Abstract
For the purpose of determining the pathogenic role of transforming growth factor-β1 (TGF-β1) in the mechanism of chronic rheumatic heart disease, we evaluated the expression of TGF-β1, proliferation of myofibroblasts, and changes in extracellular matrix components including collagen and proteoglycan in 30 rheumatic mitral valves and in 15 control valves. High TGF-β1 expression was identified in 21 cases (70%) of rheumatic mitral valves, whereas only 3 cases (20%) of the control group showed high TGF-β1 expression (p<0.001). Additionally, increased proliferation of myofibroblasts was observed in the rheumatic valves. High TGF-β1 expression positively correlated with the proliferation of myofibroblasts (p=0.004), valvular fibrosis (p<0.001), inflammatory cell infiltration (p=0.004), neovascularization (p=0.007), and calcification (p<0.001) in the valvular leaflets. The ratio of proteoglycan to collagen deposition inversely correlated with TGF-β1 expression in mitral valves (p=0.040). In conclusion, an ongoing inflammatory process, the expression of TGF-β1, and proliferation of myofibroblasts within the valves have a potential role in the valvular fibrosis, calcification, and changes in the extracellular matrix that lead to the scarring sequelae of rheumatic heart disease.
Valvular involvement in heart disease results in stenosis, insufficiency, or both, and the most common cause of mitral stenosis is chronic rheumatic heart disease (1, 2). Rheumatic fever (RF) is the consequence of a pharyngeal infection with group A streptococcus in a susceptible host, leading to autoimmune disease induced by antigen mimicry of human cardiac myosin with the streptococcal glycoprotein (3). While most disease manifestations are transient and leave no residua, rheumatic carditis can lead to chronic rheumatic heart disease (CRHD), which is characterized by fibrotic valvular deformity and can produce permanent and severe cardiac dysfunction even decades later (1).
The current hypothesis of CRHD is that fibrotic valvular deformity evolves through organization of acute inflammation induced by RF, with subsequent thickening and retraction of valvular leaflets, and with secondary damage resulting from turbulent flow induced by valvular dysfunction (1, 4). There are some evidences that CRHD is associated with ongoing inflammation of valvular leaflets. The plasma concentrations of TNF-α and high sensitive C-reactive protein (CRP) are significantly higher in CRHD than in controls (5-7). It has been shown that lymphocytes obtained from CRHD patients exhibit T helper 2 (Th2) type cytokine response whereas those from RF patients show T helper 1 (Th1) cytokine profile in cell culture with streptococcal superantigen (8). The Th2 immune response is known to promote fibrotic process by activating fibroblast proliferation, myofibroblast differentiation, extracellular matrix deposition, and transforming growth factor-beta 1 (TGF-β1) production, whereas Th1 type cytokines such as interferon-γ suppress these processes (9-11).
TGF-β1 plays a critical role in matrix remodeling and in enhancing collagen synthesis (12). Upon tissue injury, inflammation occurs and many cytokines are secreted and TGF-β1 is released, promotes myofibroblast differentiation, and stimulates fibroblasts and other reparative cells to proliferate and to synthesize extracellular matrix components. Under normal conditions this leads to provisional repair; however, with repeated injury, the increase in TGF-β1 production is sustained, leading to tissue fibrosis (13, 14). Recent studies have investigated TGF-β1 expression in diseased heart valves (15-17). Serotonin, which is associated with carcinoid heart disease (18), has been demonstrated to up-regulate TGF-β1 (15). Other reports indicate that TGF-β1 is present within calcific aortic stenosis cusps and that it mediates the calcification of aortic valve interstitial cells in cell culture via apoptosis (16). Additionally, in CRHD, the possibility that TGF-β1 gene polymorphism might play a role in determining the susceptibility to CRHD was suggested (17).
In this study, we investigated the expression of TGF-β1 and changes in extracellular matrix components in mitral valves affected by CRHD in order to determine the role of TGF-β1 in the delayed valvular fibrosis and deformity caused by CRHD.
