Abstract
Airway smooth muscle (ASM) hyperplasia and angiogenesis are important features associated with airway remodeling. We investigated the effect of IL-4 and amphiregulin, an epidermal growth factor family member, on the proliferation of human ASM cells and on the release of vascular endothelial growth factor (VEGF) and monocyte chemotactic protein (MCP)-1 from human ASM cells. Human ASM cells were growth-arrested for 48 hr and incubated with platelet-derived growth factor (PDGF)-BB, interleukin (IL)-4, amphiregulin, and VEGF to evaluate cell proliferation. The cells were treated with PDGF, IL-4 and amphiregulin to evaluate the release of VEGF, MCP-1. IL-4 suppressed unstimulated and PDGF-stimulated ASM cell proliferation. Amphiregulin stimulated ASM cell proliferation in a dose-dependent manner. VEGF did not have any influence on ASM cell proliferation. IL-4 stimulated VEGF secretion by the ASM cells in a dose-dependent manner and showed added stimulatory effects when co-incubated with PDGF. Amphiregulin did not promote VEGF secretion. IL-4 and amphiregulin showed no stimulatory effects on MCP-1 secretion. The results of this study showed that IL-4 had bifunctional effects on airway remodeling, one was the suppression of the proliferation of the ASM cells and the other was the promotion of VEGF release by the ASM cells, and amphiregulin can promote human ASM cell proliferation.
Asthma, a chronic airway inflammatory disease, is characterized by reversible airway obstruction, airway inflammation and airway hyperreactivity. Repeated airway inflammation can lead to irreversible structural change and airflow obstruction, namely airway remodeling. Airway smooth muscle (ASM) cell hyperplasia and hypertrophy are key factors in airway remodeling (1). Airway smooth muscle cells are the major effector cells that regulate bronchomotor tone in asthma. However, new evidence suggests that ASM cells are also an important source of pro-inflammatory cytokines, chemokines, growth factors, and extracellular matrix (ECM) components (2).
Interleukin (IL)-4 and IL-13 are T helper (Th) 2 lymphocyte-derived cytokines that induce T cell differentiation to a Th2 phenotype as well as isotype switching of B cells to IgE producing cells. Even though experimental evidence has firmly established a key role for IL-4 and IL-13 in acute allergic inflammation (3), their activity in airway remodeling has not been fully elucidated. IL-4 and IL-13 act through a common subunit of their receptor complexes, the IL-4 receptor α subunit (IL-4Rα) in ASM cells, and regulate allergic inflammation and tissue remodeling in the airways (4). IL-4Rα-deficient mice fail to develop goblet-cell metaplasia and airway hyperreactivity (5). However, many studies have shown greater activity of IL-13 compared to IL-4 in allergic inflammation including goblet-cell metaplasia, mucus overproduction, airway hyperreactivity, ASM cell migration, and airway remodeling (3, 6, 7, 8). Furthermore, it has been suggested that they have different activities in their effector properties; IL-4 plays a more prominent role in the initiation phase of Th2 inflammation, whereas IL-13 is more prominent in the effector phase of Th2 inflammation (1). IL-4 has been shown to have either proliferative or anti-proliferative properties depending on the cell type (9-14). However, there is limited information on the effect of IL-4 on airway smooth muscle cell proliferation (12).
The vascular endothelial growth factor (VEGF) contributes to the airway remodeling in asthma by increasing angiogenesis and vascular permeability. Patients with asthma have been shown to have increased levels of VEGF in bronchoalveolar lavage fluid as well as VEGF receptor positive vessels in biopsy samples (15). The effect of IL-4 on the regulation of VEGF has not been completely characterized. In smooth muscle cells, IL-4 and IL-13 have been shown to increase VEGF expression (16, 17). On the other hand, in patients with rheumatoid arthritis, IL-4 inhibits VEGF production in synovial fibroblasts (18). Furthermore, to date, the effects of VEGF on cellular proliferation remain unclear.
In this study, we investigated the impact of IL-4, VEGF, and amphiregulin on the proliferation of human ASM cells. Amphiregulin is a polypeptide growth factor that belongs to the epidermal growth factor (EGF) family. Amphiregulin, like other EGF family members, plays an important role in cell processes. These include cell proliferation, survival, differentiation, and migration. However, it has not been demonstrated whether amphiregulin can promote human ASM cell proliferation. In addition, we evaluated whether IL-4 and amphiregulin induced the release of VEGF, MCP-1 and MIP-1α from ASM cells.
Primary human ASM cells and cell growth supplement were purchased from Clonetics (San Diego, CA, U.S.A.). Penicillin, streptomycin, fetal bovine serum (FBS) and 10% Dulbecco's modified Eagle's medium (DMEM), DMEM/F-12 medium were obtained from Gibco BRL. Bovine serum albumin (BSA) and insulin-transferrin-selenium (ITS) were obtained from Sigma. IL-4, amphiregulin, platelet-derived growth factor (PDGF)-BB, VEGF, monoclonal anti-human VEGF antibody, and monoclonal anti-human VEGF R2 antibody were purchased from R&D (R&D systems, Minneapolis, MN, U.S.A.).
