Abstract
Recent studies indicate that several Toll-like receptors (TLRs) are implicated in recognizing viral structures and instigating immune responses against viral infections. The aim of this study is to examine the expression of TLRs and proinflammatory cytokines in viral skin diseases such as verruca vulgaris (VV) and molluscum contagiosum (MC). Reverse transcription-polymerase chain reaction and immunostaining of skin samples were performed to determine the expression of specific antiviral and proinflammatory cytokines as well as 5 TLRs (TLR2, 3, 4, 7, and 9). In normal human skin, TLR2, 4, and 7 mRNA was constitutively expressed, whereas little TLR3 and 9 mRNA was detected. Compared to normal skin (NS), TLR3 and 9 mRNA was clearly expressed in VV and MC specimens. Likewise, immunohistochemistry indicated that keratinocytes in NS constitutively expressed TLR2, 4, and 7; however, TLR3 was rarely detected and TLR9 was only weakly expressed, whereas 5 TLRs were all strongly expressed on the epidermal keratinocytes of VV and MC lesions. In addition, the mRNA expression of IFN-β and TNF-α was upregulated in the VV and MC samples. Immunohistochemistry indicated that IFN-β and TNF-α were predominately localized in the granular layer in the VV lesions and adjacent to the MC bodies. Our results indicated that VV and MC skin lesions expressed TLR3 and 9 in addition to IFN-β and TNF-α. These viral-induced proinflammatory cytokines may play a pivotal role in cutaneous innate immune responses.
Toll-like receptors (TLRs) are mammalian homologues of Toll, which was originally identified in Drosophila (1). These receptors are expressed on both immune as well as non-immune cells and respond to specific components of microbial pathogens. TLR signaling results in the activation of nuclear factor κB (NF-κB), which triggers the production of a variety of antimicrobial and proinflammatory cytokines and chemokines (1, 2).
At least ten different human TLRs have been identified and have variable expression in different cell types as well as differential responses to a wide range of pathogens (3-5). For instance, TLR2 mediates responses to gram-positive bacteria-derived peptidoglycan, lipoprotein, and zymosan, whereas TLR4 mediates cellular responses to lipopolysaccharide derived from gram-negative bacteria (4, 5). Likewise, TLR2 and 4 can be activated by certain viral envelope proteins (6), TLR3 by double-stranded viral RNA (7), TLR7 by imidazoquinlones (8), and TLR9 by unmethylated CpG DNA derived from bacterial and viral genomes (9).
TLRs are expressed not only in peripheral blood mononuclear cells such as monocytes and macrophages (2, 3, 10), but also in various tissue cells such as fibroblasts (11) and endothelial cells (12). Our laboratory and other groups have reported that human keratinocytes express TLR2 and 4 (13, 14). Previous immunohistochemical studies indicated that TLR2 appears to be primarily expressed in the granular layer of the epidermis, while TLR4 is expressed principally in the basal epidermal region (15, 16). Epidermal keratinocytes in normal skin (NS) also constitutively expressed TLR1 and 5, while TLR3 was barely detectable in most cases (16). However, no studies have, to date, been carried out on the cutaneous expression patterns of TLRs in viral skin diseases.
In this study, we compared the expression of TLR2, 3, 4, 7, and 9 in the epidermis of normal human skin to that in verruca vulgaris (VV) and molluscum contagiosum (MC) skin lesions. The modulation of cutaneous proinflammatory cytokines was also examined in NS, VV, and MC skin lesions.
Ten patients with VV, including 8 patients with VV and 2 patients with condyloma acuminatum, and 8 patients with MC were enrolled in this study. Three-millimeter punch biopsies were taken from the skin lesions of each patient. In one half portion of the biopsy specimen the epidermis was separated from the dermis using a scalpel and was snap-frozen in liquid nitrogen for reverse transcription polymerase chain reaction (RT-PCR) studies. The other half of the specimen was embedded in optimal cutting temperature (OCT) compound (Sakura, Tokyo, Japan) for immunostaining. Five normal human skin samples were obtained from the adjacent NS tissue of patients who underwent benign cutaneous tumor surgery. All the skin samples were stored at -70℃ until further use. Informed consent was obtained from all the patients prior to collection of the samples.
