Abstract
The aim of this study was to investigate the effect of erythropoietin (EPO) on histological brain injury, subventricular zone (SVZ) expansion, and sensorimotor function deficits induced by hypoxia-ischemia (HI) in newborn rat pups. Seven-day-old male rat pups were divided into six groups: normoxia control, normoxia EPO, hypoxia control, hypoxia EPO, HI control, and HI EPO group. Sham surgery or HI was performed in all animals. HI was induced by ligation of the right common carotid artery followed by 90 min of hypoxia with 8% oxygen. Recombinant human EPO 3 U/g or saline was administered intraperitoneally, immediately, at 24- and 48-hr after insult. At two weeks after insult, animals were challenged with cylinder-rearing test for evaluating forelimb asymmetry to determine sensorimotor function. All animals were then sacrificed for volumetric analysis of the cerebral hemispheres and the SVZ. The saline-treated HI rats showed marked asymmetry by preferential use of the non-impaired, ipsilateral paw in the cylinder-rearing test. Volumetric analysis of brains revealed significantly decreased preserved ipsilateral hemispheric volume and increased ipsilateral SVZ volume compared with the sham-operated animals. Treatment of EPO significantly improved forelimb asymmetry and preserved ipsilateral hemispheric volume along with decreased expansion of ipsilateral SVZ following HI compared to the saline-treated HI rats. These results support the use of EPO as a candidate drug for treatment of neonatal hypoxic-ischemic brain damage.
Perinatal hypoxic-ischemic encephalopathy (HIE) is common, and reported to affect two to four of every 1,000 full-term neonates, and considerably more preterm babies (1). Approximately 15 to 20% of term asphyxiated newborns with HIE die during the newborn period and 25% of the survivors exhibit permanent neuropsychological deficits such as mental retardation, cerebral palsy, seizures, and learning disabilities (1). Despite the fact that perinatal asphyxia closely corresponds to experimental models of cerebral hypoxia-ischemia (HI), where successful neuroprotective interventions have not been introduced, to date, no reliable treatment strategies have been developed to mitigate neurological injury and the resulting impairments in the clinical setting.
The protective effect of exogenously administered erythropoietin (EPO) has received a great deal of attention for various brain damage in adult animals, and promising data are emerging with perinatal animal models also. The neuroprotective effects exerted by EPO are mediated by various direct and indirect mechanisms (2-4). For evaluation of the efficacy of a pharmacological treatment of brain injury, it is very important to assess not only the histopathological evidence, but also the improvement of neurobehavioral performance. Recent studies have suggested that use of EPO has a neuroprotective effect in the neonatal HI rat model, based on neuropathological and biochemical data (5-7). However, there has been limited emphasis on the effectiveness of EPO on behavioral alterations after EPO treatment in a postnatal day (P) 7 HI rat model (8-10).
The subventricular zone (SVZ), found along the ependymal layer of the lateral ventricle, is known to generate neural progenitor cells throughout postnatal life (11), and neurogenesis at this cerebral region might be capable of replacing neurons after ischemic brain injury (12, 13). Recent experimental data indicate that diverse forms of ischemic injury stimulate neural precursor proliferation; however, most of these investigations have focused on neuronal production in the adult rodent brain (12, 13). A recent report from Plane et al. (14) and Chang et al. (15) showed that HI or transient middle cerebral artery occlusion in a neonatal rodent model resulted in augmentation of the SVZ size similar to results from the adult model, and that the expansion of the SVZ was directly proportional to the severity of the injury. Yang et al. (16) and Ong et al. (17) also recently reported that hypoxia-ischemia injuries resulted in expansion of the SVZ in neonatal rats.
However, there have been conflicting reports on the finding of the effects of EPO on the changes in the SVZ. Wang et al. (18) reported that EPO enhanced neurogenesis and expansion of the SVZ after stroke in the adult rodent; Chang et al. (15) reported that EPO markedly preserved hemispheric volume and decreased the expansion of SVZ in a neonatal rat stroke model. However, the effect of EPO on changes in the neonatal SVZ after HI in the newborn rat has not been previously studied.
Therefore, herein, the therapeutic potential effects of EPO in P7 HI rats were investigated by evaluating histopathology, using volumetric analysis of brain volume; in addition, the neurofunctional outcome was assessed by the examination of sensorimotor function using the cylinder-rearing test. Furthermore, we also evaluated whether EPO administration affects the expansion of the SVZ in response to a hypoxic- ischemic insult to the neonatal brain, by a volumetric analysis of SVZ of the rat brain.
The experimental protocols were reviewed and approved by the Institutional Animal Care and Use Committee (IACUC) of the Samsung Biomedical Research Institute (SBRI), Seoul, Korea. This study also followed the institutional and National Institutes of Health guidelines for laboratory animal care. Sprague-Dawley rats (Daihan Biolink Co., Seoul, Korea) with dated pregnancies were maintained at the same center and housed in individual cages with free access to water and laboratory chow. The offspring delivered spontaneously were reared with their dams. To exclude gender effects, male P7 rat pups weighing 12-15 g were used for the experiments (19).
