Abstract
Venous air embolism (VAE) is the entrapment of air or medical gases into the venous system causing symptoms and signs of pulmonary vessel obstruction. The incidence of VAE during cesarean delivery ranges from 10 to 97% depending on surgical position or diagnostic tools, with a potential for life-threatening events. We reviewed extensive literatures regarding VAE in detail and herein described VAE during surgery including cesarean delivery from background and history to treatment and prevention. It is intended that present work will improve the understanding of VAE during surgery.
Venous air embolism (VAE) is the entrainment of air from ruptured veins to the central venous system producing embolism at the right heart or pulmonary artery. In addition, medical gases such as carbon dioxide (1, 2), nitrous oxide (3, 4), nitrogen (5, 6), and helium (7) can also cause or aggravate this condition.
Numerous studies on VAE have been reported in medical or surgical procedures and trauma. Neurosurgery performed in a sitting position has a high risk of VAE, with an incidence of about 76% in posterior fossa surgery (8) but 25% (8) or 7% in cervical laminectomy (9). Whereas, neurosurgical procedures in lateral, supine, or prone positions showed an incidence of 15% to 25% (10). Air embolism occurred in 30% cases of total hip replacement surgery (11). During laparoscopic cholecystectomy, the incidence is reported to be 69% (12). Also, VAE cases associated with central venous catheters (13), endoscopic procedures (14), or inadvertent injection of air as contrast media (15) have been recently reported. Lung (16) or abdominal trauma (17) can also induce VAE.
Cesarean section has also been associated with VAE. The first fatal case of VAE in association with pregnancy was reported in 1850 by Cormack (18). In 1947, Durant et al. (19) indicated for the first time that the uterine sinuses are theoretically vulnerable to the entrance of air, especially in the presence of placenta previa or during manual extraction of the placenta. Until recently, not many cases of massive VAE during cesarean section with devastating morbidity or mortality have been reported (20-28). The incidence of VAE during cesarean delivery ranges from 10 to 97% depending on surgical position or diagnostic tools (Table 1) (29-35). However, it is reported that VAE is responsible for about 1 percent of all maternal deaths (36).
A vascular access and a gradient between the injury site and the right heart are prerequisites for VAE to occur (37). Factors that determine the severity of VAE include the rate, volume, and duration of air entrapment, patient's position at the time of VAE occurrence, the size of the patient's pulmonary vasculature, type of gas exposure, and the patient's general condition (18, 19). Gravitational gradients from the venous portal of entrance to the right side of the heart as small as 5 cm have been reported to produce entrainment of enough large amounts of air that may embolize the venous pulmonary circulation (10).
The filtering action of pulmonary vessels can protect the systemic and coronary circulation from air emboli derived from the venous circulation (38). Butler and Hills (39) in their studies on dogs reported that the filtration of venous bubbles by the pulmonary vasculature was completed when the air infusion rates were maintained below a threshold value of 0.30 mL/kg/min. Nelson (40) assumed that 500 to 600 mL of air administered in a single rapid injection would be uniformly fatal to human (7 mL/kg) and the required fatal amount would be half (3 mL/kg) if the introduction of air was prolonged for as much as one or two minutes.
VAE may lead to trapping of air bubbles in the pulmonary vessels producing a broad array of physiological changes (Table 2). Gas exchange abnormalities such as decreased PaO2, increased PaCO2, and an increase in the alveolar-arterial oxygen difference can occur (41, 42). Ventilation-perfusion (VA/Q) mismatch is the main cause of these abnormalities (43, 44). Increased regions of high VA/Q contribute to the increase in PaCO2, whereas, decreased PaO2 results from changes in the intrapulmonary shunt, mixed venous oxygen saturation, and a shift in the distribution of pulmonary blood flow to regions which are relatively overperfused and underventilated (low VA/Q) (41). Entrapment of venous bubbles in the pulmonary microcirculation may lead to cellular injury and lung edema due to the release of vasoactive mediators (45, 46) and increased microvascular permeability characterized by increased flow of protein-rich lymph (47). This blocking effect of air bubbles and pulmonary artery vasoconstriction due to vasoactive mediators may result in acute cor pulmonale and right ventricular decompensation secondary to the acute rise in right ventricular afterload. Consequently, the preloading of left ventricle and cardiac output can be severely diminished, followed by cardiac arrest (18).
