Abstract
We performed this study to investigate the feature of rejection in porcine-to-rat corneal orthotopic transplantation and to evaluate the effect of cyclosporine and mycophenolate on the xeno-rejection. Orthotopic corneal transplantation was done at 91 Sprague-Dawley rats, and they were divided into 10 groups based on the combination of immunosuppressants including dexamethasone, cyclosporine, and mycophenolate mofetil. Graft survival was analyzed and grafted eyes were examined with Hematoxylin & Eosin and CD4 or CD8 staining. Enzyme-linked immunosorbent assays were done for interleukin-2 (IL-2), IL-4, IL-5, IL-10, and interferon (IFN)-γ in cornea, lacrimal gland, and cervical lymph nodes. The longest median survival of the immune suppressant group was 11.00±1.96 days, which showed no statistical differences compared with that of control (8.00±1.52 days). The neutrophils were prominent in the early phase but soon gave way to the monocytes. The number of CD8+ cells was higher than that of CD4+ cells. IL-2 and IFN-γ markedly increased at 10 to13 days in cornea, lacrimal glands, and cervical lymph nodes, which showed a decrease with immunosuppressants except in the cornea. In conclusion, cyclosporine and mycophenolate could not prevent the rejection in porcine to rat orthotopic corneal xenograft associated with infiltraton of CD8+ and innate immune cells.
Because of the deficiency in availability of donor organs, tissues harvested from suitable xenogeneic sources may provide reasonable alternatives for transplantation. There are important immunologic concerns that require precise understanding of the relevant immune reactions prior to successful xenotransplantation. Recently, a Gal knock out pig has been developed which may help with the problems related to hyperacute rejection of xenografts; the feasibility of clinical use of such porcine xenografts is currently under investigation. The cornea, an avascular organ, has an immune-privileged environment and might be regarded as less immungeneic (1, 2). The naturally existing antibodies, presumed to be the main obstacle for transplantation in other organs, might not have an access directly to the graft in the cornea (3). Blockage of xenonatural antibodies have had little effect on graft survival in the cornea; therefore, the role of antibodies may be limited in cornea xenotransplantation (4). By contrast, in allografts, CD4+ T cells have been recognized as the main cause of the corneal rejection; so the suppression of these cells generally ensures long-term graft acceptance for the allocorneal graft (5). However, the exact mechanism involved in rejection of a corneal xenograft remains still unclear. Moreover, the immune system varies in every species, and each has specific antigen recognition. One report has suggested that T cells are primarily involved in xeno-corneal graft rejection in a mouse model; however, there is an another evidence of non-T cell populations in other xenografts as well as in inflamed cornea (4, 6, 7).
We have focused our investigation on the feasibility of using porcine cornea for a xenograft; this is because pigs are physiologically similar to human, have excellent breeding characteristics, and have similar refractive properties to humans. In addition, porcine transplantation has been widely investigated in other fields of medicine (8). Therefore, we investigated the full features of cellular responses in orthotopic porcine-to-rat corneal transplantation by evaluating the effect of immune suppressants on the survival of the graft and by analyzing the changes of cytokine produced after transplantation.
All procedures used in this study conformed to the principles embodied in the ARVO Statement for the Use of Animals in Ophthalmic and Vision Research, and the protocol was approved by the Research Ethics Committee at Seoul National University Hospital. Ninety-one fresh porcine corneas were obtained within six hours of death and were used for this study. In addition, 91 Sprague-Dawley (10 week old) rats were divided into 10 groups: 21 rats in control (Group 1), and the others were divided according to the immune suppressant regimen used after surgery: dexamethasone (DEXA) 2 mg/kg (Group 2, n=5), dexa 2 mg/kg with cyclosporine A (CsA, Sandimum®, Novatis, Basel, Switzerland) 10 mg/kg (Group 3, n=5), dexa 1 mg/kg (Group 4, n=7), dexa 1 mg/ kg with CsA 5 mg/kg (Group 5, n=7), DEXA 1 mg/kg with CsA 2.5 mg/kg (Group 6, n=6), dexa 1 mg/kg with CsA 1.25 mg/kg (Group 7, n=7), dexa 1 mg/kg with CsA 1.25 mg/kg and mycophenolate mofetil (MMF, CellCept®, Roche, Florence, SC, U.S.A.) 6 mg/kg (Group 8, n=8), dexa 1 mg/ kg with CsA 1.25 mg/kg and MMF 1.25 mg/kg (Group 9, n=7), and the final Group 10 was treated daily with CSA 2.5 mg/kg peritoneally for a week prior to the surgery (n= 18). Then 0.1 cc of low molecular weight dextran sulfate (MW 5000, 1 mg/mL) was injected intracamerally after completion of surgery; they were then treated with DEXA 1 mg/kg, CsA 1.25 mg/kg, and MMF 1.25 mg/kg. All of the immunosuppressant medications were applied after surgery and injected intramuscularly once a day for two weeks regardless of the group. Animals were anesthetized by intramuscular injection of a combination of 10 mg/kg solution of Zoletil (Virbac, Carros, France) and 2 mg/kg of Xylazine (Bayer, Levorkusen, German). The cornea was fixed firmly with an anterior chamber maintainer (Barron, Katena Product, Denville, NJ, U.S.A.) and using an 8 mm-sized trephine blade (Barron), the donor cornea was scored at 500 µm depth, and the anterior corneal lamella was removed carefully with a crescent knife (Alcon, Fort Worth, TX, U.S.A.). The remaining thin posterior lamella and endothelium were used to compensate for the corneal discrepancy. They were trephined from the inside with a 6 mm-sized blade (Mediprize, Seki, Japan), and the recipient rat cornea was also trephined carefully with a 4 mm-sized blade (Mediprize), grasping the globe firmly with the two fine tooth forceps until place at the immediate entrance into the anterior chamber. The graft was secured with 12 to 14 interrupted 10-0 nylon sutures and tarsorrhaphy was performed finally at one-third of the area from the lateral canthus to finally provide good coverage of the corneal surface. Prednisolone acetate 1% (Pred forte®, Allergan, Irvine, CA, U.S.A.) and levofloxacin (Cravit®, Allergan, Irvine, CA, U.S.A.) were applied twice a day; in addition, apraclonidine 0.5% (Iopidine®, Alcon), latanoprost 50 mg/mL (Xalatan, Pfizer, New York, NY, U.S.A.) and timolol maleate 0.25% (Timoptix XE®, Merck & Co., Inc. Whitehouse Station, NJ, U.S.A.) were instilled once a day after surgery. The IOP was obtained with Tonopen (Tonolab, Helsinki, Finland) until 13 days post-surgery to exclude the possibility of other causes of corneal edema. Grafted eyes were examined daily using an operating microscope (Carl Zeiss, Oberkochen, Germany) and a portable slit lamp (Welch & Allen, Jungingen, Germany) to evaluate edema and graft rejection. Total graft rejection was defined as fully developed graft edema or necrosis when the graft lost its transparency and totally obscured the contour of the iris behind it. To evaluate the pattern of cell infiltration, one rat from the control group were respectively sacrificed at 4, 7, 10, 13, and 16 days after transplantation, and the rest were sacrificed at the time of clinically evident rejection. The median survivals of the grafts in each group were calculated from the life table method, and the statistical differences were verified with log-rank test in 95% confidence interval (SPSS, Ver. 12.0). The corneas were sent for histological evaluation or RNA extraction. The infiltrating cells were stained with Hematoxylin & Eosin and were counted in five consecutive high power fields (×400) under light microscopy.