Each skin sample was homogenized in a polytron (Kinematica AG, Luzernerstrasse, Littau-Lucerne, Switzerland). The total mRNA was isolated using TRIZOL® reagent (Sigma, Saint Louis, MO, U.S.A.) according to the manufacturer's instructions (17). cDNA was synthesized by performing reverse transcription using 3 μg of total mRNA from skin samples with Avian Myeloblastosis Virus Reverse Transcriptase (Promega Corporation, Madison, WI, U.S.A.) and random primers at 42℃ for 90 min. RT-PCR was performed as described in the manufacturer's protocol. Briefly, initial denaturation was performed at 94℃ for 5 min, followed by 35 amplification cycles of denaturing at 94℃ for 30 sec, annealing for 30 sec (the temperatures are listed in Table 1), extending at 72℃ for 40 sec, and finally incubating at 72℃ for 10 min. Specific oligonucleotide primers used to amplify TLRs, tumor necrosis factor-α (TNF-α), and interferon-β(IFN-β) were based on the published sequences (Table 1). The PCR amplified products were analyzed by 1.5% agarose gel electrophoresis and visualized by ethidium bromide staining with ultraviolet illumination. The quantifications of the PCR products were normalized by comparison with the amplified β-actin PCR product. The comparative mRNA levels of different gene products in the viral infected skin samples were designated as fold increases compared to the normal human skin.
Skin specimens were prepared for immunohistochemical staining as previously described (18). Briefly, five-micron cryostat sections were cut on a cryostat microtome (Leica Microsystems AG, Wetzlar, Germany) and then fixed in acetone for 15 min. After three washes in phosphate buffered saline (PBS), the sections were incubated in methanol with 0.3% hydrogen peroxide for 10 min to block the endogenous peroxidase activity. Nonspecific antibody binding was blocked by incubating the sections with 10% normal donkey serum (Jackson ImmunoResearch Laboratories, West Grove, PA, U.S.A.) for 60 min. The sections were incubated with rabbit antihuman polyclonal antibodies of TLR2 and 4, IFN-β, and TNF-α (1:100 in phosphate-buffered saline [PBS], Santa Cruz Biotechnology, Santa Cruz, CA, U.S.A.) or with goat anti-human polyclonal antibodies of TLR3, 7, and 9 (1:100 in PBS, Santa Cruz Biotechnology) overnight at 4℃. Subsequently, tissue sections were incubated with secondary antibodies (1:300 in PBS, Jackson ImmunoResearch Laboratories) for 60 min. As controls, the same skin specimens were incubated with an isotype-matched antibody using identical experimental conditions. As a negative control, skin samples were incubated with PBS without primary antibodies. The color reaction of treated tissue was carried out using the substrate 3-amino-9-ethylcarbazole (Vector Laboratories, Burlingame, CA, U.S.A.) for 10 min. The skin sections were also counterstained with hematoxylin (Vector Laboratories) for 1 to 2 min. Three observers independently reviewed the stained cells under a light microscope. The staining intensity was graded as 0 for negative, + for weakly positive, ++ for moderately positive, and +++ for strongly positive.
Skin sections were prepared for immunofluorescence staining as previously described (19). Briefly, five-micrometer cryostat sections were incubated in methanol with 0.3% hydrogen peroxide, followed by blocking with 5% normal donkey serum (Jackson ImmunoResearch Laboratories). They were incubated overnight at 4℃ with goat anti-human TLR3 polyclonal antibodies (1:50 in PBS, Santa Cruz Biotechnology). Then, tissue sections were incubated with Alexa Fluor 488-conjugated secondary antibodies (1:800 in PBS, Molecular probes, Eugene, OR, U.S.A.) for 60 min. After three washes in PBS, the tissue sections were blocked with 2.5% normal horse serum (Vector Laboratories) and were then incubated overnight at 4℃ with rabbit anti-human IFN-β polyclonal antibodies (1:50 in PBS, Santa Cruz Biotechnology). Subsequently, the tissue sections were incubated with biotin-conjugated secondary antibodies (1:200 in PBS, Jackson ImmunoResearch Laboratories) for 60 min and also with Streptavidin Alexa Fluor 546 conjugate (1:1,000 in PBS, Molecular probes) for 60 min. The immunolocalization of TLR3 and IFN-β in the skin was determined by Laser Scanning Confocal microscopy. The excitations of Alexa Fluor 488 and Alexa Fluor 546 were measured at laser wavelengths of 488 nm and 546 nm, respectively.
We examined the expression of TLR2, 3, 4, 7, and 9 mRNA in samples of normal human skin and in the VV and MC specimens by RT-PCR (Fig. 1). TLR2, 4, and 7 mRNA was constitutively expressed in normal (non-infected) human skin. Little expression of TLR3 and 9 mRNA was detected in these skin samples. Compared to NS, TLR3 and 9 mRNA was markedly upregulated in the VV and MC skin specimens when compared to β-actin mRNA expression for each experimental condition (Fig. 1). In contrast, there were little quantitative differences in the mRNA levels of TLR2, 4, and 7 between the NS and the viral-infected skin samples.
