Abstract
Interleukin-6 (IL-6) can stimulate a variety of tumors including prostatic carcinoma. Research has recently shown that IL-6 may act to stimulate the progression of prostatic cancer. To date, little research has been performed to better understand the nature of granulocyte macrophage colony-stimulating factor (GM-CSF) and the expression of IL-6. The aim of this study was to evaluate the effects of GM-CSF on the expression of IL-6 in prostate cancer-3 (PC-3) cells. The bone-derived PC-3 cell line was used in this study. Reverse transcription polymerase chain reaction (RT-PCR) and real- time PCR were performed to detect IL-6 mRNA expression. The IL-6 protein was measured by enzyme-linked immunosorbent assay (ELISA) after treatment with hGM-CSF. Our data indicated that IL-6 mRNA expression did not increase after treatment with hGM-CSF in comparison to the control group. However, the expression of IL-6 protein was increased compared to the control group. GM-CSF may modulate the post-transcription pathway of IL-6 expression in prostate carcinoma cells. Our data suggest that GM-CSF may have a role in IL-6-mediated development of prostate cancer.
The prevalence of prostate cancer is highest in Western countries. In Korea, the frequency of prostate cancer has increased recently, so that prostate cancer has become important in Korea as well (1).
Interleukin-6 (IL-6) has been shown to have a central role in host defense mechanisms by regulating immune responses, homeostasis, and the induction of the acute phase reaction; it also it plays an important role in the progression of prostate cancer (2). Increased IL-6 levels have been shown to be associated with prostate cancer progression and to the androgen-independent phenotype (3). Many cancers such as melanoma, renal cell carcinoma, Kaposi's sarcoma, ovarian cancer, lymphoma, leukemia, and multiple myeloma have shown to be activated by IL-6 (4, 5). The presence of IL-6 suggests a worse prognosis in many carcinoma types. IL-6 can regulate the expression of androgen-responsive genes in an androgen-independent manner and can induce androgen-independent growth of androgen-dependent human prostate cancer cells (6).
Granulocyte macrophage colony-stimulating factor (GM-CSF) is one of the hematopoietic colony-stimulating factors. It is a glycoprotein that belongs to the family of human growth factors and mediates promyelocyte differentiation and proliferation, enhances the function of mature monocytes and macrophages, inhibit osteoclasts, and activate the division of osteoblasts (7). Several reports suggest that GM-CSF and its receptors are present in many cancers. GM-CSF could be part of a network of paracrine and autocrine loops that modulate prostate carcinoma cell activity, and might be highly expressed with advanced stages of prostate cancer where there is bone and lymph node metastasis (8). However, the pathophysiological functions of GM-CSF in normal as well in as cancer tissue remain unclear. Moreover, immunological effects have resulted in the investigation of GM-CSF in a number of immunotherapeutic antitumor strategies for prostate cancer (9, 10). In our previous study, the viability of the prostate cancer-3 (PC-3) cells has shown to be significantly increased by GM-CSF administration in a dose-and time-dependent manner (11).
Therefore GM-CSF and IL-6 play an important role in the development of prostate cancer as well as its growth and metastasis. The aim of this study was to investigate the relationship of GM-CSF and IL-6.
The hormone refractory PC-3 cell line was obtained from the Korean cell line bank (Seoul, Korea). The basic cell wells
were filled with RPMI 1640 (Gibco, Carlsbad, NY, U.S.A.)
including 2% fetal bovine serum, L-glutamine, penicillin, streptomycin,
and the environment was maintained temperature
37℃ and 5% CO2, room air 95%, and proper humidity.