VAE may manifest as chest tightness or short breath with or without hypotension or decreased oxygen saturation in conscious patients undergoing cesarean section under regional anesthesia. In unconscious patients, severe VAE may manifest as acute decrease in end-tidal carbon dioxide (ETCO2), increase in end-tidal nitrogen (ETN2), hypotension or hypoxia inexplicable by deep anesthesia or hypovolemia. However, other conditions having similar manifestations, such as amniotic fluid embolism, pulmonary thromboembolism, pneumothorax, bronchospasm, pulmonary edema, or myocardial infarction, should also be differentiated.
There has been advancement in monitoring technology contributing to the prevention and treatment of VAE. The ideal monitoring device for VAE in parturients undergoing cesarean section should be sensitive as well as specific for severe or hemodynamically unstable VAE and practically available. Although there is no such ideal monitoring device yet, the proper selection of a monitoring device considering the operation and medical condition of the patient is helpful to detect and diagnose VAE in time for prevention and treatment. In Table 3, each monitoring system is listed in order of sensitivity, their characteristics summarized and compared.
Transesophageal echocardiography (TEE) is relatively invasive but is the most sensitive device for monitoring air embolism. TEE enables to detect a bolus dose of air as small as 0.02 mL/kg (48). Because it is easy to secure the probe at the selected position for a long period, continuous images can be obtained for a relatively long time without intervention compared to transthoracic echocardiogram (TTE). TTE, however, has the advantage of noninvasive examination. TEE and TTE have the advantage of being able to detect paradoxical air embolism, intracardiac defects and evaluate intravascular volume status (48, 49). The echocardiogram is useful to identify the route of air emboli entrained and classify severity of air embolism (Table 4, Fig. 1) (12). In massive air embolism detected by TTE, hyperdynamic left ventricular function with a dilated right ventricle, dilated right ventricular outflow tract and pulmonary artery, and decreased right ventricular function were observed, but no air was found initially (13). In repeated TTE imaging, a large influx of air filling the right heart and pulmonary artery was demonstrated (13). However, the majority of VAE cases in cesarean section are not fatal and have no adverse sequelae despite its high incidence (29-36). TEE is less available than other monitoring devices since it is expensive and requires a well-trained and certified echocardiographer or anesthesiologist for proper and continuous monitoring (50).
Precordial Doppler ultrasound is considered efficacious and favored for the surveillance of air embolism for its simplicity, noninvasiveness, and being almost as sensitive as TEE (51). It can detect a bolus dose of air as small as 0.05 mL/kg with immediate alterations in the ultrasonic pattern (48). Because this device produces a sound resembling a "washing machine" or "drum", it is easy to distinguish among other alarm sounds and to monitor air embolism while tending to other duties of patient care. It is worth pointing out that a positive Doppler signal does not always indicate clinical significance because hemodynamically significant air embolisms during caesarean section are rare and audible Doppler signals are sometimes false-positive (35).
Proper positioning of the precordial Doppler probe over the anterior chest wall is crucial for early and exact detection of venous air embolism, especially in obese patients or parturients (52). To increase the sensitivity and specificity of precordial Doppler, the right sternal border over the right heart has been recommended as the ideal position of the Doppler probe. However, placing the probe over the left sternal border has also been used (52). Normal saline or carbon dioxide may be injected to verify proper positioning of the precordial Doppler probe.
The pulmonary artery catheter is less sensitive than TEE, precordial Doppler ultrasound, or gas-sensing methods but the most invasive way among the monitoring devices mentioned in related articles. The pulmonary artery catheter is capable of detecting as little as 0.5 mL/kg of air in 80% of bolus injections when pulmonary artery pressure exceeding baseline by 25% is defined as a positive sign of VAE (53). Although the pulmonary artery pressure is less sensitive than ETCO2, it changes earlier than ETCO2, when air embolism occurs as much as volumes of 1.5 mL/kg (53). The pulmonary artery catheter has limitations in detecting VAE in normal patients undergoing elective cesarean section due to its invasiveness. However, in patients with other complicated indications requiring its use, the pulmonary artery catheter can provide useful information such as pulmonary artery hypertension, low cardiac output and low mixed venous saturation that manifests in severe or fatal VAE (53).
The most sensitive method of VAE detection among gassensing methods is the ETN2 monitor. Increased ETN2 in a patient ventilated with denitrogenated gas has been considered as a specific sign of venous air embolism (54). ETN2 monitor is capable of detecting as little as 0.25±0.5 mL/kg of air (53, 55) and increases rapidly after an experimental VAE is large enough to cause minimal changes in blood pressure (56). The time to maximal change in ETN2 (32±8 sec) was 30-60 sec earlier than that of pulmonary artery catheters and ETCO2 (53). The mass spectrometer used in the study by Matjasko et al. (53) had a nitrogen sensitivity of 0.01%, in contrast to the 0.1% in clinically used mass spectrometers. Emission spectrometry can also be used to detect increased ETN2 levels indicative of venous air embolism as an alternative to mass spectrometers, and may be a more sensitive detector (0.1 mL/kg) (54).
