Abstract
To examine regional abnormalities in the brains of patients with obsessive-compulsive disorder (OCD), we assessed the gray matter (GM) density using voxel-based morphometry (VBM). We compared magnetic resonance images (MRIs) acquired from 71 OCD patients and 71 age- and gender-matched normal controls and examined the relationship between GM density and various clinical variables in OCD patients. We also investigated whether GM density differs among the subtypes of OCD compared to healthy controls. We detected significant reduction of GM in the inferior frontal gyrus, the medial frontal gyrus, the insula, the cingulate gyrus, and the superior temporal gyrus of OCD patients. A significant increase in GM density was observed in the postcentral gyrus, the thalamus, and the putamen. Some of these regions, including the insular and postcentral gyrus, were also associated with the severity of obsessive-compulsive symptoms. These findings indicate that the frontal-subcortical circuitry is dysfunctional in OCD, and suggest that the parietal cortex may play a role in the pathophysiology of this disease.
Several studies have investigated the pathophysiology of obsessive-compulsive disorder (OCD) and found evidence of associated abnormalities in the frontal-subcortical circuits (1, 2). In particular, the orbitofrontal and anterior cingulate regions are hypothesized to play an important role in producing the symptoms of OCD. Several functional studies have identified hypermetabolism in the orbitofrontal cortex, the inferior frontal gyrus, and the anterior cingulate cortex, under baseline conditions and during symptom provocation (3, 4). Normal metabolic activity in these regions was restored with treatment (5).
Structural studies have compared regions of interest (ROI) to observe regional changes in brain volume, or used voxel-based morphometry (VBM) to investigate structural differences in the whole brain; however, the results of these studies were inconsistent. ROI-based studies reported decreased volumes of the orbitofrontal cortex and the caudate nucleus in OCD patients (6, 7), whereas Scarone et al. (8) reported that the volume of the caudate nucleus increased compared to normal controls. Other studies have found no changes in regional brain volume in OCD patients (9, 10). The number of VBM studies is limited and the findings are contradictory. A recent study reported increased gray matter (GM) in the orbitofrontal cortex (11), whereas Pujol et al. (12) reported that the GM volume was reduced in the medial frontal gyrus, the medial orbitofrontal cortex, and in the left insulo-opercular region. In addition, although several studies identified abnormalities in regions such as the parietal cortex (4, 11, 13), few ROI-based studies have addressed the same regions.
We used VBM to investigate structural abnormalities associated with OCD in comparison to healthy controls in a large number of subjects. VBM methodology has permitted investigators to examine regional differences in GM density across the entire brain without an a priori definition of anatomical borders. We hypothesized that patients with OCD would show volumetric abnormalities in specific regions, including the parietal, orbitofrontal, and cingulate cortices, as well as in some subcortical areas. We also hypothesized that abnormalities in GM density would be correlated with clinical variables, such as symptom severity, duration of illness, and symptom dimensions.
Seventy-one patients with OCD were recruited from the outpatient clinic at Seoul National University Hospital. Diagnoses were established using the Structured Clinical Interview for DSM-IV Axis I disorders (SCID-IV) (14). The participants consisted of 47 males (66.20%) and 24 females (33.80%) with a mean (±standard deviation) age of 26.60±7.49 yr. The mean age of males was 25.66±7.90 yr and that of females was 28.46±6.39 yr (t=-1.501, df=69, p=0.138). The mean age of OCD onset was 18.59±6.52 yr (males, 18.19±6.81 yr; females, 19.38±5.98 yr; t=-0.721, df=69, p=0.473). The mean duration of the illness was 8.02±6.10 yr (males, 7.55±6.32 yr; females, 8.95±5.67 yr; t=-0.972, df=69, p=0.365). Sixty-eight subjects were right-handed and three subjects were left-handed.
The Yale-Brown Obsessive Compulsive Scale (Y-BOCS) and the symptom checklist (15) were used to assess the severity and subtypes of OCD symptoms. Sixty-four of the patients had completed the symptom checklist and were assigned a score of 0 (absent), 1 (mild), or 2 (prominent) for each of the five clinical dimensions (16). The highest score for any of the checklist elements composing a given dimension was used as the overall score for that dimension; a score of 2 (prominent) was allowed for more than one dimension. The demographic and clinical characteristics of the participants are presented in Table 1. Four patients had a history of major depressive disorder (MDD) and three patients displayed anxiety disorder with comorbidity. One patient had a history of both MDD and anxiety disorder. Fifty-nine patients were taking selective serotonin reuptake inhibitors (SSRIs), and the remaining subjects were medication-free for at least 4 weeks prior to the study.
An equivalent number of healthy control subjects were recruited from the community and assessed using the SCID-IV to exclude any concurrent or lifetime history of DSM-IV Axis I disorders. The control group was age- and gender-matched to the OCD group (47 males, 24 females; mean age=26.68±6.09 yr) and all control subjects were right-handed. None of the control subjects had a history of Axis I psychiatric disorders, neurological illnesses, or medical illnesses.