The P7 male rat pups (n=43) were randomly divided into six groups: 1) normoxia control (n=5), normoxia plus saline; 2) normoxia EPO (n=5), normoxia plus EPO; 3) hypoxia control (n=5), hypoxia plus saline; 4) hypoxia EPO (n=5), hypoxia plus EPO; 5) HI control (n=11), HI plus saline; and 6) HI EPO (n=12), HI plus EPO. Cerebral hypoxia-ischemia was induced by a modification of the method reported originally by Rice et al. (20) as previously described (21, 22). Rat pups were anesthetized in a small jar containing cotton soaked with methoxyflurane, and deep anesthesia was maintained during the surgical procedure by placing a small plastic tube containing cotton soaked with methoxyflurane over the nose. The neck was incised in the midline, and the right common carotid artery was permanently ligated with 4-0 silk. The total time for surgery in each animal never exceeded three minutes. Originally described Rice-Vannuci rat hypoxic-ischemic model produces large infarcts (20) and depletes dorsal SVZ (23). Therefore, we reduced the severity of the insult by reducing the hypoxia exposure time to 90 min and found that it produced a moderate level of injury (21, 22). Following a two-hr recovery and feeding period, the animals were exposed to 90 min of hypoxia (8% O2 and 92% N2) by placing them in airtight containers partially submerged in a 37℃ water bath to maintain a constant thermal environment. Animals in the groups of normoxia control, normoxia EPO, hypoxia control, and hypoxia EPO received a sham-operation. The sham-operated pups underwent the same surgical procedure with exposure of the carotid artery without ligation. Pups received either 0.9% saline with the same volume as the EPO or recombinant human EPO (Epokine prefilled®: erythropoietin alpha, CJ Corp., Seoul, Korea) (a kind gift from CJ Corp.) at a dose of 3 units per gram of body weight injected intraperitoneally three times: upon retrieval from the hypoxia chambers or the sham operation, 24 and 48 hr after insult. After the injection, the pups were returned to their dams for 14 days until sacrifice.
It has been shown that episodes of unilateral cerebral HI in P7 rats result in neuronal loss in the cortex, basal ganglia, and hippocampus (24). As in humans, rats that experience cerebral HI have motor and cognitive deficits. In a cylinder-rearing test of paw placement while exploring a transparent cylinder, rats that underwent unilateral HI on P7 have been shown to exhibit an ipsilateral paw weight-bearing preference as early as on P21 (25). This cylinder-rearing test has been shown to reveal lateralizing sensorimotor deficits in neonatal HI (25).
Forelimb use bias was analyzed by the movements of each rat during exploratory activity in a transparent polymethyl-methacrylate (Atoglas, Atofina Chemicals Inc., Philadelphia, PA, U.S.A.) cylinder that measured 20 cm in diameter and 30 cm in height, at 14 days after surgery (P21). The size of the cylinder allowed free movements but was small enough to encourage rearing and wall exploration. Its height prevented the rat from reaching the top edge. The cylinder was sufficiently heavy that it did not move when the animal supported its weight against the wall. Animals were handled for about 10 min per day three days before testing. Each animal was individually placed in the cylinder and observed for three minutes. The initial forepaw placement of each weight-bearing contact with the wall during a full rear was recorded, or "both" were recorded for simultaneous contact with both forepaws (26). Results were expressed as the percentage of use of the non-impaired forelimb (right, ipsilateral) relative to the total number of forepaw movements at initiation. Investigators blinded to the treatment group were assigned to the scoring. All animals were recorded twice and the average scores were used for data analysis.
For the histopathological examination, after cylinder-rearing testing, animals were anesthetized with 10 mg/kg ketamine (Yuhan Corp., Gunpo, Korea), and euthanized at P21 by transcardiac perfusion with 0.1 M phosphate-buffered saline (PBS, pH 7.4). Their brains were rapidly removed, cryoprotected, embedded in optimal cutting temperature (OCT) compound (Tissue-Tek®, Sakura Finetek, Torrance, CA, U.S.A.), and quickly frozen. The 10 µm thick serial sections were made using a cryostat (Thermo Electron Corp., Waltham, MA, U.S.A.) and were mounted in the slide with intersection intervals of 80 µm. The mounted sections were air-dried, stained with hematoxylin-eosin, dehydrated in graded ethanol solutions, cleared in Citrosolv (Fischer Scientific, Pittsburg, PA, U.S.A.), and cover-slipped in Permount (Fisher Scientific).
All volumetric quantifications were performed with an Olympus BX 40 photomicroscope (Olympus Optical Co., Ltd., Tokyo, Japan) equipped with a high-resolution charge-coupled device (CCD) camera (Olympus Optical Co. Ltd.), a motorized XYZ axis computer-controlled stage, and the Stereoinvestigator software package (Version 6.52, Micro-Bright Field, Inc, Williston, VT, U.S.A.). When calculating the volume, the cross-sectional areas of the region of interest (ROI) in each section were traced on the computer screen at low power using a 1.5×/4× lens and then, the volume was calculated using the Stereoinvestigator software according to the Cavalieri's principle (27). The sections encompassed the whole striatum rostrally from the genu of the corpus callosum and caudally to the rostral part of the hippocampus corresponding to Plates 7-30 of the Structure of the rat brain (28). For the ROI, the right and left hemispheres and the SVZ were traced. Morphologic criteria were used consistently in all animals to determine the boundaries of the SVZ. The dorsolateral striatal extension of the SVZ, which resembles the dark band and thin long triangle in the sections corresponding to Plates 10-23 of Structure of the rat brain (28), was outlined. Briefly, the superior-medial boundary of SVZ was defined by the corpus callosum, the lateral boundary by the striatum, and the inferior boundary by the lateral ventricular margin.