Human ASM cells were placed in 75 cm2 culture flask with 10% FBS/DMEM containing 100 IU/mL penicillin, 100 µg/mL streptomycin, and 2 mM L-glutamine and incubated in a humidified incubator at 37℃, 5% CO2. When the cells became confluent, they were passaged with the use of 0.025% trypsin in 0.01% EDTA. Cells at passages 3 to 6 were used in all experiments.
Human ASM cells were seeded at a density of 1×104 cells/cm2 in 96-well culture plates. When cells reached 70% confluence, growth was arrested in serum-free DMEM/F-12 medium containing 0.1% BSA for 48 hr. The cells were then incubated with 20 ng/mL of PDGF-BB, 10, 50, and 100 ng/mL of IL-4, 10, 30, and 50 ng/mL of VEGF and 10, 50, and 100 ng/mL of amphiregulin for 48 hr. Cells were also treated with 100 ng/mL of monoclonal anti-human VEGF antibody and/or 100 ng/mL monoclonal anti-human VEGF R2 antibody in the presence of PDGF to evaluate the effect of VEGF on the cell proliferation.
Cell proliferation was measured using a bromodeoxyuridine (BrdU) cell proliferation ELISA kit (Roche Applied Science, Mannheim, Germany). Briefly, cells were cultured in 96-well plates under the conditions described above and incubated with 10 µM BrdU for 24 hr. BrdU incorporation into DNA was detected using a commercial kit.
To evaluate the effect of cytokines on the production of VEGF, MCP-1 and MIP-1α from human ASM cells, the cells were cultured to confluence in 10% FCS/DMEM in humidified 5% CO2 air at 37℃ in 24-well culture plates and growth-arrested in serum-free DMEM/F-12 medium for 48 hr. Cells were stimulated with 20 ng/mL of PDGF-BB, 10, 50, and 100 ng/mL of IL-4 and 50, 100, and 150 ng/mL of amphiregulin. After 24-hr incubation, the cell culture supernatant was harvested and stored at -80℃ until the ELISA for cytokines was performed.
Fig. 1 shows the proliferation of human ASM cells treated with 20 ng/mL of PDGF and the indicated concentrations of IL-4. IL-4 significantly suppressed the proliferation of ASM cells at 10, 50, and 100 ng/mL compared to the untreated cells (p<0.001). To determine the effect of IL-4 on PDGF-induced proliferation, the cells were treated with IL-4 in the presence of PDGF. IL-4 also significantly inhibited the PDGF-induced proliferation of ASM cells at 10 and 100 ng/mL (p<0.001) (Fig. 1).
To evaluate the effect of amphiregulin on the proliferation of ASM cells, different concentrations of amphiregulin were added to the cultured ASM cells. Amphiregulin induced significant cell proliferation at 50 and 100 ng/mL (p<0.01). We also evaluated the effect of amphiregulin on the proliferation of ASM cells in combination with PDGF. However, amphiregulin did not demonstrate synergistic effects on cell proliferation compared to the proliferation of cells stimulated with PDGF only (Fig. 2).
Fig. 3 shows the effect of VEGF on the proliferation of human ASM cells. Cells were incubated with 10, 30, and 50 ng/mL of VEGF for 48 hr. However, VEGF did not show any effect on the proliferation of ASM cells at all concentrations, whereas PDGF increased the proliferation of ASM cells. When ASM cells were incubated with VEGF in combination with PDGF, the proliferation was not augmented compared to that of cells treated with PDGF only.
When ASM cells were incubated with PDGF, not only cell proliferation but also VEGF secretion was elevated. We investigated whether ASM cell proliferation could be regulated by anti-VEGF antibody or anti-VEGF R2 antibody. ASM cells were stimulated with PDGF and treated with 100 ng/mL of anti-human VEGF antibody and/or 100 ng/mL of anti-human VEGF R2 antibody in presence of PDGF. However, neither anti-human VEGF antibody nor anti-human VEGF R2 antibody influenced ASM cell proliferation (Fig. 3).
Fig. 4 shows the release of VEGF by human ASM cells treated with the indicated concentration of IL-4, amphiregulin and PDGF for 24 hr. The concentration of VEGF released from control ASM cell culture was approximately 1,046±81.99 pg/105 cells. IL-4 increased the production of VEGF by human ASM cells in a concentration-dependent manner (p<0.001). PDGF (20 ng/mL) also significantly increased VEGF secretion from the human ASM cells (p<0.001). We evaluated the release of VEGF by ASM cells treated with the indicated concentrations of IL-4 in presence of PDGF. The release of VEGF by cells treated with IL-4 and PDGF was significantly higher than that of the cells incubated with PDGF only (p<0.01). Amphiregulin treatment had little effect on VEGF release from the human ASM cells at all concentrations (50, 100, and 150 ng/mL) (Fig. 4).
Fig. 5 shows the effect of IL-4, amphiregulin and PDGF on the release of MCP-1. PDGF increased the release of MCP-1 from the ASM cells, whereas IL-4 and amphiregulin had little stimulatory effect on MCP-1 secretion at 50 and 100 ng/mL concentrations (Fig. 5). MIP-1α was not detected in the cultured cell supernatants (data not shown).