The corneas obtained from the control and Group 10 at the postoperative 4, 7, 10, 13, and 16 days were fixed in 10% neutral buffered formalin and incubated overnight at 4℃. They were cut to 4 µm thickness and dried at 60℃ for 1 hr and deparaffinized with ethanol. Protenase K (20 µg/mL, Sigma, St. Louis, MO, U.S.A.), 3% H2O2 and 0.3% triton X-100 were used for serially treatments and then 1% serum was finally added. Then they were incubated at room temperature with primary antibodies of CD4 (1:100 Chemicon, Billerica, MA, U.S.A.), CD8 (1: 100, Chemicon) and PBS (negative control). The anti-rabbit IgG (1:5,000, Southernbiotech, Birmingham, AB, U.S.A.), anti-streptavidn (1:5,000, Usbiological, Swampscott, MA, U.S.A.), and anti-mouse IgG (1:5,000, Sigma) were used as secondary antibodies and incubated for 30 min. The chromophore of Alexa 488 (1:500 Invitrogen, Carlsbad, CA, U.S.A.) and Hoechest 33342 (Sigma) were used for counter staining. At 400× magnification, under the confocal microscopy confocal (LSM 510 Meta, Zeiss, Yena, Germany), the number of immunofluorescent cells were counted five times in a single sample, and the results were then averaged.
The cornea, ipsilateral cervical lymph nodes, and lacrimal glands were removed from the rats in the control and group 10 at 4, 7, 10, 13, and 16 days after transplantation; each tissue was sliced with a scissor into small pieces and then homogenized by a sonificator (Fisher, Pittsburgh, PA, U.S.A.). The samples were again lysed in 350 µL extraction buffer (100 nM/L Tris HCL PH 8.0 and 10 mM/L lice) and were then centrifuged at 2,500 rpm to remove cellular debris for 20 min. The supernatants were transferred to a new tube and put into a deep freezer at -70℃. The detection and quantification of protein from the cytokines produced were as follows. Briefly, the harvested proteins were diluted at 0.1 µg for each well in coating buffer (0.05 M carbonate bicarbonate buffer) and incubated overnight at 4℃. Then they were washed in 0.15 M phosphate-buffered saline. The primary monoclonal mouse antibody of anti-rat IL-2 IL-4, IL-5, IL-10, and IFN-γ (R&D systems, Minneapolis, MN, U.S.A.) were added and incubated for 2 hr at 37℃. After washing three times with PBS-buffered saline, secondary antibodies of 100 µL HRP-goat anti-mouse IgG conjugate (1:5,000, Invitrogen, Carlsbad, CA) was added into each well. After 15 min later, H2SO4 (1 M, 100 µL) was also added finally, and the concentrations of each cytokine were measured using an enzyme-liked immunosorbent assay (ELISA) kit (Molecular devices, Sunnyvale, CA, U.S.A.) at 490 nm. Normal healthy tissues were used to compare baseline activity.
In comparison to the xenograft without any systemic immunosuppression, the influence of intramuscularly injected DEXA 1 mg/kg, CsA 1.25 mg/kg, and MMF 1.25 mg/kg on the production of cytokines was investigated on the 10th postoperative day, when most of the rejections were presumed to occur.
Survival analysis using the life-table method was performed to estimate the median time to graft failure in each group, and the log-rank test were used to assess the statistical significance of the differences in the median survival time between the groups. Data were expressed as the median survival time in the survival analysis and as mean±standard error in the IOP, cell count, and ELISA analysis.
In all rats, the mean IOP was well maintained until 10 days after surgery; then the IOP slowly went down and the stromal necrosis followed (Fig. 1). The grafts looked clear, and the anterior chamber was well reformed at 3 days after surgery. In most of the rejections, the corneal edema that obscured the iris structure was found at first; and then, severe necrosis involving the corneal stroma developed soon (Fig. 2). The median survival was 9.26 days for the control group (Group 1) in the orthotopic porcine-to-rat corneal xenograft, while it was 7.00 days (p=0.21) for Group 2 with dexamethasone 2 mg/kg, 7.25 days (p=0.11) for Group 3 with dexamethazone 2 mg/kg and cyclosporin A 10 mg/kg, 9.50 days (p=0.10) for Group 4 with dexamethasone 1 mg/kg, 10.25 days (p=0.91) for Group 5 with dexamethazone 1 mg/kg and cyclosporin A 5 mg/kg, 9.07 days (p=0.71) for Group 6 with dexamethazone 1 mg/kg and cyclosporin A 2.5 mg/kg, 8.77 days (p=0.64) for Group 7 with dexamethazone 1 mg/kg and cyclosporin A 1.25 mg/kg, 11.35 days (p=0.75) for Group 8 with dexamethazone 1 mg/kg, cyclosporin A 1.25 mg/kg and mycophenolate mofetil 6 mg/kg, 8.50 days (p=0.16) for Group 9 with dexamethazone 1 mg/kg, cyclosporin A 1.25 mg/kg, and mycophenolate mofetil 1.25 mg/kg, and 9.87 days (p=0.74) for Group 10 with dexamethazone 1 mg/kg, cyclosporin A 1.25 mg/kg, mycophenolate mofetil 1.25 mg/kg, and pretreatment with dextran 1 mg/mL, intracameral injection and cyclosporin A 2.5 mg/kg intraperitoneal injection for a week injection; these survivals were not significantly different from the control (Fig. 3).
In the early phase of post-surgery on day 4, the dominant infiltrating cells were polymorphonuclear cells (PMNs); however, from the 7th day, the cells began to infiltrate deep into the stroma and the mononuclear cells increased in number (Fig. 4, 5). At 13 days after surgery, some activated fibroblasts were seen in the stroma, but most of the layered architecture was almost completely destroyed. CD4+ lymphocytes and CD8+ lymphocytes were observed at 7 days, but they were few in number (Fig. 6). The immunohistochemical staining revealed that the number of CD4+ stained cells was always smaller than that of the CD8+ stained cells till the 16 days. Mean percentages of CD8+ expressed cells were higher (15%) than those of CD4+ stained cells (7%) throughout the study period (Fig. 7).
The expression of IL-2 and IFN-γ demonstrated a steady increase in all tissues while there was a rapid remarkable increase in the cornea at about 10 to 13 days after transplantation. The increase of IL-4 was found at 10 days in the lacrimal glands and cervical lymph nodes (Fig. 8). After suppression of cellular reactivity with the application of Dexa 1 mg/kg, CsA 1.25 mg/kg and MMF 1.5 mg/kg, the production of IL-2, IL-4 and IFN-γ was markedly reduced in the lacrimal glands and lymph nodes. However, there was no reduction of IL-2, IL-4, IL-10, and IFN-γ in the cornea, even with systemic immune suppression (Fig. 9).
We found cyclosporine and mycophenolate combined with dexamethasone did not prolong the median survival in orthotopic porcine-to-rat corneal xenotransplantation. Histology showed that the main infiltrative cells were monocytes and CD8+ lymphocytes during the rejection, and cytokine assay revealed the time gap between the rising time of IL-2 and IFN-γ (10-13 days) and the median rejection time (8 days), suggesting that CD4+ cell-mediated delayed type cellular rejection seems not to be mainly involved in rejection of this porcine-rat xeno-model.