Using this sampling strategy, approximately ten histological sections per brain were evaluated for hemispheric measurements, and approximately five sections for SVZ measurement per brain were analyzed. Size alterations of the SVZ were assessed by calculating the percent SVZ volume in the affected versus control hemisphere for each animal. For anatomical evaluation of cerebral injury, the ratio of the ipsilateral remaining cerebral hemisphere volume to the volume of corresponding contralateral cerebral hemisphere was expressed as a percentage. Quantification was conducted by an examiner blinded to the treatment group.
Data were presented as mean±SD. For statistical analysis, nonparametric methods were used. Significance was set at p<0.05. The Kruskal-Wallis test was applied for comparisons of multiple groups followed by the Wilcoxon rank sum test including the Bonferroni correction for comparison between two groups. Evaluation of the relationship between the percentage of preserved hemispheric volume, and expansion of the SVZ as well as the percentage of non-impaired paw initiation was performed by linear regression. All statistical analyses were done using SAS® Enterprise Guide, version of 3.0.2 (SAS Institute, Cary, NC, U.S.A.).
There were no significant differences in the whole brain volume of animals in the sham-operated groups, whether they were exposed to hypoxia, or administered with EPO, or not. The contralateral hemispheric volumes of the animals were not statistically different among the six experimental groups (data not shown). For anatomical assessment of cerebral injury, the ratio of the ipsilateral remaining cerebral hemispheric volume to the volume of corresponding contralateral cerebral hemisphere was expressed as a percentage (Fig. 1). For the saline treated animals after HI, there was a significant decrease in the percentage of preserved ipsilateral hemispheric volume compared with the sham-operated animals (p<0.05). Animals that received EPO following HI showed a significant reduction of ipsilateral hemispheric volume loss compared with the saline treated HI animals (p<0.05).
From the rostral portion of the hematoxylin and eosin-stained brain sections corresponding to Plate 10 of the Structure of the rat brain (28), the SVZ was observed as a densely stained band like structure between the corpus callosum and the subcallosal striatum (Fig. 2A). Moving caudally to the caudal sections corresponding to Plate 23 of the Structure of the rat brain (28), the SVZ widened dorsolaterally and showed a long triangular shape with a dorsolateral tail beneath the corpus callosum (Fig. 2D). In the sham-operated animals treated with saline versus those treated with EPO, or exposed with normoxia versus those exposed with hypoxia, there were no differences in the morphology of the SVZ. Whereas the contralateral SVZ did not show any changes in morphology, the ipsilateral SVZ in the saline-treated HI animals revealed marked morphological alterations in more rostral sections with significant expansion rostrally and laterally (Fig. 2B). In addition, in the more caudal sections, a more triangular shape was noted, caused by ipsilateral atrophy of striatum and corresponding widening of the lateral ventricle (Fig. 2E). EPO administration following HI significantly ameliorated these morphological changes of SVZ rostrally (Fig. 2C) and caudally (Fig. 2F). When we measured the SVZ volume on the contralateral side to operation, there were no statistical differences among the six experimental groups (data not shown). We calculated the percentage volume ratio of the SVZ ipsilateral over contralateral to the insult to measure the degree of volume changes in the ipsilateral SVZ (Fig. 3A). Saline-treated HI animals showed a significantly increased percentage volume of the ipsilateral SVZ compared to the sham-operated animals (p<0.05). EPO treatment significantly attenuated this ipsilateral SVZ expansion following HI (p<0.05). The relationship between the degree of SVZ enlargement and the degree of brain tissue injury was evaluated by comparison between the ratio of percentage of the hemispheric volume and the percentage SVZ volume, which showed an inverted linear relationship with coefficient (r2) of 0.2701 (p<0.05) (Fig. 3B). Therefore, the ipsilateral SVZ size was larger than its counterpart in the contralateral hemisphere, despite the fact that the ipsilateral hemisphere itself was smaller in size than the contralateral hemisphere.
The percentage of non-impaired forepaw initiation during weight-bearing episodes with the cylinder-rearing test was calculated in P21 rats (Fig. 4A) to evaluate the lateralizing sensorimotor deficit (25). Sham-operated animals showed symmetrical use of paws bilaterally when rearing in the cylinder, and the percentage of ipsilateral and contralateral paw initiation was the same at about 30%. HI animals treated with saline showed significant asymmetry due to the preferential use of the non-impaired, ipsilateral paw when rearing, compared with the sham-operated animals (p<0.01). EPO treatment significantly improved this unimpaired forelimb bias for wall movements compared with saline-treated HI animals (p<0.05) (Fig. 4A). When the percentage of hemispheric volume ipsilateral versus contralateral and the percentage of the non-impaired paw initiation with the cylinder test was compared, an inverted linear relationship with the coefficient (r2) of 0.2437 was observed (p<0.05) (Fig. 4B).