In this study, IL-4 inhibited the proliferation of stimulated and unstimulated human ASM cells. The lowest inhibitory concentration of IL-4 was 10 ng/mL, and the inhibition was not dose dependent. Both IL-4 and IL-13 have been shown to play a key role in regulating allergic inflammation and Th2-induced tissue remodeling (19, 20). However, the actual role of IL-4 on airway remodeling has not been fully elucidated. Even though IL-4 and IL-13 have in common the IL-4 receptor, IL-4Rα, originally, IL-13 was presumed to have the same effector function as IL-4. However, new evidence suggests that they have different roles in allergic inflammation and airway remodeling. IL-4-mediated remodeling responses have been reported to be less efficient than IL-13 (1, 3, 7). In a study using transgenic Balb/c mice, the mice with the IL-4 transgene do not show airway wall fibrosis or bronchial hyperreactivity, whereas IL-13 promotes subepithelial fibrosis, goblet-cell metaplasia and smooth-muscle cell proliferation (7, 21). However, IL-4-induced fibrosis appears to be a strain-specific response, since recent findings showed that fibrosis was found in transgenic IL-4 C57BL/6 mice in contrast to Balb/c mice (22). IL-13 has also been shown to augment human ASM migration toward PDGF, while IL-4 does not (8, 23). IL-13, but not IL-4, enhances Src-kinase phosphorylation and increases human ASM cell migration. Src is a key member of the mitogenic signaling cascade in many cell types (23). PDGF and EGF induce rapid activation of Src and promote cell proliferation. It is possible that failure of Src-kinase phosphorylation of IL-4 contributes to the inhibition of ASM cell proliferation.
IL-4 has different regulatory functions in cell proliferation depending on the cell type. IL-4 suppresses the proliferation of human tumor cell lines, astrocytes, human umbilical vein endothelial cells, vascular smooth muscle cells, pre-adipocyte cells, and airway smooth muscle cells, while it promotes the proliferation of fibroblasts and endothelial cells (10, 12, 24-28). IL-4Rα as well as IL-13RαI and IL-13RαII have all been reported to be a constitutively expressed in human ASM cells (4, 29). However, there is limited data on the signaling pathway of IL-4 after it binds to its receptor. In one study, on cultured human ASM cells, IL-4 and IL-13 activated IL-4Rα and induced phosphorylation of its signal tranducer and activation of transcription-6 (STAT6), p42/p44 ERK and p38 mitogen-activated protein (MAP) kinase in cultured human ASM cells (29). However, since ERK and p38 MAP kinase are known to be important intracellular pathways for cell proliferation (30), it is unlikely that IL-4 suppresses ASM cell proliferation through them. It has been suggested that IL-4 decreases ASM cell proliferation by a decrease in cyclin D1 protein expression rather than a c-AMP dependent mechanism (12) or through STAT6 activation (28). However, IL-4 also enhances PDGF-induced proliferation in fibroblasts via the STAT6 pathway (31). Therefore, IL-4 appears to play a different role based on the cell type via mainly STAT6.
Contrary to our results, it has been suggested that IL-4 and IL-13 induce ASM cell proliferation via an autocrine loop of PDGF (32). The pretreatment with fibroblast growth factor (FGF)-2 caused stimulation of PDGF receptor (PDGFR) alpha expression and ASM cell proliferation was augmented with IL-4 and IL-13. However, in that study, neither IL-4 nor IL-13 induced ASM cell proliferation without FGF-2 pretreatment, even though they induced PDGF-AA and PDGF-CC. Since we did not stimulate with FGF-2, the PDGFR alpha expression might not have been facilitated. However, we evaluated the ASM cells with and without PDGF-BB, and IL-4 inhibited cell proliferation in both cases. PDGF-BB binds to both the PDGFR alpha and PDGFR beta, while PDGF-AA binds only to the PDGFR alpha (33). PDGFR beta is five to six times more prominent in the ASM cells compared to PDGFR alpha and PDGF-BB has a more potent mitogenic effect than does PDGF-AA (34). Therefore, it is unlikely that upregulated PDGFR alpha expression was related to the IL-4-mediated cell proliferation. Further studies are needed to identify the signaling pathways that mediate IL-4-induced inhibition of PDGF-enhanced ASM proliferation.
Increased vascularity and enlarged congested mucosal blood vessels have been reported in biopsy specimens from the airways of asthmatics (35). VEGF is important to angiogenic activity in the airways. Expression of VEGF and its receptors is upregulated in asthma. The degree of airway vascularity has been found to correlate with VEGF expression (36). In this study, VEGF release by ASM cells was augmented by stimulation with IL-4, but not with amphiregulin. Even though smooth muscle hyperplasia and increased vascularity are common findings in the airways of asthmatic subjects, only PDGF enhanced both VEGF release and cell proliferation. IL-4 increased VEGF release, but it suppressed smooth muscle proliferation. By contrast, amphiregulin increased smooth muscle hyperplasia, but did not augment VEGF release. Even though airway remodeling is associated with the injury and repair process during allergic inflammation, factors associated with the remodeling may be different from those related to the allergic inflammation.
Amphiregulin, a member of the EGF family, plays an important role in the proliferation of vascular smooth muscle cells (37), neural stem cells (38), cell survival (39), and differentiation (38). There are only a few reports on the relationship between amphiregulin and airway remodeling in asthma. In a study on asthmatic subjects, amphiregulin expression was upregulated in the mast cells, in patients with asthma, compared to normal controls. In addition, its upregulation is significantly correlated with the goblet cell hyperplasia in the mucosa of the patients with asthma (40). Furthermore, the amphiregulin, secreted from mast cells, promoted the proliferation of primary human lung fibroblasts and induced lung fibrosis (41). In this study, amphiregulin augmented human ASM cell proliferation. This is the first report to demonstrate that amphiregulin promotes ASM cell proliferation. However, it did not influence the secretion of VEGF and MCP-1 from human ASM cells. In a chronic asthma mouse model, amphiregulin was not associated with remodeling of the walls of airways (42).