We next examined the epidermal localization of TLR2, 3, 4, 7, and 9 expression by immunohistochemical staining of normal human skin and VV and MC skin specimens (Fig. 2). Our results indicated that TLR2 was diffusely expressed throughout the whole epidermis except the horny layer in the NS. In MC skin samples TLR2 was most strongly expressed in the epidermal cells adjacent to the molluscum bodies in the suprabasal layer of the epidermis, whereas TLR2 expression was primarily localized in the granular and horny epidermal layer in the VV skin specimens. In contrast to TLR2,TLR3 was barely detectable in the epidermis of NS; however, it was highly expressed in epidermal cells adjacent to the molluscum bodies and also in the epidermal granular and horny layer of the epidermis that express viral inclusion bodies in the MC and VV skin samples. TLR4 was also expressed in the epidermis of NS predominantly beneath the granular layer and was more pronounced in epidermal cells adjacent to the molluscum bodies in the MC specimens and localized to the granular and horny layer in the VV specimens. In NS samples TLR7 was found to be expressed in the epidermal spinous and basal layer, but principally localized in the granular layer of the epidermis in cells containing viral inclusion bodies. Finally, TLR9 was weakly expressed in the basal and lower spinous layer of the epidermis in NS but strongly expressed in the epidermal cells expressing viral particles.
In addition to TLR, we also measured the expression of IFN-β and TNF-α in normal human skin and in VV and MC specimens by RT-PCR and immunohistochemical staining. These particular cytokines were selected for these studies because they are believed to play an important role in host responses to viral infections. Our results indicated that IFN-β and TNF-α mRNA was upregulated in both the VV and MC skin lesions compared to expression in NS (Fig. 3). Immunohistochemical studies indicated that both IFN-β and TNF-α were highly expressed in epidermal cells adjacent to the molluscum bodies in the MC skin specimens and strongly expressed in the granular layer in the VV skin samples (Fig. 4A, B).
Next, the co-expression of IFN-β and TLR3 in normal, VV, and MC skin samples was examined by double-labeled immunofluorescence staining in three VV and MC infected skin specimens. It was demonstrated that IFN-β and TLR3 were principally co-expressed in the epidermal cells adjacent to the molluscum bodies in the MC skin specimens as well as in the epidermal granular and horny layers with viral inclusion bodies in the VV skin samples. IFN-β expression was principally localized to the TLR3-positive epidermal cells. Therefore, these results indicated that the expression of IFN-β was localized to the TLR3 expressing epidermal cells (Fig. 4C).
Our results in this study indicated that normal human skin constitutively expresses TLR2, 4, and 7 mRNA but little TLR3 and 9 mRNA. Likewise, the immunohistochemical staining of NS revealed that TLR2, 4, and 7 were diffusely expressed throughout the epidermis; however, TLR9 was weakly expressed in the basal and lower spinous layers and TLR3 was barely detected. Kawai et al. (13) previously reported that both TLR2 and 4 were highly expressed in the basal layer of normal human skin. In contrast, Pivarcsi et al. (20) reported that TLR2 was principally expressed in the granular epidermal layer, whereas TLR4 was expressed in the basal layer of the epidermis. Baker et al. (16) reported that TLR2 was strongly expressed in the whole epidermis of normal human skin, but the expression of TLR3 and 4 was not detected. Kollisch et al. (21) failed to detect TLR4, 7, and 9 mRNA expression in neonatal foreskin. Most recently, Lebre et al. (22) demonstrated that normal keratinocytes constitutively express TLR 2, 3, and 4 mRNA, but not TLR7. This discrepancy with other studies might be due to differences in the methodological techniques or reagents used in this study (16). In addition, these results might be due to the difference in the biopsy site and skin specimens, or to the staining methods. On the other hand, we demonstrated TLR7 expression in normal keratinocytes, which has never been reported in previous studies. However, it is possible that there might be only a nonspecific activation of normal keratinocytes by undefined TLR7 ligands, endogenous or exogenous, which cannot be explained by TLR7-mediated specific effects such as the TLR7-imiquimod interaction.
VV and MC are caused by the double stranded DNA human papilloma virus and MC virus, respectively (23, 24). The precise mechanism of the human immune response to these viral skin diseases remains unclear. Cutaneous viral infections have been proposed to induce localized inflammatory and antiviral responses that are believed to result in the resolution of viral skin lesions (25, 26). Keratinocytes are one of the first cutaneous cell types to encounter various microbes including the papilloma and molluscum viruses. Keratinocyte TLRs are believed to play an important role in pathogen recognition and host innate immune responses in the skin. In recent studies, TLR3, 7, and 9 were shown to recognize different kinds of viruses (27, 28). In addition, imiquimod, a TLR7 agonist, has been shown to be an effective drug for the treatment of papilloma- induced warts (29). To our knowledge, however, the expression patterns of the TLRs in common viral skin diseases such as VV and MC are still unknown.