In spite of an urgent need for alternatives to human organ donation for transplantation, the outcome of xenografts remains unclear. We investigated the immunological feasibility of using a porcine cornea as a xenograft for human transplantation. There is some data on porcine islet cells as well as kidney and heart cells transplanted into primate recipients (8-10). However, these outcomes cannot be directly applied to draw conclusions regarding porcine corneal transplantation because the cornea differs from other organs; it has no blood vessels, is regarded to be immune privileged, and is exposed directly to the environment with continuous exposure to pathogens. We started our investigations with a porcine-to-rat animal model as the first step for the evaluation of mechanisms of rejection; although primate experiments may have been preferred, which was not an option for us at this time. Among the animals that are widely used in the ophthalmic research, rats are easy to acquire the antibodies for an in vitro assay, compared to rabbits, and have larger corneas than mice, thus easier to operate on and observe the outcome after surgery.
The predominant role of humoral immunity has been extensively studied, and the recent advances with transgenic or cloned pigs, together with the scanty distribution of the xenonatural antibody a-gal (gal alpha 1-3gal beta 1-4Glc- NAc-R) epitope, as well as the immune privileged environment of the cornea, have made it possible to overcome hyperacute rejection regardless of the presence of immunoglobulin or B lymphocytes in the graft (1, 2, 11). Actually, the porcine-to-rat model is disconcordant in terms of pedigree. While considering both rats and pigs that have a-gals, the porcine-to-rat model is a concordant model in terms of a-gal aspect. Therefore, we did not expect a hyperacute rejection associated with a-gal in these models. However, there remains the possibility of acute or delayed graft rejection in association with innate immune cells or T lymphocytes associated with adaptive responses even if immunoglobulins do not directly influence the graft. There is some evidence that even the nonvascularized tissue, such as pancreatic islet cells or neuronal tissue, which are not susceptible to anti-a-gal antibodies directly, can be destroyed by different immune pathways (12, 13). Generally, the rejection associated with a corneal allograft is mediated primarily by CD4+ T cells; one report has suggested a critical role for CD4+ T cells in xeno-corneal rejection in a guinea pig-to-mouse xeno-model (4, 14). This finding led us to investigate the role of CD4+ T cells in a porcine-to-rat xeno-corneal transplantation model and to evaluate the positive effect of immunosuppressants on the survival.
Contrary to our expectation, cyclosporine and mycophenolate mofetil could not prolong the median survival, although they are potent immunosuppressive agents that can mainly suppress the activity of T and B cells (15). The late increase of IL-2 and IFN-γ suggested CD4+ cell-mediated cellular responses developed after 10-13 days, and it seemed to dis-match with the median rejection periods of the grafts. Effector CD8+cells also amounted to less than 15% throughout the rejection, but the number was higher than that of CD4+ cells. The time of emerging innate inflammatory cells such as neutrophils and monocytes before CD4+ cells render them activate corresponds with the time of rejection. Taken together, the rejection mechanism of the xeno-corneal transplantation in rats was more associated with effector CD8+ T cells and innate cellular reaction rather than CD4+ cell-orchestrated delayed type cellular rejection. These findings were consistent with previous studies that suggested the importance of non-CD4+ T cell mediated rejection in xenotransplanatation (16-18). For xenotransplantation conditions, the normal host immune cells might fail to respond adequately to a xenogeneic antigen; certain accessory adhesion molecules and cytokines are species-specific (19). Therefore, in contrast to the critical role of the CD4+ T cell in delayed rejection of allografts, non-T cell involvement might play a more important role in cellular rejection of a xenograft (20-22). Also, in a xenograft model of an athymic rat as recipient, the involvement of macrophages and NK-cell infiltrate have also been observed (7). The possible failure of the recipient NK cell's inhibitory receptor to recognize the species-specific donor MHC I might increase the susceptibility for non-CD4+ T cell-mediated graft rejection as well. However, whether xenograft rejection is primarily dependent on CD4+ T cells and NK depletion continues to be debated (16, 17). We believe that each type of immune cell may have a partial contribution to xenograft rejection, and their expression is likely to be time-dependent. This concept may be applied to the current model reported here in that the cells that represented innate immunity may have destroyed most of the graft at first before the activation of the adaptive immune system represented by T cells in the porcine-to-rat xeno-corneal transplantation. We are currently investigating the role of NK cells and macrophages in xeno-corneal transplantation.
Meanwhile, the low molecular weight dextran sulfate (MW 5000) is known to protect target cells from complement activation and NK cell-mediated injury (23). However, our study showed the addition of 1 mg/mL of low molecular weight dextran sulfate in Group 10 failed to prolong graft survival.
Considering that IFN-γ and IL-2 were found to markedly increase in the cornea, lacrimal glands and cervical lymph nodes after 10-13 days, Th1 cells appeared to be actively involved in the cellular reaction at that time. However, we could not confirm an association of Th2; this was because IL-4, IL- 5, and IL-10 increased very little or showed only equivocal changes compared with the control. The inefficient reduction of IL-2, IL-4, IL-10, and IFN-γ in the cornea, even with systemic immunosuppressants, suggested that the systemic immune suppressants could not reach the cornea in time because of its poor vascularity. More studies would be required about the reason why IFN-γ was rather increased in the cornea with immunosuppression, compared to that without immunosuppression.
Although our result was partially limited because T cells might not be fully blocked with our regimens, other findings including these support the importance of innate immune cells having a primary role in cellular rejection of the porcine graft before CD4+ T cell-mediated rejection occurred.
In conclusion, acute innate cellular reaction and effector CD8+ T cells appears to play a key role in the porcine-to-rat orthotopic corneal xenograft rejection before the involvement of delayed cellular responses demonstrated by CD4+ T cells. These findings provide the basis for better understanding of the immunology of xeno-corneal transplantation.
Figures and Tables
Fig. 1
Mean IOP of orthotopic rat corneal xenotransplantation. The measured IOP was well controlled until 10 day after surgery with some decline due to the tissue destruction as the rejection proceeded.