In this study, VEGF release from the human ASM cells was augmented by PDGF stimulation compared to the spontaneous release from cells. Since PDGF acted as a strong growth-stimulant factor in the ASM cells, we investigated whether VEGF activity was an autocrine growth-stimulant factor in the ASM cells response to PDGF. We blocked the VEGF receptor with VEGF R2 antibody and neutralized VEGF with anti-human VEGF antibody; then the ASM cells were stimulated with PDGF. VEGF R1, flt-1, VEGF R2, and KDR were shown to be highly expressed in the ASM cells (43). However, the blocking and neutralizing antibodies did not inhibit PDGF-enhanced cell proliferation. Furthermore, ASM cells did not proliferate with VEGF stimulation, when we treated the ASM cells with VEGF only. This finding is consistent with those reported by Kazi et al. (43). Given the unresponsiveness of the ASM cells to VEGF, VEGF-receptor blocking and VEGF-neutralizing antibodies, it is unlikely that VEGF contributes to the smooth muscle hyperplasia in airway remodeling.
MCP-1 and MIP-1α are C-C chemokines; they play an important role in allergic inflammation, bronchial hyperresponsiveness and the recruitment of eosinophils in bronchial asthma (44). C-C chemokine receptors for MIP-1α and MCP-1 were reported to be expressed in vascular smooth muscle cells (45). In this study, only MCP-1, but not MIP-1α, was released from ASM cells spontaneously and after IL-4 and amphiregulin treatment. However, in contrast to VEGF release, there was no significant difference between the spontaneous release and the release associated with IL-4 and amphiregulin.
Our findings suggest that IL-4 may have a bifunctional role in airway remodeling; one characterized by suppression of the airway smooth muscle hyperplasia, and the other by the increase in VEGF release from the airway smooth muscle cells. Since this was an in vitro cell culture study, the results include the following limitations. The effects of chronic exposure to IL-4 on airway smooth muscle cell proliferation could not be evaluated. During the acute phase of bronchial asthma, increased vascular permeability by VEGF leads to mucosal edema and airway narrowing. Sustained allergic inflammation over time leads to more permanent structural changes in the airways including subepithelial fibrosis and smooth muscle cell hyperplasia. Therefore, IL-4 stimulation for 24 to 48 hr cannot show the chronic effects on smooth muscle cell proliferation. Nevertheless, IL-4 might be used in the future as a therapeutic modality for the modification of ASM cellular proliferation in airway remodeling. Human ASM cells can also participate in the pathogenesis of asthma by release of chemokines and growth factors such as MCP-1 and VEGF, and amphiregulin can promote human ASM cell proliferation. These results suggest potential targets for the development of additional asthma therapy.
Figures and Tables
Fig. 1
Effect of IL-4 on the proliferation of human ASM cells. (A) When cells were treated with IL-4 (10, 50, and 100 ng/mL), cell proliferation was significantly suppressed at all concentrations compared to control cells. *p<0.001 vs. Control and PDGF. (B) When cells were treated with IL-4 (10, 100 ng/mL) in presence of PDGF, IL-4 inhibited PDGF-enhanced cell proliferation. †p<0.001 vs. PDGF.
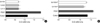
Fig. 2
Effect of amphiregulin on the proliferation of human ASM cells. (A) Amphiregulin induced cell proliferation in a dose-dependent manner. Amphiregulin significantly enhanced cell proliferation at 50 and 100 ng/mL. *p<0.01 vs. Control. (B) Amphiregulin was added in combination with PDGF to compare the proliferation of cells treated with PDGF only. Proliferation of cells treated with amphiregulin and PDGF did not show an add-on effect compared to the proliferation of cells treated with PDGF only. *p<0.01 vs. Control.
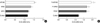
Fig. 3
(A) Effect of VEGF on the proliferation of human ASM cells. VEGF did not enhance cell proliferation at 10, 30, and 50 ng/mL. (B) Effect of anti-VEGF antibody and anti-VEGF R2 antibody on the PDGF-enhanced cell proliferation. Both 100 ng/mL of anti-VEGF antibody and/or 100 ng/mL of anti-VEGF R2 antibody did not influence PDGF-augmented cell proliferation. VEGF did not augment PDGF-enhanced cell proliferation when cells were treated with 10 ng/mL of VEGF in combination with PDGF. *p<0.01 vs. Control.
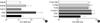
Fig. 4
Effect of IL-4 and amphiregulin on VEGF release by human ASM cells. (A) IL-4 (10 and 100 ng/mL) enhanced VEGF release in a dose-dependent manner. PDGF (20 ng/mL) also significantly increased VEGF release. *p<0.001 vs. Control, IL4-100 and PDGF. (B) IL-4 (10 and 100 ng/mL) increased PDGF-enhanced VEGF secretion. *p<0.01 vs. Control and PDGF. †p<0.01 vs. Control. (C) Amphiregulin (50, 100, and 150 ng/mL) did not augment VEGF secretion at all concentrations. *p<0.001 vs. Control, AR50, AR100 and AR150.
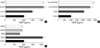
References
1. Elias JA, Lee CG, Zheng T, Ma B, Homer RJ, Zhu Z. New insights into the pathogenesis of asthma. J Clin Invest. 2003. 111:291–297.