The 5×105 PC-3 cells were incubated in the basic cell wells. After 24 hr of incubation, 50 ng/mL human GM-CSF (hGM-CSF, Pierce Biotech. Inc., Rockford, IL, U.S.A.) was added to the RPMI-1640 medium containing 2% fetal bovine serum. Cultures of the PC-3 cells were incubated for 4, 8, 12, 24, and 48 hr at 37℃ in humid air with 5% CO2, and total RNA was extracted using the RNeasy Mini Kit (QIAGEN Inc., Valencia, CA, U.S.A.) per the manufacturer's directions. To remove genomic DNA contamination, RNA samples were treated with 2 units DNase I (Roche Diagnostic Corp., Indianapolis, IN, U.S.A.) per 100 µL of RNA at 37℃ for 1 hr. RNA purity was determined by the A260/A280 ratio and quantified via A260. Reverse transcription was performed in a total volume of 40 µL with Reverse Transcriptase AMV kit (Roche, Penzberg, Germany) using 1 µg of RNA, 4 µL of 10×reaction buffer (100 mM Tris, 500 mM KCl, pH 8.3), 5 mM MgCl2, 1 mM dNTP mix (dATP, dCTP, dTTP, dGTP), 50 U RNase inhibitor, 20 U AMV reverse transcriptase, and 4 µL of oligo-p (dT)15 primer. The reverse transcription reactions were incubated at 25℃ for 10 min and then at 42℃ for 60 min followed by 99℃ for 5 min to inactivate the reverse transcriptase using GeneAmp PCR system 2400 (Applied Biosystems, Foster city, CA, U.S.A.). The control samples were incubated for 48 hr and underwent the same treatment except for adding the hGM-CSF.
Conventional polymerase chain reaction (PCR) was carried out in 20 µL volume containing 10 pM each primer (12) (Table 1), 10 mM Tris-Cl (pH 8.3), 2 mM MgCl2, and 200 mM dNTP. PCR was performed with 30 amplification cycles using the GeneAmp PCR system 2400 (Applied Biosystems), and PCR products underwent electrophoresis on 2% agarose gels.
In order to optimize the real-time PCR conditions, and verify the specificity of the designed primer pairs, the optimal primers and template concentrations were determined using 2×SYBR® Green PCR Master Mix (Applied Biosystems) and a melting curve analysis. Real-time PCR assays contained 12.5 µL of 2×SYBR® Green PCR Master Mix, 300 nM each PCR primer, and 2 µL of cDNA from the reverse transcription step (unknown samples) or PCR amplicons from each dilution (standard curve) in 25 µL. Real-time PCR was performed in the ABI PRISM® 7000 (Applied Biosystems) starting with 10 min of preincubation at 95℃, followed by 40 amplification cycles at 95℃ for 15 sec and 60℃ for 1 min and melting curve analysis. Relative quantification of genes was determined for each sample in three separate PCR reactions using the same RNA preparation. The concentration of each gene (IL-6 and glyceraldehyde 3-phosphate dehydrogenase [GAPDH]) was calculated by reference to the respective standard curve with the aid of the ABI PRISM® 7000 software. Relative gene expression was calculated as a ratio of the target gene (IL-6) concentration to housekeeping gene (GAPDH) concentration.
The 5×105 PC-3 cells were incubated in the basic 6 wells. After 24 hr of incubation, 50 ng/mL hGM-CSF was added to the RPMI-1640 wells including 2% fetal bovine serum. After 4, 8, 12, 24, and 48 hr incubation, we measured IL-6 proteins with 6 samples, respectively, using an ELISA kit (Amersham Biosciences, Uppsala, Sweden) in culture media. Six control samples underwent the same treatment except for adding the hGM-CSF. After 48 hr incubation, IL-6 protein manifestation was measured using ELISA.
IL-6 mRNA expression was gradually increased in accordance with time in control groups. After hGM-CSF treatment, IL-6 mRNA expression was increased at 4 hr, decreased until 12 hr, and then increased at 24 and 48 hr (Fig. 1).
Relative gene expression was calculated as the ratio of the target gene (IL-6) concentration to the housekeeping gene (GAPDH) concentration. The expression of IL-6 mRNA was 139.33±24.55 in the control group. After hGM-CSF treatment, the expression of IL-6 mRNA incubated at 4, 8, 12, 24, 48 hr was 134.38±39.23, 81.86±43.12, 93.15±3.11, 124±23.42, and 129.43±43.37, respectively (Fig. 2).
The IL-6 level represents the mean and standard deviation in each of the 6 wells incubated for a different period of time. The concentration of IL-6 was 23.23±4.00 pg/mL in the control group. The IL-6 concentrations, incubated at 4, 8, 12, 24, and 48 hr were 47.40±2.97, 52.19±2.18, 53.65±8.45, 54.06±6.98, and 55.52±9.28 pg/mL, respectively (Fig. 3). The IL-6 protein expression increased after 4 hr of incubation compared to the control group (p<0.01).
In this study, we demonstrated the relationship of GM-CSF with IL-6 in the PC-3 cells. Many reports have shown that GM-CSF and its receptors are expressed in normal tissue, breast cancer, ovarian cancer, bladder cancer, pancreas cancer, small cell cancer of the lung, stomach cancer, and melanoma (13). In prostate cancer, GM-CSF and its receptors are also expressed and may be part of a network of paracrine and autocrine loops that modulate prostate carcinoma cell activity (14). GM-CSF also promotes the differentiation and survival of dendritic cells (15). GM-CSF is presumed to stimulate dendritic cells to uptake and process antigens, leading to cross-priming of antigen specific T cells and an antigen T-cell response. These pleiotropic immunological effects have resulted in the investigation of GM-CSF in a number of immunotherapeutic antitumor strategies for prostate cancer (9, 10). Whether or not therapeutically administered hematopoietic growth factors can stimulate nonhematopoietic cell growth, including solid tumor cells, has remained a controversial issue.