Our results demonstrate that multiple doses of EPO followed by HI decreased histopathological brain injury along with simultaneous expansion of the ipsilateral SVZ and improved lateralizing sensorimotor deficits in a neonatal rat model. This study describes for the first time the detailed morphological effect of EPO on alterations of the SVZ in developing rats subjected to unilateral cerebral HI using irreversible right common carotid artery ligation and hypoxemia.
Perinatal HI insults occur during a period in CNS development when there is extensive proliferation, migration, and cellular differentiation. The SVZ adjacent to the lateral ventricle is a forebrain region in mammalian animals where neurogenesis persists postnatally (29, 30). The SVZ contains a population of stem cells and more mature progenitors of neurons, astrocytes, and oligodendrocytes, and normally the SVZ neuroblasts migrate tangentially along the rostral migratory stream to reach the olfactory bulb where they mature into local interneurons (29, 31). However, after injury, the cells of the SVZ proliferate and some of these cells migrate toward the damaged areas in the adult animal models (12, 13, 32). Previous studies have provided evidence that a severe HI insult in the neonatal rat brain depleted the precursors within the first 48 hr of recovery, resulting in SVZ regression (23, 33). Recently, however, Plane et al. (14), Yang et al. (16), and Ong et al. (17) using a neonatal HI model, and Chang et al. (15) using a neonatal stroke model reported that insults caused the expansion of the SVZ. These contradictory findings in neonatal animals may be due to the difference in exposure duration of hypoxia, species-related differences in the susceptibility and response of HI, and different maturational stages among animals of the same species. Furthermore, there are conflicting reports regarding the effect of EPO in stroke induced SVZ expansion. In an adult stroke model, Wang et al. (18) found that treatment with EPO significantly improved functional recovery, along with increases in density of cerebral vessels, increased numbers of bromodeoxyuridine (BrdU)-positive cells in the ipsilateral SVZ, but did not decrease the brain infarct volume significantly. Contrary to this finding, Chang et al. (15) reported that EPO treatment preserved injured hemispheric volume and decreased the expansion of SVZ in a neonatal stroke model. These contradictory results might have resulted from the differences in infarct volume reduction by EPO treatment in these two studies. Here, we showed that a moderate HI brain injury during the neonatal period expands the SVZ, which is consistent with previous studies (13, 14, 16, 17), and that the expansion of the SVZ was directly correlated with the intensity of the injury similar to the results reported by Plane et al. (14). Furthermore, our results showed that EPO decreased the SVZ expansion induced by HI injury very similar to the findings reported by Chang et al. (15). Although we did not evaluate the proliferation or differentiation of precursor cells in the SVZ in this study, morphological SVZ expansion is directly related with the proliferation of precursor cells comprising the SVZ (12-14). It has been reported that stem or precursor cells in neurogenic areas of the adult brain respond to injury (34). Therefore, we cautiously assume that the ameliorated brain injury as a result of EPO administration might influence the reactive expansion of SVZ. However, caution is needed in interpreting the effect of EPO on neonatal SVZ in this study, because we investigated only the morphologic change of SVZ at one time point, not the serial investigation of proliferation of precursor cells in SVZ. Demonstrating functional improvement accompanied by histological improvement following brain injury strengthens the promise of the neuroprotective therapy. Several studies have reported that EPO administration improved neurobehavioral dysfunction caused by HI injury in newborn rats. Kumral et al. (8) demonstrated that EPO administered immediately after a HI insult in P7 rats produced long-lasting improvements in the cognitive function of the rats using the Morris water maze test at two weeks and again nineteen weeks after injury. Spandou et al. (9) demonstrated that EPO administration before HI insult significantly reduced the severity of brain damage and improved the short functional recovery at 24 hr after insult in neonatal rats. Spandou et al. (10) also demonstrated that a single dose of EPO administered immediately after HI insult, in the neonatal period, significantly reduced the severity of brain damage and prevented long-term sensorimotor deficits. The test was done at 42 days postnatally in animals using a battery of behavioral tests including Rota-Rod treadmill, grip traction test, foot fault test, postural reflex test, and limb placing test. In our study, the cylinder-rearing test was used to determine sensorimotor ability at fourteen days after HI insult (P21). Unilateral damage to the forelimb region of the rat brain sensorimotor cortex caused chronic deficits in sensorimotor function and use of the contralateral forelimb was easily quantified. In each of the models of unilateral injury, the rats relied on the non-impaired forelimb for exploratory movements along the walls of the cylinder rearing. The cylinder-rearing test has been used in established models of unilateral stroke, Parkinson's disease, and spinal cord injury (35). The test also proved useful for the evaluation of the neuroprotective effect of previous forced limb use after 6-hydroxydopamine administration (36). Measurement of asymmetry is well suited for evaluating functional consequences after unilateral brain injury. It is a simple, sensitive, and reliable tests that allows the observer to study behavior under unforced conditions. In order to obtain reproducible results, it is critical to reduce stressful stimuli. Gustavsson et al. (26) reported that evaluation of sensorimotor