ETCO2 is a convenient and useful tool to detect VAE because of its common use in the operating room. Although ETCO2 is as sensitive as ETN2, it changes later than ETN2. A change in ETCO2 is considered significant and can be detected if it drops 0.2% (53) or 2 mmHg (57) below the baseline. Despite its clinically practical advantages, ETCO2 monitoring has drawbacks. It can be affected by other factors such as airway obstruction, systemic hypotension and reduced cardiac output which decrease specificity and reliability. Also, because secure airway and ventilation are required for reliable gas-sensing monitoring, gas-sensing methods like ETN2 and ETCO2 cannot be applied to patients undergoing regional anesthesia for cesarean section.
These monitoring systems display the late findings of VAE, detecting the complications of VAE and severe physiologic deterioration caused by VA/Q mismatch, right and/or left heart failure, or paradoxical arterial air embolism (13, 34, 58). Therefore, these methods are not suitable for the early detection of VAE, especially in patients under general anesthesia without N2O, and negative findings do not guarantee the absence of VAE.
The ECG changes during cesarean delivery were known to be nonspecific signs of VAE probably caused by hormonal influence, tachycardia, or patient position (58, 59). However, these monitoring systems are available in the operating room and are familiar to most anesthesiologists. ECG changes such as ST depression or elevation, widened QRS complex, premature ventricular contraction or T wave change, are generally shown as the consequence of severe physiologic deterioration in large or rapid air entrainment (13, 60).
Pulse oximetry has low sensitivity for detecting VAE but it is also important for patient safety. Williamson et al. (60) reported the first primary means for detection of VAE is a fall in ETCO2 (34% of 38 VAE cases) and the second one is desaturation (26% of 38 VAE cases).
It has been accepted that the 15° left lateral tilt position may increase the risk of VAE during cesarean section, due to a subatmospheric venous pressure gradient between the right atrium and uterine incision (31). In addition, a trendelenburg position or exteriorization may augment the pressure gradient and increase the risk of VAE, because the right atrium is positioned below the uterus (24, 37). A strategy to increase right atrium pressure (RAP) is rational when its physiologic effect is considered carefully. To reverse the subatmospheric venous pressure gradient, the effect of patient positioning has been investigated. The effect of a 5-10° reverse trendelenburg position on the incidence of VAE is controversial. In a case-control study conducted on 100 healthy patients undergoing cesarean section, there was no difference in incidence between the supine and 5-10° reverse trendelenburg position with a 15° lateral tilt (31). However, other investigators reported that the application of a 5° reverse trendelenburg position dramatically decreased the incidence of VAE from 44% to 1% (32).
PEEP is known to be effective in preventing VAE by increasing venous pressure at the level of incision. However, PEEP does not decrease the incidence of VAE but rather have adverse effects on the cardiovascular system such as lowering cardiac output and increasing pulmonary vascular resistance in patients in sitting positions for neurosurgery (61). However, in a supine position, PEEP does increase pulmonary vascular resistance and the incidence of paradoxical air embolism although it may lower cardiac index (61). Because PEEP up to 10 cmH2O does not alter the pulmonary arterial wedge pressure (PAWP) - RAP difference, it can be safely applied without the concern of paradoxical arterial embolism (62). Although applying PEEP up to 10 cmH2O may not be harmful in patients undergoing cesarean delivery, no benefit to overcome a potential harm has been found yet. Furthermore, sudden release of PEEP to 0 mmHg can lead to VAE and the Valsalva maneuver can increase the incidence of paradoxical arterial embolism (63).
MAST suit can increase RAP dramatically, inflated up to 40 mmHg in the lower compartment and 30 mmHg in the abdominal compartment (64) and its effect is potentiated with PEEP (64, 65). However, MAST suits may not be effective because the increase in filling pressure is only transient in half of the patients (66, 67). In addition, MAST suits may accompany complications such as nerve injury or ischemia in lower extremities and decreased vital capacity (66, 67). Due to these collateral complications, use of MAST is discouraged in cesarean section.
The goals of management when severe VAE is suspected or manifested, are prevention of further gas entry, removal of entrained gas, and maintenance of hemodynamical stability.