In this study, TLR3 mRNA was not detected in normal human skin samples, but clearly expressed in VV and MC skin specimens. Likewise, although low levels of TLR9 mRNA were noted in normal human skin, this was also augmented in VV and MC skin samples. Immunohistochemical staining likewise demonstrated that TLR2, 4, and 7 were expressed on the keratinocytes in the epidermis. Keratinocytes adjacent to epidermal cells containing viral inclusion bodies also expressed TLR3 and 9. These results support the potential role of these keratinocyte TLRs in the recognition and response to cutaneous papilloma and molluscum virus infections. Furthermore, it was notable that although TLR3 expression was barely detected in the normal human skin, expression was clearly augmented in the hyperkeratotic epidermis of the VV specimens and in the vicinity of the molluscum bodies in the MC skin lesions.
TLR3-activated cells have been reported to induce NF-κB activation, which results in the production of various proinflammatory cytokines including TNF-α , interleukin-1 (IL-1), and IL-8 (30). A second TLR3-activated signaling pathway activates the type I-IFN promoter that induces the release of antiviral cytokines such as IFNα/β (31-33). To examine the antiviral immune response through cutaneous TLR activation, we assessed IFN-β and TNF-α in normal and viral infected skin samples. Our results indicated that IFN-β and TNF-α mRNA were upregulated in the viral infected skin lesions, as compared to normal skin. Furthermore, immunohistochemical staining indicated that the expression of IFN-β and TNF-α was increased in the epidermal cells adjacent to the molluscum bodies and also in the granular layer of the VV specimens that contained many viral particles. The results of double-labeled immunofluorescent analysis also indicated that the expression of these proinflammatory cytokines was co-localized in the epidermis in the same regions as TLR3 in VV and MC skin lesions.
Although activation of TLR9 as well as TLR3 can induce production of type I IFNs (34), a recent report revealed that double-stranded RNA viral reactivity in keratinocytes is important for subsequent IFN-γ-mediated immune reactions (35), and that vaccinia virus (dsDNA virus) belonging to the Poxviridae family-like molluscum virus inhibited multiple TLR pathways, including TLR3 (36). Thus, we used a TLR3 antibody rather than a TLR9 antibody along with IFN-β and TNF-α for double immunofluorescence staining in this study.
In summary, our studies indicated that epidermal TLR3 and 9 may have an important role in cutaneous innate immune responses to commonly encountered cutaneous viral infections such as VV and MC. Understanding the relationship between viral skin infections and epidermal TLR responses may permit novel insights into cutaneous immune responses and the development of new anti-viral therapeutic agents.
Figures and Tables
Fig. 1
Expression of Toll-like receptor (TLR) mRNA in normal skin (NS), verruca vulgaris (VV), and molluscum contagiosum (MC) skin lesions. RT-PCR products were amplified from NS, VV, and MC using specific DNA oligo primers for the human TLR2, 3, 4, 7, and 9. The mRNA expression was normalized with β-actin mRNA expression for each experimental condition (A). The level of mRNA expression for each of these gene products in NS, VV, and MC was presented by fold increases over that of NS (B). Each bar represents mean±S.E.M.
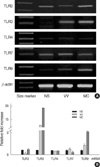
Fig. 2
Immunolocalization of Toll-like receptors (TLRs) in normal skin (NS), verruca vulgaris (VV), and molluscum contagiosum (MC) skin lesions. TLRs were immunolocalized in NS, VV, and MC skin samples using specific anti-human TLR2, 3, 4, 7, and 9 polyclonal antibodies. Original magnification, ×100.
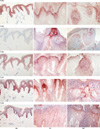
Fig. 3
IFN-β and TNF-α mRNA expression in normal skin (NS), verruca vulgaris (VV), and molluscum contagiosum (MC) skin lesions. RT-PCR products were amplified from normal skin (NS), VV, and MC skin samples using primers specific DNA oligo primers for IFN-β and TNF-α. The mRNA expression was normalized with β-actin mRNA for each experimental condition (A). The level of mRNA expression of VV and MC was presented by fold increases over that of NS (B). Each bar represents mean±S.E.M.
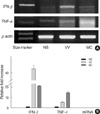
Fig. 4
Immunolocalization of IFN-β and TNF-α in normal skin (NS), verruca vulgaris (VV), and molluscum contagiosum (MC) skin lesions. IFN-β (A) and TNF-α (B) were immunolocalized in NS, VV, and MC skin samples using specific anti-human IFN-β and TNF-α polyclonal antibodies. IFN-β (red) and TLR3 (green) co-localization studies in human NS, VV, and MC skin samples were examined in C. Original magnification, ×100.
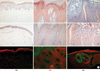
References
1. Rock FL, Hardiman G, Timans JC, Kastelein RA, Bazan JF. A family of human receptors structurally related to Drosophila toll. Proc Natl Acad Sci USA. 1998. 95:588–593.