function in freely moving animals was achievable with the cylinder rearing test ant that this approach is advantageous compared with tests that require handling, such as the grip-traction test, foot-fault test, postural reflex test, and the limb-placing test. In the neonatal stroke model, the cylinder-rearing test has been proven to be useful for sensorimotor deficits in P24 rats (15). Grow et al. (25) reported that both normal and HI rats explore the walls of a cylinder as early as P21. In the present study, HI animals showed significant asymmetry due to the preference of use of the non-impaired, ipsilateral paw when rearing, compared to the sham-operated animals. EPO treatment significantly improved this asymmetry following HI and this functional improvement was positively correlated with the degree of tissue preservation in the injured hemisphere. This is the first study, to our knowledge, to demonstrate that EPO treatment improves sensorimotor function in P21 neonatal rats after HI insult. Thus, the improvement of functional deficits and the neuroprotection observed by histopathology demonstrated in this model may be helpful for the consideration of potential therapeutic strategies.
In summary, administration of multiple doses of EPO in P7 HI rats demonstrated a neuroprotective effect when given after the end of a hypoxic exposure by showing not only histopathological improvement evidenced by decreased brain injury and reduced reactive SVZ expansion, but also sensorimotor functional improvement. This neuroprotective effect of EPO suggests consideration of possible neonatal interventions in humans with perinatal hypoxic-ischemic encephalopathy.
Figures and Tables
Fig. 1
Stereologic volumetric quantification for histological brain injury at 2 week after insult (P21). Measuring volume of contralateral hemisphere in all animals did not show any significant differences among the 6 experimental groups (data not shown). Remaining tissue volume in the ipsilateral hemisphere was shown as a ratio of intact ipsilateral over the contralateral hemispheric volume. Cerebral hypoxia-ischemia significantly decreased percentage ipsilateral hemispheric volume of the P21 rats. EPO significantly ameliorated this ipsilateral hemispheric volume loss following hypoxic-ischemic insult. Data shown as mean±SD.
*p<0.05, compared with sham-operated animals (Sham); †p<0.05, compared with hypoxia-ischemia control.
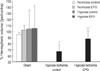
Fig. 2
Morphological changes of subventricular zone (SVZ) of the ipsilateral brain of rats at 2 weeks after insult (P21). Hematoxylin-eosin stained brain sections revealed SVZ as densely stained dark band (arrowheads) rostrally (A) and long triangular shape caudally (D) in sham-operated animals (Sham). Shapes of ipsilateral SVZ in sham operated animals (Sham) were similar, whether they were exposed with hypoxia or treated with EPO or not (data not shown). SVZ of ipsilateral brain of the rats subjected to HI expands significantly (black arrows) rostrally (B) and caudally (E), and shaped more triangular caudally (E). EPO treatment significantly decreased these expansions of SVZ (white arrows) rostrally (C) and caudally (F). Upper row represents sections including rostral part of striatal SVZ corresponding to Plate 11 of Structure of the rat brain. Lower row represents sections including mid-striatal SVZ corresponding to Plate 16 of Structure of the rat brain. Black stars indicate lateral ventricle. White star indicates widening of lateral ventricle due to striatal atrophy following hypoxic-ischemic insult. Each bar represents 0.5 mm.
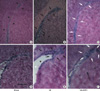
Fig. 3
Stereologic volumetric quantitification of subventricular zone (SVZ) at 2 weeks (P21) after insult in P7 rats (A). Eythropoietin (EPO) or hypoxia-exposure did not affect the ipsilateral SVZ volume in sham-operated animals (Sham), and there were no significant differences in contralateral SVZ volume in the animals among 6 groups (data not shown). Changes of ipsilateral SVZ volume was shown as a percentage of ipsilateral SVZ volume over the contralateral SVZ volume. Percentage SVZ volume was significantly increased in the saline treated hypoxic-ischemic rat brain compared to that of sham operated animals. EPO treatment significatly decreased this ipsilateral SVZ volume expansion. Data shown as mean±SD. *p<0.05 compared with sham-operated animals (Sham); †p<0.05 compared with hypoxia-ischemia control. Relationship between the percentage preserved ipsilateral hemispheric volume and ipsilateral percent expansion of SVZ volume was evaluated by regression analysis and showed a direct inverse relationship (r2=0.2701, p<0.05) (B).
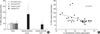
Fig. 4
Percentage of non-impaired (ipsilateral) forepaw initiation at weight-bearing when P21 rats (at 2 weeks after insult) underwent cylinder-rearing test in 6 different groups (A). Saline-treated hypoxia-ischemia control animals showed a significantly asymmetric preferential use of non-impaired forelimb compared with sham operated animals. EPO treatment significantly ameliorated this sensorimotor fucntional deficit following HI insult. Data shown as mean±SD. *p<0.05 compared with sham-operated animals (Sham); †p<0.05 compared with hypoxia-ischemia control. Forelimb use asymmetry was directly correlated with the degree of histological brain injury shown by regression analysis of percentage of non-impaired limb initiation in the cylinder-rearing test and percentage preserved hemispheric volume (r2=0.2437, p<0.05) (B).
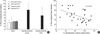
References
2. Agnello D, Bigini P, Villa P, Mennini T, Cerami A, Brines ML, Ghezzi P. Erythropoietin exerts an anti-inflammatory effect on the CNS in a model of experimental autoimmune encephalomyelitis. Brain Res. 2002. 952:128–134.