Strategies accepted to minimize the entry of gas include flooding the surgical field and increasing RAP. When VAE is diagnosed and hemodynamic deterioration is manifested, the anesthesiologist has to inform the surgeon and the surgeon should flood the surgical field with fluid such as normal saline and eliminate any origin of gas entry if possible. It has been recommended to reposition the patient thus to increase RAP and to trap the air in the right atrium. In patients undergoing cesarean section, placing the patient in a reverse Trendelenburg position mandates close monitoring, because the position can aggravate hemodynamic instability. Placing the patient in a Trendelenburg position for central venous catheterization or hemodynamic support may increase the negative pressure gradient thereby increasing gas influx even further. Therefore, the surgical field should be flooded before positioning. Placing the patient in a left lateral recumbent position may be helpful for trapping and aspirating gas entrained in the right atrium and preventing and relieving the air-lock in the right heart (68). Geissler et al. (69), however, demonstrated that VAEs of lesser degree (2.5 mL/kg at a rate of 5 mL/sec) did not result in significant differences of hemodynamics and outcome between the right lateral recumbent position and left lateral recumbent position. Despite these results, groups in the left lateral position demonstrated repositioning of the VAE to the right atrium and tricuspid area on the echocardiogram (69).
When VAE is suspected, nitrous oxide should be discontinued because ventilation with nitrous oxide expands pulmonary vascular bubbles and increases the residual time of VAE leading to aggravation of the hemodynamic consequences (65, 70-73). Also, the patient should be ventilated with 100% oxygen in order to wash out entrained air and to improve V/Q mismatch and hypoxemia. Fortunately, the development of various anesthetic agents has discouraged the use of nitrous oxide, especially in procedures with a high risk of VAE.
If a central venous catheter or pulmonary artery catheter is placed, the trapped air in right atrium (RA), right ventricle (RV), or right ventricular outflow tract (RVOT) can be aspirated. In patients with risk factors for VAE and massive hemorrhage, central catheterization prior to induction should be considered. When the central venous catheter is properly positioned, up to 50% of the entrained air can be aspirated and using a multi-orifice catheter is helpful (74). The proper position of the catheter tip is 2 cm below the superior venalright atrial junction and can be verified by chest radiography or ECG attached to the catheter (75).
Hemodynamics may be deteriorated by right ventricular failure caused by the air-lock of entrapped air bubbles in the right heart, pulmonary artery vasoconstriction due to vasoactive mediators, and subsequent left ventricular failure due to severely diminished left ventricular filling. Maintenance of coronary perfusion pressure is essential to prevent further deterioration of the right ventricle and to improve right ventricular function (76). Norepinephrine can significantly improve ventricular performance without constricting either the pulmonary or renal circulation (77, 78). Use of vasodilator for the treatment of increased pulmonary vasoconstriction and reduction of right ventricular afterload can be attempted with care when hypotension is not present (76). Prostaglandin analogue or phosphodiesterase inhibitors may be considered to treat severe pulmonary hypertension and to reduce right ventricular afterload (79). Milrinone or dobutamine may provide inotropic support and decrease the right ventricular afterload (80).
Severe and fatal VAE can lead to a cardiac arrest following dysrhythmias such as premature ventricular complex, widened QRS wave, or ventricular tachycardia. Cardiopulmonary resuscitation should be performed with drugs such as epinephrine or atropine according to the Advanced Cardiac Life Support protocol. External cardiac compression may contribute to the breakup of the locked air and its transit into pulmonary circulation (38, 39).
Hyperbaric oxygen therapy has been reported to be useful in VAE, especially for neurologic deficits caused by cerebral air embolism (81-83). The effect of hyperbaric oxygen therapy
is based upon reduction of the bubble size by accelerated resorption of nitrogen and increased delivery of oxygen to ischemic brain tissue (83).
We reviewed extensive literatures regarding VAE in detail and herein described VAE during surgery including cesarean delivery from background and history to treatment and prevention. It is intended that present work will improve the understanding of VAE during surgery, especially cesarean delivery.
Figures and Tables
Fig. 1
Venous air embolism detected by transesophageal echocardiography (mid-esophageal four-chamber view) during cesarean delivery in our recent study. (A) grade I, (B) grade II, (C) grade III, (D) grade IV.
RA, right atrium; RV, right ventricle; LA, left atrium; LV, left ventricle.
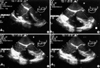
References
1. Mommerot A, Perrault LP. Carbon dioxide embolism induced by endoscopic saphenous vein harvesting during coronary artery bypass grafting. J Thorac Cardiovasc Surg. 2006. 132:1502.