2. Lazaar AL, Panettieri RA Jr. Airway smooth muscle: a modulator of airway remodeling in asthma. J Allergy Clin Immunol. 2005. 116:488–495.


3. Richter A, Puddicombe SM, Lordan JL, Bucchieri F, Wilson SJ, Djukanovic R, Dent G, Holgate ST, Davies DE. The contribution of interleukin (IL)-4 and IL-13 to the epithelial-mesenchymal trophic unit in asthma. Am J Respir Cell Mol Biol. 2001. 25:385–391.


4. Hirst SJ, Hallsworth MP, Peng Q, Lee TH. Selective induction of eotaxin release by interleukin-13 or interleukin-4 in human airway smooth muscle cells is synergistic with interleukin-1beta and is mediated by the interleukin-4 receptor alpha -chain. Am J Respir Crit Care Med. 2002. 165:1161–1171.
5. Kelly-Welch AE, Melo ME, Smith E, Ford AQ, Haudenschild C, Noben-Trauth N, Keegan AD. Complex role of the IL-4 receptor alpha in a murine model of airway inflammation: expression of the IL-4 receptor alpha on nonlymphoid cells of bone marrow origin contributes to severity of inflammation. J Immunol. 2004. 172:4545–4555.
6. Chatila TA. Interleukin-4 receptor signaling pathways in asthma pathogenesis. Trends Mol Med. 2004. 10:493–499.