Fig. 2
Clinical features of porcine-to-rat orthotopic corneal transplantation. (A) Immediate after surgery, the graft looked clear. (B) At the time of rejection, the shadow of iris was lost and most rats showed severe stromal necrosis (13 days after surgery).
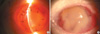
Fig. 3
(A) The survival curve of grafts without any systemic immunosuppression and with immunosuppression (life table method), (B) survival analysis of grafts based on the immunosuppressive regimen (life table method). The median survival was not significantly different among all the groups included in this study (Log-rank test).
Group 1, Control (n=21); Group 2, Dexamethasone 2 mg/kg (n=5); Group 3, Dexamethazone 2 mg/kg+cyclosporin A 10 mg/kg (n=5); Group 4, Dexamethasone 1 mg/kg (n=7); Group 5, Dexamethazone 1 mg/kg+cyclosporin A 5 mg/kg (n=7); Group 6, Dexamethazone 1 mg/kg+cyclosporin A 2.5 mg/kg (n=6); Group 7, Dexamethazone 1 mg/kg+cyclosporin A 1.25 mg/kg (n=7); Group 8, Dexamethazone 1 mg/kg+cyclosporin A 1.25 mg/kg+Mycophenolate mofetil 6 mg/kg (n=8); Group 9, Dexamethazone 1 mg/kg+cyclosporin A 1.25 mg/kg+ Mycophenolate mofetil 1.25 mg/kg (n=7); Group 10, Dexamethazone 1 mg/kg+cyclosporin A 1.25 mg/kg+Mycophenolate mofetil 1.25 mg/kg and pretreatment with dextran 1 mg/mL, intracameral injection and cyclosporin A 2.5 mg/kg intraperitoneal injection for a week injection (n=18)