Rheumatic mitral valves were obtained from pathology slides of 30 patients with CRHD who underwent valve removal and prosthetic valve replacement surgery carried out from 2002 to 2003 in Severance Hospital, Yonsei University College of Medicine, Seoul, Korea. These patients showed typical echocardiographic and gross findings of CRHD. Cases complicated with infectious endocarditis or collagen vascular disease were excluded. The age of the patients ranged from 25 to 74 yr (mean, 49.7 yr old), with 13 males and 17 females. The number of patients having mitral stenosis was 15, those with mitral regurgitation were 5, and those with both mitral stenosis and regurgitation were 10. Twenty-three patients demonstrated multiple valve involvement.
Normal heart valves were obtained from autopsies (14 cases) and from the removed heart of a transplantation recipient (1 case), with the diagnosis of dilated cardiomyopathy with Marfan syndrome but without definite valvular abnormality. The age of the patients ranged from 12 to 73 yr (mean, 39.9 yr), with 12 males and 3 females. The hearts obtained at autopsy were devoid of any abnormal findings except mild thickening of valvular leaflets in three cases. The causes of death were not heart-related. Specimens were fixed in 10% buffered formalin and embedded in paraffin. Serial sections were stained with hematoxylin and eosin for general morphology.
Pathologic examination of sections was carried out blinded to the clinical diagnosis. The status of valvular architecture was evaluated in comparison to the well established histology offered by the normal valves. The extent of fibrosis, the degree of inflammatory infiltrates and neovascularization, and the presence of patchy necrosis, calcification, and thrombus attachment in valvular leaflets were examined. The extent of fibrosis was semiquantitatively graded as follows: no change (-); mild fibrosis without architectural distortion: (1+); moderate fibrosis with architectural distortion: (2+); severe fibrosis obliterating the valvular architecture (3+). The inflammatory infiltrates was graded as follows: no inflammation: (-); scanty amount of inflammatory cell infiltration in the stroma: (1+); and moderate inflammatory cell infiltration: (2+). The cases showing severe inflammation in the stroma and endocardial surface of the valvular leaflets were excluded in this study. The degree of neovascularization was graded as: no change: (-); presence of several thin-walled vessels: (1+); presence of a few muscularized vessels: (2+); and presence of aggregates of muscularized and ectatic thin-walled vessels: (3+). Myxoid degeneration of valvular leaflets was scored as follows: <5%: (-), 6-25%: (1+), 25-50%: (2+), and >50% of areas showing myxoid degeneration in any low-power field: (3+).
Histochemical evaluation was performed for connective tissue elements with Movat pentachrome stain, which differentially stains collagen, elastin, and proteoglycans, to evaluate the architectural distortion, alcian blue (pH 2.0) to stain for proteoglycans, and Sirius red to stain for collagen, according to previously described methods. The slides stained with Movat pentachrome and alcian blue were examined under light microscope, and the sections stained with Sirius red were observed under polarizing microscope. Analysis of the extent of alcian blue and Sirius red staining was performed with a personal computer-based quantitative 24-bit (16.2 million unique combinations) color image analysis system (KS400, Ver. 2.0, Zeiss, Germany). The slides were scanned, negative background (black) was chosen for the threshold and the positive area was calculated by subtraction. The same threshold was applied to all specimens. The percentage of the total area with positive color for each section was recorded.
Paraffin-embedded tissues were sectioned in 4-µm slices. Sections were preincubated with 3% hydrogen peroxide, and antibodies against TGF-β1 (Serotec, Oxford, U.K.), vimentin (M7020, Dako Cytomation, Glostrup, Denmark), alpha-smooth muscle actin (SMA) (Dako Cytomation), CD3 (Novocastra, Newcastle, U.K.), CD20 (BD Pharmingen, San Diego, CA, U.S.A.), and CD68 (Dako Cytomation) were applied and incubated for 60 min at room temperature. Sections were incubated with biotinylated secondary antibody (Cap-plus kit, Zymed, San Francisco, CA, U.S.A.) for 30 min and then incubated with horseradish peroxidase-labelled streptavidin solution (Cap-plus kit, Zymed) for 30 min. Slides were rinsed in phosphate-buffered saline (pH 7.4) after each incubation step. Peroxidase activity was revealed by diaminobenzidine. Slides were counterstained with hematoxylin and mounted. Positive reaction for TGF-β1 was semi-quantitatively evaluated according to the extent of positive stromal area in any lowpower field (X40) as follows: <5%: negative, 5 to 25%: low, and >25%: high expression. The proliferation of myofibroblasts was graded according to the extent of SMA-positive myofibroblasts among valvular interstitial cells as: <5%: negative, 5 to 25%: low, and >25%: high degree.