2. Staffieri F, Lacitignola L, De Siena R, Crovace A. A case of spontaneous venous embolism with carbon dioxide during laparoscopic surgery in a pig. Vet Anaesth Analg. 2007. 34:63–66.


3. Kama NA. Influence of nitrous oxide anesthesia on venous gas embolism with carbon dioxide and helium during pneumoperitoneum. Surg Endosc. 2001. 15:1237–1238.


4. Cottin V, Viale JP, Bouffard Y, Delafosse B. Severe nitrous oxide embolism during venous stripping. Intensive Care Med. 1997. 23:1287–1288.


5. Boussuges A, Blanc F, Carturan D. Hemodynamic changes induced by recreational scuba diving. Chest. 2006. 129:1337–1343.


6. Risberg J, Englund M, Aanderud L, Eftedal O, Flook V, Thorsen E. Venous gas embolism in chamber attendants after hyperbaric exposure. Undersea Hyperb Med. 2004. 31:417–429.
7. Mitchell SJ, Benson M, Vadlamudi L, Miller P. Cerebral arterial gas embolism by helium: an unusual case successfully treated with hyperbaric oxygen and lidocaine. Ann Emerg Med. 2000. 35:300–303.


8. Papadopoulos G, Kuhly P, Brock M, Rudolph KH, Link J, Eyrich K. Venous and paradoxical air embolism in the sitting position. A prospective study with transoesphageal echocardiography. Acta Neurochir (wien). 1994. 126:140–143.
9. Losasso TJ, Muzzi DA, Dietz NM, Cucchiara RF. Fifty percent nitrous oxide does not increase the risk of venous air embolism in neurosurgical patients operated upon in the sitting position. Anesthesiology. 1992. 77:21–30.


10. Albin MS, Carroll RG, Maroon JC. Clinical considerations concerning detection of venous air embolism. Neurosurgery. 1978. 3:380–384.


12. Schmandra TC, Mierdl S, Bauer H, Gutt C, Hanisch E. Transoesophageal echocardiography shows high risk of gas embolism during laparoscopic hepatic resection under carbon dioxide pneumoperitoneum. Br J Surg. 2002. 89:870–876.


13. Maddukuri P, Downey BC, Blander JA, Pandian NG, Patel AG. Echocardiographic diagnosis of air embolism associated with central venous catheter placement: case report and review of the literature. Echocardiography. 2006. 23:315–318.


14. Rabe C, Balta Z, Wüllner U, Heller J, Hammerstingl C, Tiemann K, Sommer T, Schepke M, Fischer HP, Sauerbruch T. Biliary metal stents and air embolism: a note of caution. Endoscopy. 2006. 38:648–650.