Fig. 4
The inflammatory cells infiltration after porcine-to-rat xenotransplantation. At 4 days after graft, there were relatively few cells and polymorphonuclear cells (PMNs) were dominant (A, red arrows indicate PMNs; ×400). Many infiltrating cells were found, deep in the stroma at 7 days (B, red arrows indicate PMNs, white arrows indicate monocytes; ×400). However, most infiltrating cells were replaced by monocytes or lymphocytes at 10 days(C, ×400) and 13 days (D, ×200).
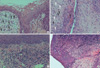
Fig. 5
The feature of inflammatory cells infiltrated after porcine-to-rat xenotransplantation. PMNs dominate until 10 days, and then monocytes are increasing after 7 days. The number of lymphocytes was not very high until the last examination.
*The percent denoted the expression of the individual cells against the whole inflammatory cells.
N, polymorphonuclear neutrophils; P, plasma cell; L, lymphocyte; M, monocyte; Ma, macrophage.

Fig. 6
The immunohistochemical staining of the grafts in the control (A, B) and immunosuppressed rats (C, D) at 10 days showed few reactive cells indicating CD4+ cells (A and C, ×400, white arrows indicate stained cells), and a relatively higher number of positively stained CD8 + cells (B and D, ×400, white arrows indicate stained cells).