7. Rankin JA, Picarella DE, Geba GP, Temann UA, Prasad B, DiCosmo B, Tarallo A, Stripp B, Whitsett J, Flavell RA. Phenotypic and physiologic characterization of transgenic mice expressing interleukin 4 in the lung: lymphocytic and eosinophilic inflammation without airway hyperreactivity. Proc Natl Acad Sci USA. 1996. 93:7821–7825.


8. Parameswaran K, Radford K, Fanat A, Stephen J, Bonnans C, Levy BD, Janssen LJ, Cox PG. Modulation of human airway smooth muscle migration by lipid mediators and Th-2 cytokines. Am J Respir Cell Mol Biol. 2007. 37:240–247.


9. Monroe JG, Haldar S, Prystowsky MB, Lammie P. Lymphokine regulation of inflammatory processes: interleukin-4 stimulates fibroblast proliferation. Clin Immunol Immunopathol. 1988. 49:292–298.


10. Vadiveloo PK, Stanton HR, Cochran FW, Hamilton JA. Interleukin-4 inhibits human smooth muscle cell proliferation. Artery. 1994. 21:161–181.
11. Toi M, Harris AL, Bicknell R. Interleukin-4 is a potent mitogen for capillary endothelium. Biochem Biophys Res Commun. 1991. 174:1287–1293.