Statistical analysis was performed using the PC-SPSS program (Ver 11.0, IL, U.S.A.). The data were calculated as mean±standard deviation when availabel. Fisher's exact test was used to analyze the data obtained from immunohistochemical findings, and the non-parametric Mann-Whitney U test was employed to evaluate the association of TGF-β1 expression with the deposition of proteoglycan and collagen. A p value less than 0.05 was considered to be statistically significant.
Histologic findings of the rheumatic mitral valves and control valves were summarized in Table 1. All control valvular leaflets showed architectural preservation of three layers including spongiosa, fibrosa, and ventricularis (Fig. 1A). Mild fibrosis was seen in three of them, mild to moderate myxoid degeneration in thirteen, and scanty lymphocytic infiltrates in two. Several CD68-positive histiocytes were present in the stroma of valve leaflets. Neovascularization, calcification, thrombus attachment, and necrosis of the leaflets were not observed.
By contrast, in all rheumatic mitral valves, valvular architectures were markedly distorted due to moderate-to-severe fibrosis (Fig. 2A). Neovascularization was seen in the central portion of the valvular leaflet in 26 cases. Six cases showed thin-walled small vascular structures and others contained thick muscularized vessels. Inflammatory cells infiltrated into the perivascular stroma in 15 cases (Fig. 2B) and were predominantly composed of CD3+ T lymphocytes. Thrombi were attached on the valvular leaflets in 11 cases, calcification was noted in 18 cases, and focal necrosis was found in the fibrotic stroma in 11 cases. Myxoid degeneration of valvular stroma was seen in 18 cases.
In those cases showing extensive stromal fibrosis in the vavluar leaflets, more pronounced neovascularization (p<0.001), increased inflammatory infiltrates (p=0.033), calcification (p<0.001), stromal necrosis (p=0.004), and thrombus attachment (p=0.009) were observed. Myxoid degeneration was seen in areas where fibrosis or calcification was not intense.
In CRHD, 21 cases of rheumatic valves (70%) showed high TGF-β1 expression, whereas only three cases of control valves (20%) demonstrated high TGF-β1 expression (p<0.001) (Table 2). Control valves exhibited TGF-β1 positivity on the subendothelial stroma of valvular leaflets (Fig. 1B). On the other hand, in CRHD, TGF-β1 was expressed in the endothelial cells and smooth muscle cells of the blood vessels (13 cases), the interstitial cells and perivascular stroma (3 cases), or both (14 cases) (Fig. 2C). High TGF-β1 expression correlated with severe valvular fibrosis (p<0.001), inflammatory cell infiltration (p=0.004), neovascularization (p=0.007) and calcification (p<0.001), but no correlation was observed between TGF-β1 expression and myxoid degeneration (p=0.173).
The number of SMA-positive myofibroblasts was significantly increased in valvular leaflets of CRHD compared to control valves (Table 3), and the proliferation of SMA-positive myofibroblasts positively correlated with TGF-β1 expression (p=0.004) (Table 4) and fibrosis of valvular leaflets (p<0.001). SMA-positive myofibroblasts were usually seen in the areas showing increased collagen deposition, and in the subendocardial stroma (Fig. 2D).