Fig. 7
The ratio of specific T-cells against the whole infiltrating cells per high power field in the control (A) and immunosuppressed cornea B at 10 days. The surface reactivity for the CD4, CD8 cells were relatively low regardless of the time spent. There was no significant differences between the control and immunosuppressed groups.
*Percent (%) denotes the expression of positively stained cells for anti-CD4, CD8 antibody against the whole cells.
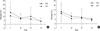
Fig. 8
Cytokine production in normal xenografts. The expression of IL-2 and IFN-γ increased steadily after surgery in all tissues, but the IL-4 showed a peak at 10 days in lacrimal glands and lymph nodes.
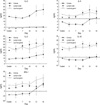
Fig. 9
Cytokine profile in the xenografts after immune suppression. After systemic suppression of cellular reactivity, the cytokines produced in lacrimal gland and lymph node showed generalized reduction. However, in the cornea the cytokines were not suppressed efficiently.
Control, normal cornea without transplantation; Non-suppression, transplanted cornea without systemic immunosuppression; Suppression, transplanted cornea with systemic immunosuppression containing dexamethazone 1 mg/kg+cyclosporin A 1.25 mg/kg+mycophenolate mofetil 1.25 mg/kg. and pretreatment with dextran 1 mg/mL, intracameral injection and cyclosporin A 2.5 mg/kg intraperitoneal injection for a week injection.
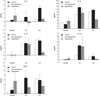
References
1. Amano S, Shimomura N, Kaji Y, Ishii K, Yamagami S, Araie M. Antigenicity of porcine cornea as xenograft. Curr Eye Res. 2003. 26:313–318.