12. Hawker KM, Johnson PR, Hughes JM, Black JL. Interleukin-4 inhibits mitogen-induced proliferation of human airway smooth muscle cells in culture. Am J Physiol. 1998. 275:L469–L477.
13. Estes ML, Iwasaki K, Jacobs BS, Barna BP. Interleukin-4 downregulates adult human astrocyte DNA synthesis and proliferation. Am J Pathol. 1993. 143:337–341.
14. Lee IY, Kim J, Ko EM, Jeoung EJ, Kwon YG, Choe J. Interleukin-4 inhibits the vascular endothelial growth factor- and basic fibroblast growth factor-induced angiogenesis in vitro. Mol Cells. 2002. 14:115–121.
15. Feltis BN, Wignarajah D, Zheng L, Ward C, Reid D, Harding R, Walters EH. Increased vascular endothelial growth factor and receptors: Relationship to angiogenesis in asthma. Am J Respir Crit Care Med. 2006. 173:1201–1207.
16. Wen FQ, Liu X, Manda W, Terasaki Y, Kobayashi T, Abe S, Fang Q, Ertl R, Manouilova L, Rennard SI. Th2 cytokine-enhanced and TGF-beta-enhanced vascular endothelial growth factor production by cultured human airway smooth muscle cells is attenuated by IFN-gamma and corticosteroids. J Allergy Clin Immunol. 2003. 111:1307–1318.
17. Faffe DS, Flynt L, Bourgeois K, Panettieri RA Jr, Shore SA. Interleukin-13 and interleukin-4 induce vascular endothelial growth factor release from airway smooth muscle cells: role of vascular endothelial growth factor genotype. Am J Respir Cell Mol Biol. 2006. 34:213–218.
18. Hong KH, Cho ML, Min SY, Shin YJ, Yoo SA, Choi JJ, Kim WU, Song SW, Cho CS. Effect of interleukin-4 on vascular endothelial growth factor production in rheumatoid synovial fibroblasts. Clin Exp Immunol. 2007. 147:573–579.


19. Voehringer D, Shinkai K, Locksley RM. Type 2 immunity reflects orchestrated recruitment of cells committed to IL-4 production. Immunity. 2004. 20:267–277.


20. Leigh R, Ellis R, Wattie JN, Hirota JA, Matthaei KI, Foster PS, O'Byrne PM, Inman MD. Type 2 cytokines in the pathogenesis of sustained airway dysfunction and airway remodeling in mice. Am J Respir Crit Care Med. 2004. 169:860–867.


21. Zhu Z, Homer RJ, Wang Z, Chen Q, Geba GP, Wang J, Zhang Y, Elias JA. Pulmonary expression of interleukin-13 causes inflammation, mucus hypersecretion, subepithelial fibrosis, physiologic abnormalities, and eotaxin production. J Clin Invest. 1999. 103:779–788.


22. Ma B, Blackburn MR, Lee CG, Homer RJ, Liu W, Flavell RA, Boyden L, Lifton RP, Sun CX, Young HW, Elias JA. Adenosine metabolism and murine strain-specific IL-4-induced inflammation, emphysema, and fibrosis. J Clin Invest. 2006. 116:1274–1283.


23. Bassa BV, Noh JW, Ganji SH, Shin MK, Roh DD, Kamanna VS. Lysophosphatidylcholine stimulates EGF receptor activation and mesangial cell proliferation: regulatory role of Src and PKC. Biochim Biophys Acta. 2007. 1771:1364–1371.


24. Toi M, Bicknell R, Harris AL. Inhibition of colon and breast carcinoma cell growth by interleukin-4. Cancer Res. 1992. 52:275–279.
25. Kim J, Cheon IS, Won YJ, Na HJ, Kim YM, Choe J. IL-4 inhibits cell cycle progression of human umbilical vein endothelial cells by affecting p53, p21(Waf1), cyclin D1, and cyclin E expression. Mol Cells. 2003. 16:92–96.
26. Barna BP, Estes ML, Pettay J, Iwasaki K, Zhou P, Barnett GH. Human astrocyte growth regulation. Interleukin-4 sensitivity and receptor expression. J Neuroimmunol. 1995. 60:75–81.


27. Saito A, Okazaki H, Sugawara I, Yamamoto K, Takizawa H. Potential action of IL-4 and IL-13 as fibrogenic factors on lung fibroblasts in vitro. Int Arch Allergy Immunol. 2003. 132:168–176.


28. Hua K, Deng J, Harp JB. Interleukin-4 inhibits platelet-derived growth factor-induced preadipocyte proliferation. Cytokine. 2004. 25:61–67.