2. Aderem A, Ulevitch RJ. Toll-like receptors in the induction of the innate immune response. Nature. 2000. 406:782–787.


3. Ulevitch RJ, Mathison JC, da Silva Correia J. Innate immune responses during infection. Vaccine. 2004. 22:Suppl 1. S25–S30.


5. Yoshimura A, Lien E, Ingalls RR, Tuomanen E, Dziarski R, Golenbock D. Cutting edge: recognition of Gram-positive bacterial cell wall components by the innate immune system occurs via Toll-like receptor 2. J Immunol. 1999. 163:1–5.
7. Matsumoto M, Kikkawa S, Kohase M, Miyake K, Seya T. Establishment of a monoclonal antibody against human toll-like receptor 3 that blocks double-stranded RNA-mediated signaling. Biochem Biophys Res Commun. 2002. 293:1364–1369.


8. Hemmi H, Kaisho T, Takeuchi O, Sato S, Sanjo H, Hoshino K, Horiuchi T, Tomizawa H, Takeda K, Akira S. Small anti-viral compounds activate immune cells via the TLR7 MyD88-dependent signaling pathway. Nat Immunol. 2002. 3:196–200.


9. Lund J, Sato A, Akira S, Medzhitov R, Iwasaki A. Toll-like receptor 9-mediated recognition of herpes simplex virus-2 by plasmacytoid dendritic cells. J Exp Med. 2003. 198:513–520.


10. Bourke E, Bosisio D, Golay J, Polentarutti N, Mantovani A. The toll-like receptor repertoire of human B lymphocytes: inducible and selective expression of TLR9 and TLR10 in normal and transformed cells. Blood. 2003. 102:956–963.


11. Wang PL, Azuma Y, Shinohara M, Ohura K. Toll-like receptor 4-mediated signal pathway induced by Porphyromonas gingivalis lipopolysaccharide in human gingival fibroblasts. Biochem Biophys Res Commun. 2000. 273:1161–1167.