2. Sano Y, Okamoto S, Streilein JW. Induction of donor-specific ACAID can prolong orthotopic corneal allograft survival in "high-risk" eyes. Curr Eye Res. 1997. 16:1171–1174.
3. Tanaka K, Yamada J, Joyce N, Streilein JW. Immunobiology of xenogeneic cornea grafts in mouse eyes. I. Fate of xenogeneic cornea tissue grafts implanted in anterior chamber of mouse eyes. Transplantation. 2000. 69:610–616.
4. Tanaka K, Yamada J, Streilein JW. Xenoreactive CD4+ T cells and acute rejection of orthotopic guinea pig corneas in mice. Invest Ophthalmol Vis Sci. 2000. 41:1827–1832.
5. Yamada J, Kurimoto I, Streilein JW. Role of CD4+ T cells in immunobiology of orthotopic corneal transplants in mice. Invest Ophthalmol Vis Sc. 1999. 40:2614–2621.
6. Sonoda KH, Nakao S, Nakamura T, Oshima T, Qiao H, Hisatomi T, Kinoshita S, Ishibashi T. Cellular events in the normal and inflamed cornea. Cornea. 2005. 24:Suppl 8. S50–S54.


7. Larkin DF, Takano T, Standfield SD, Williams KA. Experimental orthotopic corneal xenotransplantation in the rat. Mechanisms of graft rejection. Transplantation. 1995. 60:491–497.


8. Rood PP, Buhler LH, Bottino R, Trucco M, Cooper DK. Pig-to-non-human primate islet xenotransplantation: a review of current problems. Cell Transplant. 2006. 15:89–104.


9. Cabezuelo JB, Ramirez P, Chavez R, Majado M, Munitiz V, Munoz A, Hernandez Q, G-Palenciano C, Pino-Chavez G, Loba M, Yelamos J, Vizcaino AS, Cayuela M, Segura B, Marin F, Rubio A, Fuente T, Gago MR, Rios A, Montoya M, Esteban A, Bueno FS, Robles R, Cozzi E, White DJ, Parrilla P. Assessment of renal function during the postoperative period following liver xenotransplantation from transgenic pig to baboon. Transplant Proc. 2002. 34:321–322.