15. Cuvelier A, Muir JF. Images in clinical medicine: venous air embolism. N Engl J Med. 2006. 354:e26.
17. Southern DA, Arramovic J, Fitzsimmons K. Air embolism following abdominal stab-wound. Anaesth Intensive Care. 1996. 24:623.
18. Cormack JR. The entrance of air by the open mouths of the veins, considered as cause of danger and death after parturition. London Med J. 1850. 2:928.
20. Waldrop GS. Fatal air embolism during term labor and the puerperium. Obstet Gynecol. 1953. 1:454–459.
21. Merrill DG, Samuels SI, Silverberg GD. Venous air embolism of uncertain etiology. Anesth Analg. 1982. 61:65–66.


22. Younker D, Rodriguez V, Kavanagh J. Massive air embolism during cesarean section. Anesthesiology. 1986. 65:77–79.


23. Fong J, Gadalla F, Gimbel AA. Precordial Doppler diagnosis of haemodynamically compromising air embolism during caesarean section. Can J Anaesth. 1990. 37:262–264.


24. Lang S. Precordial Doppler diagnosis of haemodynamically compromising air embolism during caesarean section. Can J Anaesth. 1991. 38:255–256.


25. Mushkat Y, Luxman D, Nachum Z, David MP, Melamed Y. Gas embolism complicating obstetric or gynecologic procedures. Case reports and review of the literature. Eur J Obstet Gynecol Reprod Biol. 1995. 63:97–103.


26. Epps SN, Robbins AJ, Marx GF. Complete recovery after near-fatal venous air embolism during cesarean section. Int J Obstet Anesth. 1998. 7:131–133.


27. Kostash MA, Mensink F. Lethal air embolism during cesarean delivery for placenta previa. Anesthesiology. 2002. 96:753–754.


28. Nims M, Hallonguist H, Camann W. Coronary arterial air embolus occurring during cesarean delivery. Int J Obstet Anesth. 2006. 15:166–169.


29. Malinow AM, Naulty JS, Hunt CO, Datta S, Ostheimer GW. Precordial ultrasonic monitoring during cesarean delivery. Anesthesiology. 1987. 66:816–819.


30. Fong J, Gadalla F, Pierri MK, Druzin M. Are Doppler-detected emboli during cesarean section air emboli? Anesth Analg. 1990. 71:254–257.
31. Karuparthy VR, Downing JW, Husain FJ, Knape KG, Blanchard J, Solomon D. Incidence of venous air embolism during cesarean section is unchanged by the use of a 5 to 10o head-up tilt. Anesth Analg. 1989. 69:620–623.
32. Fong J, Gadalla F, Druzin M. Venous emboli occurring caesarean section: the effect of patient position. Can J Anaesth. 1991. 38:191–195.
33. Handler JS, Bromage PR. Venous air embolism during cesarean delivery. Reg Anesth. 1990. 15:170–173.
34. Vartikar JV, Johnson MD, Datta S. Precordial Doppler monitoring and pulse oximetry during cesarean delivery: detection of venous air embolism. Reg Anesth. 1989. 14:145–148.
35. Lew TW, Tay DH, Thomas E. Venous air embolism during cesarean section: more common than previously thought. Anesth Analg. 1993. 77:448–452.


36. Kaunitz AM, Hughes JM, Grimes DA, Smith JC, Rochat RW, Kafrissen ME. Causes of maternal mortality in the united states. Obstet Gynecol. 1985. 65:605–612.


37. Maroon JC, Goodman JM, Horner TG, Campbell RL. Detection of minute venous air emboli with ultrasound. Surg Gynecol Obstet. 1968. 127:1236–1238.
39. Butler BD, Hills BA. Transpulmonary passage of venous air emboli. J Appl Physiol. 1985. 59:543–547.


40. Nelson PK. Pulmonary gas embolism in pregnancy and the puerperium. Obstet Gynecol Surv. 1960. 15:449–481.


41. Hlastala MP, Bobertson HT, Ross BK. Gas exchange abnormalities produced by venous gas emboli. Respir Physiol. 1979. 36:1–17.


42. Deem S, McKinney S, Polissar NL, Hedges RG, Swenson ER. Hemodilution during venous gas embolization improves gas exchange, without altering V(A)/Q or pulmonary blood flow distributions. Anesthesiology. 1999. 91:1861–1872.