29. Laporte JC, Moore PE, Baraldo S, Jouvin MH, Church TL, Schwartzman IN, Panettieri RA Jr, Kinet JP, Shore SA. Direct effects of interleukin-13 on signaling pathways for physiological responses in cultured human airway smooth muscle cells. Am J Respir Crit Care Med. 2001. 164:141–148.


30. Orsini MJ, Krymskaya VP, Eszterhas AJ, Benovic JL, Panettieri RA Jr, Penn RB. MAPK superfamily activation in human airway smooth muscle: mitogenesis requires prolonged p42/p44 activation. Am J Physiol. 1999. 277:L479–L488.
31. Kriebel P, Patel BK, Nelson SA, Grusby MJ, LaRochelle WJ. Consequences of STAT6 deletion on Sis/PDGF- and IL-4-induced proliferation and transcriptional activation in murine fibroblasts. Oncogene. 1999. 18:7294–7302.


32. Bosse Y, Stankova J, Rola-Pleszezynski M. The Th2 cytokines, IL-4 and IL-13, induce human bronchial smooth muscle proliferation. J Allergy Clin Immunol (abstract book). 2006. 117:S721.
33. Yu J, Moon A, Kim HR. Both platelet-derived growth factor receptor (PDGFR)-alpha and PDGFR-beta promote murine fibroblast cell migration. Biochem Biophys Res Commun. 2001. 282:697–700.
34. Hirst SJ, Barnes PJ, Twort CH. PDGF isoform-induced proliferation and receptor expression in human cultured airway smooth muscle cells. Am J Physiol. 1996. 270:L415–L428.


35. Li X, Wilson JW. Increased vascularity of the bronchial mucosa in mild asthma. Am J Respir Crit Care Med. 1997. 156:229–233.


36. Hoshino M, Nakamura Y, Hamid QA. Gene expression of vascular endothelial growth factor and its receptors and angiogenesis in bronchial asthma. J Allergy Clin Immunol. 2001. 107:1034–1038.


37. Kato M, Inazu T, Kawai Y, Masamura K, Yoshida M, Tanaka N, Miyamoto K, Miyamori I. Amphiregulin is a potent mitogen for the vascular smooth muscle cell line, A7r5. Biochem Biophys Res Commun. 2003. 301:1109–1115.


38. Falk A, Frisen J. Amphiregulin is a mitogen for adult neural stem cells. J Neurosci Res. 2002. 69:757–762.


39. Hurbin A, Dubrez L, Coll JL, Favrot MC. Inhibition of apoptosis by amphiregulin via an insulin-like growth factor-1 receptor-dependent pathway in non-small cell lung cancer cell lines. J Biol Chem. 2002. 277:49127–49133.


40. Okumura S, Sagara H, Fukuda T, Saito H, Okayama Y. Fcepsilon-RI-mediated amphiregulin production by human mast cells increases mucin gene expression in epithelial cells. J Allergy Clin Immunol. 2005. 115:272–279.
41. Wang SW, Oh CK, Cho SH, Hu G, Martin R, Demissie-Sanders S, Li K, Moyle M, Yao Z. Amphiregulin expression in human mast cells and its effect on the primary human lung fibroblasts. J Allergy Clin Immunol. 2005. 115:287–294.


42. Kumar RK, Herbert C, Foster PS. Expression of growth factors by airway epithelial cells in a model of chronic asthma: regulation and relationship to subepithelial fibrosis. Clin Exp Allergy. 2004. 34:567–575.


43. Kazi AS, Lotfi S, Goncharova EA, Tliba O, Amrani Y, Krymskaya VP, Lazaar AL. Vascular endothelial growth factor-induced secretion of fibronectin is ERK dependent. Am J Physiol Lung Cell Mol Physiol. 2004. 286:L539–L545.


44. Pype JL, Dupont LJ, Menten P, Van Coillie E, Opdenakker G, Van Damme J, Chung KF, Demedts MG, Verleden GM. Expression of monocyte chemotactic protein (MCP)-1, MCP-2, and MCP-3 by human airway smooth-muscle cells. Modulation by corticosteroids and T-helper 2 cytokines. Am J Respir Cell Mol Biol. 1999. 21:528–536.