10. Candinas D, Belliveau S, Koyamada N, Miyatake T, Hechenleitner P, Mark W, Bach FH, Hancock WW. T cell independence of macrophage and natural killer cell infiltration, cytokine production, and endothelial activation during delayed xenograft rejection. Transplantation. 1996. 62:1920–1927.
11. Tanemura M, Galili U. T cells interacting with the alpha-Gal epitope: studies in alpha1, 3 Galactosyltransferase knock-out mice. Transplant Proc. 2000. 32:921–923.
12. McKenzie IF, Koulmanda M, Mandel TE, Sandrin MS. Pig islet xenografts are susceptible to "anti-pig" but not Gal alpha(1,3)Gal antibody plus complement in Gal o/o mice. J Immunol. 1998. 161:5116–5119.
13. Rijkelijkhuizen JK, Haanstra KG, Wubben J, Tons A, Roos A, van Gijlswijk-Janssen DJ, Ringers J, Bouwman E, Jonker M. T-cell-specific immunosuppression results in more than 53 days survival of porcine islets of langerhans in the monkey. Transplantation. 2003. 76:1359–1368.


14. Qian Y, Dana MR. Molecular mechanisms of immunity in corneal allotransplantation and xenotransplantation. Expert Rev Mol Med. 2001. 3:1–21.


15. Kobashigawa JA, Miller LW, Russell SD, Ewald GA, Zucker MJ, Goldberg LR, Eisen HJ, Salm K, Tolzman D, Gao J, Fitzsimmons W, First R. Study Investigators. Tacrolimus with mycophenolate mofetil (MMF) or sirolimus vs. cyclosporine with MMF in cardiac transplant patients: 1-year report. Am J Transplant. 2006. 6:1377–1386.


16. Simeonovic CJ, Ceredig R, Wilson JD. Effect of GK1.5 monoclonal antibody dosage on survival of pig proislet xenografts in CD4+ T cell-depleted mice. Transplantation. 1990. 49:849–856.


17. Karlsson-Parra A, Ridderstad A, Wallgren AC, Moller E, Ljunggren HG, Korsgren O. Xenograft rejection of porcine islet-like cell clusters in normal and natural killer cell-depleted mice. Transplantation. 1996. 61:1313–1320.
18. Nielsen B, Steinbruchel DA, Lillevang ST, Kemp E. Evidence for a primarily humoral rejection mechanism in concordant xenogeneic heart transplantation. A sequential immunohistological study in a hamster-to-rat model. APMIS. 1993. 101:587–594.


19. Brouard S, Gagne K, Blancho G, Soulillou JP. T cell response in xenorecognition and xenografts: a review. Hum Immunol. 1999. 60:455–468.


20. Goslings WR, Yamada J, Dana MR, Streilein JW, van Beelen E, Prodeus AP, Carroll MC, Jager MJ. Corneal transplantation in antibody-deficient hosts. Invest Ophthalmol Vis Sci. 1999. 40:250–253.
21. Andres A, Toso C, Morel P, Bosco D, Bucher P, Oberholzer J, Mathe Z, Mai G, Wekerle T, Berney T, Buhler LH. Macrophage depletion prolongs discordant but not concordant islet xenograft survival. Transplantation. 2005. 79:543–549.


22. Tanaka K, Sonoda K, Streilein JW. Acute rejection of orthotopic corneal xenografts in mice depends on CD4(+) T cells and self-antigen-presenting cells. Invest Ophthalmol Vis Sci. 2001. 42:2878–2884.
23. Laumonier T, Walpen AJ, Maurus CF, Mohacsi PJ, Matozan KM, Korchagina EY, Bovin NV, Vanhove B, Seebach JD, Rieben R. Dextran sulfate acts as an endothelial cell protectant and inhibits human complement and natural killer cell-mediated cytotoxicity against porcine cells. Transplantation. 2003. 76:838–843.