IL-6 is implicated in the regulation of a variety of cellular events in tumor cells. IL-6 exerts different effects on proliferation and apoptosis, and stimulates angiogenesis through the regulation of vascular endothelial growth factor (16). IL-6 is produced in many organs, but its serum level is very low with appropriate modulation in healthy people (17, 18). However, the IL-6 level is significantly increased in metastatic prostate cancer and is strongly related to the serum prostate-specific antigen (PSA) level (19). Some reports have suggested that IL-6 is increased in the hormone refractory phase of prostate cancer when compared to chronic prostatitis and benign prostatic hyperplasia (BPH) (20). Therefore, IL-6 is a potential mediator associated with prostate cancer morbidity and a marker of disease activity for predictive clinical testing. IL-6 receptor mRNA has been identified in all prostate cancer cell lines, but not in epithelia or stromal cells extracted from BPH (21). Okamoto et al. reported that IL-6 functions as a paracrine growth factor for human LNCaP androgen-sensitive prostate cancer cells and as an autocrine growth factor for human DU-145 and PC-3 androgen-insensitive prostate cancer cells (20). However, IL-6 has no function in the stimulation of epithelia derived from BPH (22). The stimulatory and inhibitory effects of IL-6 in the same cell line are not always consistent. Results of numerous studies have demonstrated the pleiotropic nature of IL-6 action in prostate carcinoma cells. Charyulu and Lopez (23) demonstrated for the first time that GM-CSF plays an important role in increased IL-6 production by B-lymphocytes and that this effect, irrespective of the upregulation of tumor necrotic factor-α by GM-CSF, appears to depend on the actual presence of GM-CSF in the microenvironment. They concluded that GM-CSF might function as a direct activator or inducer of IL-6 production. In this study, IL-6 mRNA expression was not increased after hGM- CSF treatment at specific incubation times in hormone-refractory prostate cancer cells in comparison with the control group when measured by conventional and real-time PCR. However, the IL-6 protein concentration was increased after hGM-CSF treatment when measured by ELISA. Therefore, GM-CSF may modulate the post-transcription pathway of IL-6 expression in prostate carcinoma cells. Collectively, our results suggest that GM-CSF may have a more complex role in IL-6-mediated development of prostate cancer. It was postulated that GM-CSF might induce overexpression of IL-6 in the extracellular pathway in addition to the known intracellular pathway. Our data cannot exactly explain the reason for this discrepancy. Further studies to investigate the relationship of IL-6 and GM-CSF in prostate cancer are needed.
Figures and Tables
Fig. 1
Time course of the IL-6 mRNA expression by conventional RT-PCR in PC-3 cells. IL-6 mRNA expression was gradually increased in control groups (lanes 1-5). IL-6 mRNA expression was increased at 4 hr, decreased until 12 hr, and then increased at 24 and 48 hr after hGM-CSF treatment (lanes 6-10). M, molecular size marker; 1-5, control groups; 6-10, hGM-CSF-treated groups.
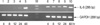
Fig. 2
Time course of the IL-6 mRNA expression by real-time PCR. The PC-3 cells were treated with hGM-CSF for the indicated times. The control samples were incubated for 48 hr. The IL-6 mRNA expression was not increased at specific incubation time.
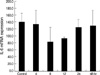
Fig. 3
The average IL-6 concentration (ng/mL) and their standard deviation by the incubations period as determined by ELISA. The control samples were incubated for 48 hr. The IL-6 protein expression was significantly increased at 4, 8, 12, 24, and 48 hr after hGM-CSF treatment compared with the control group. *p<0.01.
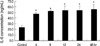
References
1. National Statistical office. Republic of Korea. Korea Statistical Information System. Dec., 31, 2002. Available from: URL:http://kosis.nso.go.kr.
3. Tan D, Wu X, Hou M, Lee SO, Lou W, Wang J, Janarthan B, Nallapareddy S, Trump DL, Gao AC. Interleukin-6 polymorphism is associated with more aggressive prostate cancer. J Urol. 2005. 174:753–756.