Extracellular matrix deposition of proteoglycan and collagen was markedly different in the CRHD and control groups. In control valves, the proteoglycan was present in the spongiosa and collagen was found in the fibrosa. Whereas, in CRHD, proteoglycan was deposited in the perivascular stroma or in areas showing myxoid degeneration, and the collagen was in the stroma showing dense fibrosis or myofibroblastic proliferation. The proportion of alcian blue-positive areas quantitatively calculated by image analyzer occupied a mean of 3.75% (range 0-23.5%) of valvular matrix in CRHD, compared to the mean of 13.2% (range 0-41.9%) in the control group (p=0.014) (Fig. 3A). The proportion of Sirius redpositive areas, which represent type I and III collagen deposition, averaged 23% (range 8.1-51.4%) in CRHD and 11.2% (range 5.9-22.0%) in the control group (p<0.001) (Fig. 3B). Additionally, the ratio of proteoglycan to collagen deposition was lower in the rheumatic mitral valve group than in the control valve group (p=0.001). The proportion of collagen and proteoglycan deposition was not associated with TGF-β1 expression (p=0.34 and 0.087), but the ratio of proteoglycan to collagen deposition was lower in the TGF-β1 positive group than in the negative group (p=0.04) (Fig. 3C).
CRHD, the most significant complication of RF, demonstrates severe fibrosis and distortion of valvular leaflets (1, 3). The factors leading to continued fibrosis and subsequent valvular disease remain incompletely defined. There are considerable evidences to indicate that TGF-β1 overproduction plays some role in fibrosis and dysfunction in heart valves. TGF-β1 expression is increased in carcinoid valve cusps (15), calcific aortic valve cusps (16), and prolapsed valves in a mouse model of Marfan syndrome (19). In cases of CRHD, TGF-β1 polymorphisms may predispose to valvular disease. Chou et al. demonstrated that patients with CRHD have a lower frequency of the TGF-β1 C509T CC genotype and a higher frequency of the T869C T allele (17). They proposed the possibility that TGF-β1 gene polymorphisms might play a role in determining susceptibility to CRHD. However, there has been no study showing localized overproduction of TGF-β1 in valvular leaflets of CRHD. Our study has demonstrated high TGF-β1 expression in valvular leaflets of CRHD and might support the hypothesis that TGF-β1 overproduction would play a role in the valvular fibrosis of CRHD.
It may be difficult to appreciate that, in valvular leaflets of CRHD, fibrosis and remodeling is going on even several decades after initial attack. There are some studies demonstrating persistent inflammation in valvular leaflets of CRHD (4, 20, 21). Lymphocytic infiltrations have been identified in valves removed 10-20 yr after initial attacks of RF, and the predominant cellular infiltrate was T lymphocytes (4). Prolonged persistence of group A streptococcal carbohydrate antibody was demonstrated in CRHD even decades later (20). Additionally, the plasma concentrations of TNF-α and highly sensitive CRP are significantly higher in CRHD (5, 6, 7, 21). According to the study by Bhatnagar et al. (8), lymphocytes derived from CRHD patients exhibit the Th2 type cytokine response whereas those from RF patients show the Th1 cytokine profile with response to the streptococcal superantigen. The Th2 immune response is associated with fibrotic process including TGF-β1 secretion, whereas Th1 type cytokines suppresses diverse fibrotic activities (9-11). Guilherme et al. (22) demonstrated that CD4+ T cells derived from heart lesions predominantly secrete Th1 type cytokines in both RF and CRHD patients. The different results observed in these two studies are probably due to the different characterization of CRHD patients analysed. The study by Guilherme et al. included the early stage of CRHD patients with age range from 10 to 20 yr, whereas the age of the patients in the study by Bhatnagar et al. ranged from 22 to 54 yr (8, 22). Therefore, the cytokine profiles of early stage CRHD might be similar to that of RF. It is possible that Th2 type cytokine response seen in the advanced CRHD (8) might be induce the TGF-β1 overexpression and the fibrotic process as well as prolonged inflammation seen in the distorted rheumatic valves. Our report confirmed the fact that ongoing inflammation mainly composed of T lymphocytes was present in the apparently resting valves of advanced CRHD, and TGF-β1 expression correlated with the degree of inflammatory infiltrates. Additionally, TGF-β1 expression was present predominantly in the perivascular stroma where inflammatory cells mainly infiltrated. These results support the hypothesis that a continued inflammatory process and overproduction of TGF-β1 within the valves of the susceptible hosts would lead to the prolonged fibrosis of valvular leaflets in advanced CRHD.
