Sun HY, Choi MH, Bai YH, Shin SH. Staphylococcus aureus siderophore-mediated iron-acquisition system plays a dominant and essential role in the utilization of transferrin-bound iron. J Microbiol. 2005. 43:183–190.
32. Park RY, Sun HY, Choi MH, Bae YH, Shin SH. Growth of Staphylococcus aureus with defective siderophore production in human peritoneal dialysate solution. J Microbiol. 2005. 43:54–61.
33. Park RY, Sun HY, Choi MH, Bai YH, Shin SH. Utilization of transferrin-bound iron by medically important staphylococcal species. J Bacteriol Virol. 2005. 35:103–112.
34. Chung JH, Park MH, Kim JH, Lim Y, Shin SH. Growth and siderophore production of Staphylococci in human peritoneal dialysate. J Korean Med Sci. 2003. 18:158–162.


35. Trivier D, Courcol RJ. Iron-depletion and virulence in Staphylococcus aureus. FEMS Microbiol Lett. 1996. 141:117–127.
36. Kontoghiorghes GJ. Iron mobilization from transferrin and non-transferrin-bound-iron by deferiprone: implications in the treatment of thalassemia, anemia of chronic disease, cancer and other conditions. Hemoglobin. 2006. 30:183–200.


37. al-Refaie FN, De Silva CE, Wonke B, Hoffbrand AV. Changes in transferrin saturation after treatment with the oral iron chelator deferiprone in patients with iron overload. J Clin Pathol. 1995. 48:110–114.


38. Kontoghiorghes GJ, Pattichis K, Neocleous K, Kolnagou A. The design and development of deferiprone (L1) and other iron chelators for clinical use: targeting methods and application prospects. Curr Med Chem. 2004. 11:2161–2183.


39. Ashrafian H. Hepcidin: the missing link between hemochromatosis and infections. Infect Immun. 2003. 71:6693–6700.
40. Kontoghiorghes GJ, Aldouri MA, Hoffbrand AV, Barr J, Wonke B, Kourouclaris T, Sheppard L. Effective chelation of iron in β-thalassemia with the oral chelator 1,2-dimethyl-3-hydroxypyrid-4-one. Br Med J (Clin Res Ed). 1987. 295:1509–1512.