43. Souders JE. Pulmonary air embolism. J Clin Monit Comput. 2000. 16:375–383.
44. Verstappen FT, Bernards JA, Kreuzer F. Effects of pulmonary gas embolism on circulation and respiration in the dog. IV. Origin of arterial hypoxemia during pulmonary gas embolism. Pflugers Arch. 1977. 370:71–75.
45. Wang D, Ki MH, Hsu K, Shen CY, Chen HI, Lin YC. Air embolism induced lung injury in isolated rat lungs. J Appl Physiol. 1992. 72:1235–1242.
46. Albertine KH, Wiener-Kronish JP, Koike K, Staub NC. Quantification of damage by air emboli to lung microvessels in anesthetized sheep. J Appl Physiol. 1984. 57:1360–1368.


47. Ohkuda K, Nakahara K, Binder A, Staub NC. Venous air emboli in sheep: reversible increase in lung microvascular permeability. J Appl Physiol. 1981. 51:887–894.


48. Furuya H, Suzuki T, Okumura F, Kishi Y, Uefuji T. Detection of air embolism by transesophageal echocardiography. Anesthesiology. 1983. 58:124–129.


49. Milani RV, Lavie CJ, Gilliland YE, Cassidy MM, Bernal JA. Overview of transesophageal echocardiography for the chest physician. Chest. 2003. 124:1081–1089.


50. Gibby GL. Precordial Doppler is not obsolete for venous air embolism monitoring. Anesthesiology. 1988. 68:829.


51. Gildenberg PL, O'Brien RP, Britt WJ, Frost EA. The efficacy of Doppler monitoring for the detection of venous air embolism. J Neurosurg. 1981. 54:75–78.


52. Schubert A, Deogaonkar A, Drummond JC. Precordial Doppler probe placement for optimal detection of venous air embolism during craniotomy. Anesth Analg. 2006. 102:1543–1547.


53. Matjasko J, Petrozza P, Mackenzie CF. Sensitivity of end-tidal nitrogen in venous air embolism detection in dogs. Anesthesiology. 1985. 63:418–423.


54. Russell GB, Richard RB, Snider MT. Detection of venous air embolism in dogs by emission spectrometry. J Clin Monit. 1990. 6:18–23.


55. Drummond JC, Prutow RJ, Scheller MS. A comparison of the sensitivity of pulmonary artery pressure, end-tidal carbon dioxide, and end-tidal nitrogen in the detection of venous air embolism in the dog. Anesth Analg. 1985. 64:668–692.


56. Matjasko MJ, Hellman J, Mackenzie CF. Venous air embolism, hypotension, and end-tidal nitrogen. Neurosurgery. 1987. 21:378–382.


57. Losasso TJ, Black S, Muzzi DA, Michenfelder JD, Cucchiara RF. Detection and hemodynamic consequences of venous air embolism. Does nitrous oxide make a difference? Anesthesiology. 1992. 77:148–152.
58. Burton A, Camann W. Electrocardiographic changes during cesarean section: a review. Int J Obstet Anesth. 1996. 5:47–53.


59. Mathew JP, Fleisher LA, Rinehouse JA, Sevarino FB, Sinatra RS, Nelson AH, Prokop EK, Rosenbaum SH. ST segment depression during labor and delivery. Anesthesiology. 1992. 77:635–641.


60. Williamson JA, Helps SC, Westhorpe RN, Mackay P. Crisis management during anaesthesia: embolism. Qual Saf Health Care. 2005. 14:e17.


61. Giebler R, Kollenberg B, Pohlen G, Peters J. Effect of positive end-expiratory pressure on the incidence of venous air embolism and on the cardiovascular response to the sitting position during neurosurgery. Br J Anaesth. 1998. 80:30–35.


62. Zasslow MA, Pearl RG, Larson CP, Silverberg G, Shuer LF. PEEP does not affect left atrial-right atrial pressure difference in neurosurgical patients. Anesthesiology. 1988. 68:760–763.


63. Clarke NR, Timperley J, Kelion AD, Banning AP. Transthoracic echocardiography using second harmonic imaging with Valsalva manoeuvre for the detection of right to left shunts. Eur J Echocardiogr. 2004. 5:176–181.