3. Digicaylioglu M, Lipton SA. Erythropoietin-mediated neuroprotection involves cross-talk between Jak2 and NF-kappaB signalling cascades. Nature. 2001. 412:641–647.
4. Shingo T, Sorokan ST, Shimazaki T, Weiss S. Erythropoietin regulates the in vitro and in vivo production of neuronal progenitors by mammalian forebrain neural stem cells. J Neurosci. 2001. 21:9733–9743.
5. Aydin A, Genc K, Akhisaroglu M, Yorukoglu K, Gokmen N, Gonullu E. Erythropoietin exerts neuroprotective effect in neonatal rat model of hypoxic-ischemic brain injury. Brain Dev. 2003. 25:494–498.


6. Kumral A, Ozer E, Yilmaz O, Akhisaroglu M, Gokmen N, Duman N, Ulukus C, Genc S, Ozkan H. Neuroprotective effect of erythropoietin on hypoxic-ischemic brain injury in neonatal rats. Biol Neonate. 2003. 83:224–228.


7. Sun Y, Zhou C, Polk P, Nanda A, Zhang JH. Mechanisms of erythropoietin-induced brain protection in neonatal hypoxia-ischemia rat model. J Cereb Blood Flow Metab. 2004. 24:259–270.


8. Kumral A, Uysal N, Tugyan K, Sonmez A, Yilmaz O, Gokmen N, Kiray M, Genc S, Duman N, Koroglu TF, Ozkan H, Genc K. Erythropoietin improves long-term spatial memory deficits and brain injury following neonatal hypoxia-ischemia in rats. Behav Brain Res. 2004. 153:77–86.