TGF-β1 induces the differentiation of cardiac fibroblasts to myofibroblasts (24), which can produce up to twice as much collagen as their fibroblast precursors (25). The emergence of these cells has been closely correlated with valvular fibrosis and degenerative lesions (26). Our study demonstrated that increased proliferation of myofibroblasts was observed in the rheumatic valves without alteration of cellularity of valvular interstitial cells and that high TGF-β1 expression was related to the proliferation of myofibroblasts and calcification of valvular leaflets. Calcific aortic stenosis cusps demonstrate higher concentrations of TGF-β1 within the extracellular matrix compared with noncalcified cusps (16). When cultured interstitial cells are exposed to TGF-β1 for a long period of time, aggregates of cells evolve into nodules that become apoptotic, express alkaline phosphatase, and then calcify (16). Therefore, increased TGF-β1 expression in rheumatic heart valves may induce the differentiation of valvular interstitial cells to the myofibroblasts and the overproduction of extracellular matrix, resulting in progressive fibrosis and valvular distortion, and rolonged exposure to TGF-β1 probably induces calcification of valvular leaflets seen in CRHD.
TGF-β1 is also known to play a critical role in matrix remodeling by enhancing collagen synthesis (12). The normal heart valve is composed of collagen, proteoglycan and elastin, and approximately 85% of the total collagen in the heart is type I collagen (27). In rheumatic valves, the total amounts of collagen, proteoglycan, and elastin are significantly increased (27). Our result demonstrated that the proportion of collagen deposition was increased, and proteoglycan deposition was decreased in rheumatic valvular leaflets compared to control valves, but that it did not correlate with TGF-β1 overexpression. Because only the percentage of collagen and proteoglycan deposited areas in the valvular leaflets was evaluated and the total amount of collagen was not calculated, we were unable to investigate the accurate relationship of collagen deposition to TGF-β1 expression. However, the ratio of proteoglycan to collagen deposited areas was lower in the TGF-β1 positive group than in the negative group. Therefore TGF-β1 expression might be related to the conversion of extracellular matrix from proteoglycan to collagen rather than to the percentage of collagen or that of proteoglycan.
There are a number of efforts that have aimed to reduce fibrosis in cardiovascular disease, such as post-ischemic fibrosis, and dilated and hypertrophic cardiomyopathies, and targeting the TGF-β1 may be effective in attenuating fibrosis and hypertrophy in the heart (26). Our study indicated that TGF-β1 may play some role in valvular fibrosis and deformity in CRHD, and therefore, TGF-β1 might be an appropriate target for prevention of subsequent valvular dysfunction in patients having suffered from RF.
In conclusion, TGF-β1 expression is increased in rheumatic mitral valves, and higher TGF-β1 expression is associated with valvular fibrosis, inflammatory cell infiltration, differentiation of valvular fibroblasts to myofibroblasts, calcification, and changes in the extracellular matrix in the valves of CRHD. These results support the hypothesis that TGF-β1 expression might play some role in the prolonged fibrosis and severe valvular deformity typical of CRHD.
Figures and Tables
Fig. 1
Histologic and immunohistochemical findings of control valves. (A) Valvular leaflets obtained from the control group showed well-preserved leaflet architecture without fibrosis or inflammatory cell infiltration (H&E, ×40). (B) Immunohistochemical staining for TGF-β1 demonstrated positivity in the subendothelial stroma of valvular leaflets (TGF-β1, ×40).