64. Meyer PG, Cuttaree H, Charron B, Jarreau MM, Perie AC, Sainte-Rose C. Prevention of venous air embolism in paediatric neurosur-gical procedures performed in the sitting position by combined use of MAST suit and PEEP. Br J Anaesth. 1994. 73:795–800.


65. Butler BD, Conkin J, Luehr S. Pulmonary hemodynamics, extravascular lung water and residual gas bubbles following low dose venous gas embolism in dogs. Aviat Space Environ Med. 1989. 60:1178–1182.
66. Martin JT. Neuroanesthetic adjuncts for surgery in the sitting position. II. The antigravity suit. Anesth Analg. 1970. 49:588–593.
67. Tinker JH, Vandam LD. How effective is the G suit in neurosurgical operations? Anesthesiology. 1972. 36:609–611.


68. Oppenheimer MJ, Durant TM, Lynch P. Body position in relation to venous air embolism and the associated cardiovascular-respiratory changes. Am J Med Sci. 1953. 225:362–373.


69. Geissler HJ, Allen SJ, Mehlhorn U, Davis KL, Morris WP, Butler BD. Effect of body repositioning after venous air embolism. An echocardiographic study. Anesthesiology. 1997. 86:710–717.
70. Butler BD, Luehr S, Katz J. Venous gas embolism: time course of residual pulmonary intravascular bubbles. Undersea Biomed Res. 1989. 16:21–29.
71. Kyttä J, Tanskanen P, Randell T. Comparison of the effects of controlled ventilation with 100% oxygen, 50% oxygen in nitrogen, and 50% oxygen in nitrous oxide on responses to venous air embolism in pigs. Br J Anaesth. 1996. 77:658–661.


72. Roberts MW, Mathiesen KA, Ho HS, Wolfe BM. Cardiopulmonary responses to intravenous infusion of soluble and relatively insoluble gases. Surg Endosc. 1997. 11:341–346.


73. Knüttgen D, Stölzle U, Köning W, Müller MR, Doehn M. Air embolism in the sitting position. Oxygen/nitrogen versus oxygen/laughing gas. Anaesthesist. 1989. 38:490–497.
74. Adornato DC, Gildenberg PL, Ferrario CM, Smart J, Frost EA. Pathophysiology of intravenous air embolism in dogs. Anesthesiology. 1978. 49:120–127.


75. Bowdle TA, Artru AA. Positioning the air aspiration pulmonary artery catheter introducer sheath by intravascular electrocardiography. Anesthesiology. 1988. 69:276–279.


76. Calvin JE Jr. Acute right heart failure: pathophysiology, recognition, and pharmacological management. J Cardiothorac Vasc Anesth. 1991. 5:507–513.


77. Vlahakes GJ, Turley K, Hoffman JI. The pathophysiology of failure in acute right ventricular hypertension: hemodynamic and biochemical correlations. Circulation. 1981. 63:87–95.


78. Angle MR, Molloy DW, Penner B, Jones D, Prewitt RM. The cardiopulmonary and renal hemodynamic effects of norepinephrine in canine pulmonary embolism. Chest. 1989. 95:1333–1337.


79. Monrad ES, Baim DS, Smith HS, Lanoue AS. Milrinone, dobutamine, and nitroprusside: comparative effects on hemodynamics and myocardial energetics in patients with severe congestive heart failure. Circulation. 1986. 73:III168–III174.
80. Jardin F, Genevray B, Brun-Ney D, Margairaz A. Dobutamine: a hemodynamic evaluation in pulmonary embolism shock. Crit Care Med. 1985. 13:1009–1012.


81. Nayagam J, Ho KM, Liang J. Fatal systemic air embolism during endoscopic retrograde cholangio-pancreatography. Anaesth Intensive Care. 2004. 32:260–264.


82. Murphy BP, Harford FJ, Cramer FS. Cerebral air embolism resulting from invasive medical procedures. Treatment with hyperbaric oxygen. Ann Surg. 1985. 201:242–245.


83. Russell GB, Snider MT, Richard RB, Loomis JL. Venous air emboli with 15N2: pulmonary excretion and physiologic responses in dogs. Undersea Biomed Res. 1991. 18:37–45.