9. Spandou E, Soubasi V, Papoutsopoulou S, Karkavelas G, Simeonidou C, Kaiki-Astara A, Guiba-Tziampiri O. Erythropoietin prevents hypoxia/ischemia-induced DNA fragmentation in an experimental model of perinatal asphyxia. Neurosci Lett. 2004. 366:24–28.


10. Spandou E, Papadopoulou Z, Soubasi V, Karkavelas G, Simeonidou C, Pazaiti A, Guiba-Tziampiri O. Erythropoietin prevents long-term sensorimotor deficits and brain injury following neonatal hypoxia-ischemia in rats. Brain Res. 2005. 1045:22–30.


11. Lois C, Garcia-Verdugo JM, Alvarez-Buylla A. Chain migration of neuronal precursors. Science. 1996. 271:978–981.


12. Arvidsson A, Collin T, Kirik D, Kokaia Z, Lindvall O. Neuronal replacement from endogenous precursors in the adult brain after stroke. Nat Med. 2002. 8:963–970.


13. Parent JM, Vexler ZS, Gong C, Derugin N, Ferriero DM. Rat forebrain neurogenesis and striatal neuron replacement after focal stroke. Ann Neurol. 2002. 52:802–813.


14. Plane JM, Liu R, Wang TW, Silverstein FS, Parent JM. Neonatal hypoxic-ischemic injury increases forebrain subventricular zone neurogenesis in the mouse. Neurobiol Dis. 2004. 16:585–595.


15. Chang YS, Mu D, Wendland M, Sheldon RA, Vexler ZS, McQuillen PS, Ferriero DM. Erythropoietin improves functional and histological outcome in neonatal stroke. Pediatr Res. 2005. 58:106–111.