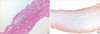
Fig. 2
Histologic and immunohistochemical findings of the rheumatic mitral valves. (A) Rheumatic mitral valves showed severe fibrosis and distorted architecture (H&E, ×40). (B) A high-power view demonstrated small thin-walled vessels and perivascular lymphocytic infiltration (H&E, ×200). (C) High TGF-β1 expression was seen in the endothelial cells and smooth muscle cells of the vessels, in the perivascular interstitial cells, and stroma of the valves (×200). (D) Myofibroblasts that were positive for SMA immunostaining were present in the subendothelial densely fibrotic area (×40).
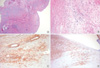
Fig. 3
Proteoglycan and collagen deposition in rheumatic mitral valves and control valves. (A) The proportion of proteoglycan deposited areas was lower in rheumatic mitral valve group (RM) than in the control group (black bars represented mean values: 3.75% vs. 13.2%) (p=0.014). (B) The rheumatic mitral valve group (RM) showed extensive collagen deposition compared to the control group (black bars represented mean values: 23% vs. 11.2%) (p<0.001). (C) The ratio of proteoglycan to collagen deposited areas was lower in the TGF-β1 positive group than in the TGF-β1 negative group (black bars represented mean values: 0.38 vs. 0.66) (p=0.040).
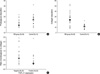
ACKNOWLEDGEMENTS
We thank Kyung Moo Yang, Pathologist in National Institute of Scientific Investigation for his contribution of collection of control valves, and members of the Department of Pathology, Inha University Hospital and Yonsei University College of Medicine for their supports. We express our sorrow over the death of Dr. Dong Hwan Shin, one of the coauthors of this article.
References
1. Schoen FJ. Cotran RS, Kumar V, Collins T, editors. The heart. Robbins pathologic basis of disease. 1999. 6th ed. Philadelphia: W.B. Saunders;543–599.


2. Rosai J. Rosai J, editor. Cardiovascular system. Ackerman's surgical pathology. 1996. 8th ed. St. Louis: Mosby;2173–2226.
3. Veasy LG, Hill HR. Immunologic and clinical correlations in rheumatic fever and rheumatic heart disease. Pediatr Infect Dis J. 1997. 16:400–407.


4. Kemeny E, Grieve T, Marcus R, Sareli P, Zabriskie JB. Identification of mononuclear cells and T cell subsets in rheumatic valvulitis. Clin Immunol Immunopathol. 1989. 52:225–237.


5. Chen MC, Chang HW, Wu CJ, Yang CH, Yu TH, Chen CJ, Hung WC. Balance between plasma levels of tumor necrosis factor-alpha and interleukin-10 in rheumatic mitral stenosis. Cardiology. 2005. 104:171–175.
6. Chiu-Braga YY, Hayashi SY, Schafranski M, Messias-Reason IJ. Further evidence of inflammation in chronic rheumatic valve disease (CRVD): high levels of advanced oxidation protein products (AOPP) and high sensitive C-reactive protein (hs-CRP). Int J Cardiol. 2006. 109:275–276.


7. Davutoglu V, Celik A, Aksoy M. Contribution of selected serum inflammatory mediators to the progression of chronic rheumatic valve disease, subsequent valve calcification and NYHA functional class. J Heart Valve Dis. 2005. 14:251–256.
8. Bhatnagar A, grover A, ganguly NK. Superantigen-induced T cell responses in acute rheumatic fever and chronic rheumatic heart disease patients. Clin Exp Immunol. 1999. 116:100–106.


9. Lakos G, Melichian D, Wu M, Varga J. Increased bleomycin-induced skin fibrosis in mice lacking the Th1-specific transcription factor T-bet. Pathobiology. 2006. 73:224–237.


10. Kimura T, Ishii Y, Yoh K, Morishima Y, Iizuka T, Kiwamoto T, Matsuno Y, Homma S, Nomura A, Sakamoto T, Takahashi S, Sekizawa K. Overexpression of the transcription factor GATA-3 enhances the development of pulmonary fibrosis. Am J Pathol. 2006. 169:96–104.