12. Zhang FX, Kirschning CJ, Mancinelli R, Xu XP, Jin Y, Faure E, Mantovani A, Rothe M, Muzio M, Arditi M. Bacterial lipopolysaccharide activates nuclear factor-kappa B through interleukin-1 signaling mediators in cultured human dermal endothelial cells and mononuclear phagocytes. J Bio Chem. 1999. 274:7611–7614.
13. Kawai K, Shimura H, Minagawa M, Ito A, Tomiyama K, Ito M. Expression of functional Toll-like receptor 2 on human epidermal keratinocytes. J Dermatol Sci. 2002. 30:185–194.


14. Song PI, Park YM, Abraham T, Harten B, Zivony A, Neparidze N, Armstrong CA, Ansel JC. Human keratinocytes express functional CD14 and toll-like receptor 4. J Invest Dermatol. 2002. 119:424–432.


15. Mempel M, Voelcker V, Kollisch G, Plank C, Rad R, Gerhard M, Schnopp C, Fraunberger P, Walli AK, Ring J, Abeck D, Ollert M. Toll-like receptor expression in human keratinocytes: Nuclear Factor κB controlled gene activation by Staphylococcus aureus is toll-like receptor 2 but not toll-like receptor 4 or platelet activating factor receptor dependent. J Invest Dermatol. 2003. 121:1389–1396.


16. Baker BS, Ovigne JM, Powles AV, Corcoran S, Fry L. Normal keratinocytes express toll-like receptors 1, 2 and 5: modulation of TLR expression in chronic plaque psoriasis. Br J Dermatol. 2003. 148:670–679.
17. Fiers W, Remaut E, Devos R, Cheroutre H, Contreras R, Gheysen D, Degrave W, Stanssens P, Tavernier J, Taya Y, Content J. The human fibroblast and human immune interferon genes and their expression in homologous and heterologous cells. Philos Trans R Soc Lond B Biol Sci. 1982. 299:29–38.
18. Strausberg RL, Feingold EA, Grouse LH, Derge JG, Klausner RD, Collins FS, Wagner L, Shenmen CM, Schuler GD, Altschul SF, Zeeberg B, Buetow KH, Schaefer CF, Bhat NK, Hopkins SF, Jordan H, Moore T, Max SI, Wang SI, Wang J, Hsieh F, Diatchenko L, Marusina K, Farmer AA, Rubin GM, Hong L, Stapleton M, Soares MB, Bonaldo MF, Casavant TL, Scheetz TE, Brownstein MJ, Usdin TB, Toshiyuki S, Carninci P, Prange C, Raha SS, Loquellano NA, Peters GJ, Abramson RD, Mullahy SJ, Bosak SA, McEwan PJ, McKernan KJ, Malek JA, Gunaratne PH, Richards S, Worley KC, Hale S, Garcia AM, Gay LJ, Hulyk SW, Villalon DK, Muzny DM, Sodergren EJ, Lu X, Gibbs RA, Fahey J, Helton E, Ketteman M, Madan A, Rodrigues S, Sanchez A, Whiting M, Madan A, Young AC, Shevchenko Y, Bouffard GG, Blakesley RW, Touchman JW, Green ED, Dickson MC, Rodriguez AC, Grimwood J, Schmutz J, Myers RM, Butterfield YS, Krzywinski MI, Skalska U, Smailus DE, Schnerch A, Schein JE, Jones SJ, Marra MA; Mammalian Gene Collection Program Team. Generation and initial analysis of more than 15,000 full-length human and mouse cDNA sequences. Proc Natl Acad Sci USA. 2002. 99:16899–16903.
19. Triantafilou K, Vakakis E, Orthopoulos G, Ahmed MA, Schumann C, Lepper PM, Triantafilou M. TLR8 and TLR7 are involved in the host's immune response to human parechovirus 1. Eur J Immunol. 2005. 35:2416–2423.


20. Pivarcsi A, Bodai L, Rethi B, Kenderessy-Szabo A, Koreck A, Szell M, Beer Z, Beta-Csorgoo Z, Magocsi M, Rajnavolgyi E, Dobozy A, Kemeny L. Expression and function of Toll-like receptors 2 and 4 in human keratinocytes. Int Immunol. 2003. 15:721–730.