16. Yang Z, Levison SW. Hypoxia/ischemia expands the regenerative capacity of progenitors in the perinatal subventricular zone. Neuroscience. 2006. 139:555–564.


17. Ong J, Plane JM, Parent JM, Silverstein FS. Hypoxic-ischemic injury stimulates subventricular zone proliferation and neurogenesis in the neonatal rat. Pediatr Res. 2005. 58:600–606.


18. Wang L, Zhang Z, Wang Y, Zhang R, Chopp M. Treatment of stroke with erythropoietin enhances neurogenesis and angiogenesis and improves neurological function in rats. Stroke. 2004. 35:1732–1737.


19. Zhu C, Xu F, Wang X, Shibata M, Uchiyama Y, Blomgren K, Hagberg H. Different apoptotic mechanisms are activated in male and female brains after neonatal hypoxia-ischaemia. J Neurochem. 2006. 96:1016–1027.


20. Rice JE 3rd, Vannucci RC, Brierley JB. The influence of immaturity on hypoxic-ischemic brain damage in the rat. Ann Neurol. 1981. 9:131–141.


21. Park WS, Sung DK, Kang S, Koo SH, Kim YJ, Lee JH, Chang YS, Lee M. Neuroprotective effect of cycloheximide on hypoxic-ischemic brain injury in neonatal rats. J Korean Med Sci. 2006. 21:337–341.


22. Park WS, Sung DK, Kang S, Koo SH, Kim YJ, Lee JH, Chang YS, Lee M. Therapeutic window for cycloheximide treatment after hypoxic-ischemic brain injury in neonatal rats. J Korean Med Sci. 2006. 21:490–494.


23. Levison SW, Rothstein RP, Romanko MJ, Snyder MJ, Meyers RL, Vannucci SJ. Hypoxia/ischemia depletes the rat perinatal subventricular zone of oligodendrocyte progenitors and neural stem cells. Dev Neurosci. 2001. 23:234–247.


24. Vannucci RC, Vannucci SJ. A model of perinatal hypoxic-ischemic brain damage. Ann N Y Acad Sci. 1997. 835:234–249.


25. Grow JL, Liu YQ, Barks JD. Can lateralizing sensorimotor deficits be identified after neonatal cerebral hypoxia-ischemia in rats? Dev Neurosci. 2003. 25:394–402.


26. Gustavsson M, Anderson MF, Mallard C, Hagberg H. Hypoxic preconditioning confers long-term reduction of brain injury and improvement of neurological ability in immature rats. Pediatr Res. 2005. 57:305–309.


27. Mandarim-de-Lacerda CA. Stereological tools in biomedical research. An Acad Bras Cienc. 2003. 75:469–486.


28. Swanson L. Brain maps III: structure of the rat brain: an atlas with printed and electronic templates for data, models, and schematics. 2004. 3 ed. San Diego, CA, USA: Elsivier Academic Press.
29. Lois C, Alvarez-Buylla A. Proliferating subventricular zone cells in the adult mammalian forebrain can differentiate into neurons and glia. Proc Natl Acad Sci USA. 1993. 90:2074–2077.


30. Goldman JE. Lineage, migration, and fate determination of postnatal subventricular zone cells in the mammalian CNS. J Neurooncol. 1995. 24:61–64.


31. Luskin MB. Restricted proliferation and migration of postnatally generated neurons derived from the forebrain subventricular zone. Neuron. 1993. 11:173–189.


32. Zhang RL, Zhang ZG, Zhang L, Chopp M. Proliferation and differentiation of progenitor cells in the cortex and the subventricular zone in the adult rat after focal cerebral ischemia. Neuroscience. 2001. 105:33–41.


33. Skoff RP, Bessert DA, Barks JD, Song D, Cerghet M, Silverstein FS. Hypoxic-ischemic injury results in acute disruption of myelin gene expression and death of oligodendroglial precursors in neonatal mice. Int J Dev Neurosci. 2001. 19:197–208.


34. Lie DC, Song H, Colamarino SA, Ming GL, Gage FH. Neurogenesis in the adult brain: new strategies for central nervous system diseases. Annu Rev Pharmacol Toxicol. 2004. 44:399–421.