11. Wen FQ, Liu X, Kobayashi T, Abe S, Fang Q, Kohyama T, Ertl R, Terasaki Y, Manouilova L, Rennard SI. Interferon-gamma inhibits transforming growth factor-beta production in human airway epithelial cells by targeting Smads. Am J Respir Cell Mol Biol. 2004. 30:816–822.
12. Border WA, Noble NA. Transforming growth factor beta in tissue fibrosis. N Eng J Med. 1994. 331:1286–1292.
13. O'Kane S, Ferguson MW. Transforming growth factor betas and wound healing. Int J Biochem Cell Biol. 1997. 29:63–78.
14. Mutsaers SE, Bishop JE, McGrouther G, Laurent GJ. Mechanisms of tissue repair: from wound healing to fibrosis. Int J Biochem Cell Biol. 1997. 29:5–17.


15. Jian B, Connolly J, Savani RC, Narula N, Liang B, Levy R. Serotonin mechanisms in heart valve disease I: Serotonin-induced up-regulation of transforming growth factor-beta1 via G-protein signal transduction in aortic valve interstitial cells. Am J Pathol. 2002. 161:2111–2121.
16. Jian B, Narula N, Li QY, Mohler ER 3rd, Levy RJ. Progression of aortic valve stenosis: TGF-β1 is present in calcified aortic valve cusps and promotes aortic valve interstitial cell calcification via apoptosis. Ann Thorac Surg. 2003. 75:457–465.


17. Chou HT, Chen CH, Tsai CH, Tsai FJ. Association between transforming growth factor-beta1 gene C-509T and T869C polymorphisms and rheumatic heart disease. Am Heart J. 2004. 148:181–186.
18. Robiolio PA, Rigolin VH, Wilson JS, Harrison JK, Sanders LL, Bashore TM, Feldman JM. Carcinoid heart disease: Correlation of high serotonin levels with valvular abnormalities detected by cardiac catheterization and echocardiography. Circulation. 1995. 92:790–795.
19. Ng CM, Cheng A, Myers LA, Martinez-Murillo F, Jie C, Bedja D, Gabrielson KL, Hausladen JM, Mecham RP, Judge DP, Dietz HC. TGFbeta-dependent pathogenesis of mitral valve prolapse in a mouse model of Marfan syndrome. J Clin Invest. 2004. 114:1586–1592.
20. Ayoub EM, Toranta A, Bartley TD. Effect of valvular surgery on antibody to the group A streptococcal carbohydrate. Circulation. 1974. 50:144–150.


21. Golbasi Z, Ucar O, Keles T, Sahin A, Cagli K, Camsari A, Diker E, Aydogdu S. Increased levels of high sensitive C-reactive protein in patients with chronic rheumatic valve disease: evidence of ongoing inflammation. Eur J Heart Fail. 2002. 4:593–595.
22. Guilherme L, Cury P, Demarchi LM, Coelho V, Abel L, Lopez AP, Oshiro SE, Aliotti S, Cunha-Neto E, Pomerantzeff PM, Tanaka AC, Kalil J. Rheumatic heart disease: proinflammatory cytokines play a role in the progression and maintenance of valvular lesions. Am J Pathol. 2004. 165:1583–1591.
23. Gorelik L, Flavell RA. Transforming growth factor-beta in T-cell biology. Nat Rev Immunol. 2002. 2:46–53.
24. Walker GA, Masters KS, Shah DN, Anseth KS, Leinwand LA. Valvular myofibroblast activation by transforming growth factor-beta: implications for pathological extracellular matrix remodeling in heart valve disease. Circ Res. 2004. 95:253–260.
25. Lijnen P, Petrov V. Transforming growth factor-beta 1-induced collagen production in cultures of cardiac fibroblasts is the result of the appearance of myofibroblasts. Methods Find Exp Clin Pharmacol. 2002. 24:333–344.


26. Khan R, Sheppard R. Fibrosis in heart disease: understanding the role of transforming growth factor-β1 in cardiomyopathy, valvular disease and arrhythmia. Immunology. 2006. 118:10–24.