21. Kollisch G, Kalali BN, Voelcker V, Wallich R, Behrendt H, Ring J, Bauer S, Jakob T, Mempel M, Ollert M. Various members of Toll-like receptor family contribute to the innate immune response of human epidermal keratinocytes. Immunology. 2005. 114:531–541.
22. Lebre MC, van der Aar AM, van Baarsen L, van Capel TM, Schuitemaker JH, Kapsenberg ML, de Jong EC. Human keratinocytes express functional Toll-like receptor 3, 4, 5, and 9. J Invest Dermatol. 2007. 127:331–341.


23. Lowy DR, Androphy EJ. Freedberg IM, Eisen AZ, Wolff K, Austen KF, Goldsmith LA, Katz SI, editors. Molluscum contagiosum. Fitzpatrick's Dermatology in general medicine. 2003. 6th ed. New York: McGraw-hill;2114–2117.
24. Lowy DR, Androphy EJ. Freedberg IM, Eisen AZ, Wolff K, Austen KF, Goldsmith LA, Katz SI, editors. Warts. Fitzpatrick's Dermatology in general medicine. 2003. 6th ed. New York: McGraw-hill;2119–2131.
25. Grassegger A, Rollinger-Holzinger I, Zelger BW, Heim K, Zwierzina H, Fritsch PO, Hopfl RM. Spontaneous or interferon-gamma-induced T-cell infiltration, HLA-DR and ICAM-1 expression in genitoanal warts are associated with TH1 or mixed TH1/TH2 cytokine mRNA expression profiles. Arch Dermatol Res. 1997. 289:243–250.
26. Jackson M, McKenzie RC, Benton EC, Hunter JA, Norval M. Cytokine mRNA expression in cutaneous warts: induction of interleukin-1 alpha. Arch Dermatol Res. 1996. 289:28–34.
27. Lore K, Betts MR, Brenchley JM, Kuruppu J, Khojasteh S, Perfetto S, Roederer M, Seder RA, Koup RA. Toll-like receptor ligands modulate dendritic cells to augment cytomegalovirus- and HIV-1-specific T cell responses. J Immunol. 2003. 171:4320–4328.


28. Rudd BD, Burstein E, Duckett CS, Li X, Lukacs NW. Differential role for TLR3 in respiratory syncytial virus-induced chemokine expression. J Virol. 2005. 79:3350–3357.


29. Hengge UR, Ruzicka T. Topical immunomodulation in dermatology: potential of toll-like receptor agonists. Dermatol Surg. 2004. 30:1101–1112.


30. Alexopoulou L, Holt AC, Medzhitov R, Flavell RA. Recognition of double-stranded RNA and activation of NF-kappaB by Toll-like receptor 3. Nature. 2001. 413:732–738.
31. Doyle SE, O'Connell R, Vaidya SA, Chow EK, Yee K, Cheng G. Toll-like receptor 3 mediates a more potent antiviral response than Toll-like receptor 4. J Immunol. 2003. 170:3565–3571.


32. Tissari J, Siren J, Meri S, Julkunen I, Matikainen S. IFN-alpha enhances TLR3-mediated antiviral cytokine expression in human endothelial and epithelial cells by up-regulaing TLR3 expression. J Immunol. 2005. 174:4289–4294.
33. Matsumoto M, Funami K, Oshiumi H, Seya T. Toll-like receptor 3: a link between toll-like receptor, interferon and viruses. Microbiol Immunol. 2004. 48:147–154.


34. Iwasaki A, Medzhitov R. Toll-like receptor control of the adaptive immune responses. Nat Immunol. 2004. 5:987–995.


35. Lebre MC, Antons TC, Kalinski P, Schuitemaker JH, van Capel TM, Kapsenberg ML, de Jong EC. Double-stranded RNA-exposed human keratinocytes promote Th1 responses by inducing a Type-1 polarized phenotype in dendritic cells: role of keratinocyte-derived tumor necrosis factor alpha, type I interferons, and interleukin-18. J Invest Dermatol. 2003. 120:990–997.