4. Chung TD, Yu JJ, Spiotto MT, Bartkowski M, Simons JW. Characterization of the role of IL-6 in the progression of prostate cancer. Prostate. 1999. 38:199–207.


5. Offner FA, Obrist P, Stadlmann S, Feichtinger H, Klingler P, Herold M, Zwierzina H, Hittmair A, Mikuz G, Abendstein B, Zeimet A, Marth C. IL-6 secretion by human peritoneal mesothelial and ovarian cancer cells. Cytokine. 1995. 7:542–547.


6. Lee SO, Lou W, Hou M, de Miguel F, Gerber L, Gao AC. Interleukin-6 promotes androgen-independent growth in LNCaP human prostate cancer cells. Clin Cancer Res. 2003. 9:370–376.
7. Horwood NJ, Udagawa N, Elliott J, Grail D, Okamura H, Kurimoto M, Dunn AR, Martin T, Gillespie MT. Interleukin 18 inhibits osteoclast formation via T cell production of granulocyte macrophage colony-stimulating factor. J Clin Invest. 1998. 101:595–603.


8. Savarese DM, Valinski H, Quesenberry P, Savarese T. Expression and function of colony-stimulating factors and their receptors in human prostate carcinoma cell lines. Prostate. 1998. 34:80–91.


9. Small EJ, Reese DM, Um B, Whisenant S, Dixon SC, Figg WD. Therapy of advanced prostate cancer with granulocyte macrophage colony-stimulating factor. Clin Cancer Res. 1999. 5:1738–1744.
10. Rini BI, Weinberg V, Bok R, Small EJ. Prostate-specific antigen kinetics as a measure of the biologic effect of granulocyte-macrophage colony-stimulating factor in patients with serologic progression of prostate cancer. J Clin Oncol. 2003. 21:99–105.


11. Kim TH, Kim YS, Myung SC, Lee DH, Won EH, Lee SY. The prostaglandin E receptor agonists increase granulocyte macrophage colony-stimulating factor in prostate cancer cells. Korean J Urol. 2004. 45:1272–1278.
12. Nakata H, Uemura Y, Kobayashi M, Harada R, Taguchi H. Cyclooxygenase-2 inhibitor NS-398 suppresses cell growth and constitutive production of granulocyte-colony stimulating factor and granulocyte macrophage-colony stimulating factor in lung cancer cells. Cancer Sci. 2003. 94:173–180.


13. Guillaume T, Sekhavat M, Rubinstein DB, Hamdan O, Symann ML. Transcription of genes encoding granulocyte-macrophage colony-stimulating factor, interleukin 3, and interleukin 6 receptors and lack of proliferative response to exogenous cytokines in nonhematopoietic human malignant cell lines. Cancer Res. 1993. 53:3139–3144.
14. Rokhlin OW, Griebling TL, Karassina NV, Raines MA, Cohen MB. Human prostate carcinoma cell lines secrete GM-CSF and express GM-CSF-receptor on their cell surface. Anticancer Res. 1996. 16:557–563.
15. Markowicz S, Engleman EG. Granulocyte-macrophage-colony stimulating factor promotes differentiation and survival of human peripheral blood dendritic cells in vitro. J Clin Invest. 1990. 85:955–961.
16. Culig Z. Interleukin-6 polymorphism: expression and pleiotropic regulation in human prostate cancer. J Urol. 2005. 174:417.


17. Nakashima J, Tachibana M, Horiguchi Y, Oya M, Ohigashi T, Asakura H, Murai M. Serum interleukin 6 as a prognostic factor in patients with prostate cancer. Clin Cancer Res. 2000. 6:2702–2706.
18. Drachenberg DE, Elgamal AA, Rowbotham R, Peterson M, Murphy GP. Circulating levels of interleukin-6 in patients with hormone refractory prostate cancer. Prostate. 1999. 41:127–133.


19. Adler HL, McCurdy MA, Kattan MW, Timme TL, Scardino PT, Thompson TC. Elevated levels of circulating interleukin-6 and transforming growth factor-beta1 in patients with metastatic prostate carcinoma. J Urol. 1999. 161:182–187.
20. Okamoto M, Lee C, Oyasu R. Interleukin-6 as a paracrine and autocrine growth factor in human prostatic carcinoma cells in vitro. Cancer Res. 1997. 57:141–146.
21. Siegall CB, Schwab G, Nordan RP, FitzGerald DJ, Pastan I. Expression of the interleukin-6 receptor and interleukin-6 in prostate carcinoma cells. Cancer Res. 1990. 50:7786–7788.
22. Ivanovic V, Melman A, Davis-Joseph B, Valcic M, Geliebter J. Elevated plasma levels of TGF-beta 1 in patients with invasive prostate cancer. Nat Med. 1995. 1:282–284.