The study protocol and forms were approved by the institutional review board of Seoul National University Hospital, and written informed consent was obtained from each subject.
MRIs were obtained using a 1.5-T GE SIGNA scanner (GE Medical Systems, Milwaukee, WI, U.S.A.). Contiguous 1.5-mm sagittal images were obtained with a three-dimensional T1-weighted spoiled gradient-echo sequence (echo time=5.5 ms; repetition time=14.4 ms; flip angle=20°; field of view=21×21 cm; matrix=256×256).
All MRIs were loaded into the image-processing software package ANALYZE (version 4.1; Mayo Foundation, Rochester, NY, U.S.A.) and spatially realigned along the antero-posterior axis of the brain parallel to the intercommissural line. Then, the images were filtered using anisotropic diffusion methods to improve the signal-to-noise ratio.
A VBM template was created specifically for this study, which is necessary when working with a large number of subjects (17). The realigned filtered MRIs from the OCD patients and healthy controls were spatially normalized to the ICBM 152 template (Montreal Neurological Institute, Montreal, Canada), then smoothed with an 8-mm full-width at half-maximum (FWHM) isotropic Gaussian kernel. The normalized smoothed images were averaged to provide the customized template.
All 142 MRIs were normalized to the same high-resolution stereotactic space by registering each of the images to the customized template. This process was accomplished by minimizing the residual sum of the difference between the images and the study template. These spatially normalized images were re-sliced using trilinear interpolation to a final voxel size of 2.0×2.0×2.0 µL, and then segmented into GM, white matter (WM), and cerebrospinal fluid (CSF) using a modified mixture model cluster analysis with correction for image intensity nonuniformity. The resulting images were then smoothed with a 12-mm Gaussian kernel to render the data more normally distributed. GM images were analyzed using VBM.
Intracranial volume and the volume of the three types of brain tissue (GM, WM, and CSF) were compared using independent samples t-tests. The values for GM, WM, and CSF were obtained from non-normalized images, and the intracranial volume was calculated as the sum of these three values.
The processed images for GM were compared between the OCD and control groups using the t-test group comparison in SPM2 (Statistical Parameter Mapping, version 2; MathWorks, Natick, MA, U.S.A.). In addition, GM images were compared separately according to gender. Exploratory analyses compared patients with predominant contamination/cleaning (n=26) or aggressive/checking (n=29) symptoms (the only subgroups large enough for analysis) to the healthy control subjects.
The SPM (t) maps were then transformed into the unit normal distribution (SPM [Z]) and thresholded at a voxel level of p<0.001 (uncorrected) with a cluster threshold of 20 voxels. This threshold was chosen based on several previous structural imaging studies using SPM analyses (11); it provides protection against false-positive results when clear hypotheses exist as to the location of the abnormality.
Finally, simple regression analyses were performed to determine whether a relationship exists between whole brain GM density and the clinical variables of OCD, including symptom severity and duration of the illness.
Intracranial volumes were similar in OCD patients and control subjects (1825.28±201.98 µL vs. 1818.57±201.09 µL, respectively; t140=0.198; p=0.843). No differences were found in GM, WM, or CSF volumes between OCD patients and control subjects (GM, 740.01±65.63 m3 vs. 737.75±62.69 µL, respectively; t140=0.209, p=0.834; WM, 455.89±53.54 µL vs. 452.54±49.59 µL, respectively; t140=0.387, p=0.699; CSF, 629.38±105.52 µL vs. 628.28±111.59 µL, respectively; t140=0.060, p=0.952).
Significant decreases in GM density were detected in the cingulate gyrus of patients with OCD, especially in the anterior cingulate gyrus, the frontal lobe (bilateral inferior frontal gyrus, right medial frontal gyrus, right precentral gyrus, and left middle frontal gyrus), the bilateral insular gyrus, and the left superior temporal gyrus (Fig. 1 and Table 2). In contrast, the bilateral postcentral gyrus, the right thalamus, and the left putamen showed significant increases in GM density in OCD patients (Fig. 1 and Table 2). These results remained statistically significant when we excluded patients with comorbid diagnoses from the analysis. Exploratory analyses indicated that patients with contamination/cleaning symptoms showed significantly less GM volume in the anterior cingulate gyrus, the middle frontal gyrus, and the cingulate gyrus. However, they showed increased GM volume in the medial frontal gyrus and postcentral gyrus compared to healthy controls. Patients with aggressive/checking symptoms showed less GM volume in the superior temporal gyrus, middle frontal gyrus, anterior cingulate gyrus, and insular gyrus, and increased GM volume in the right postcentral gyrus (Table 3).
Female patients displayed decreased GM density in the right middle frontal gyrus (757 voxels, Z-max=4.38, coordinatesx,y,z=54, 44, -10), the right superior frontal gyrus (73 voxels, Z-max=4.07, coordinatesx,y,z=16, 20, 48), the left precentral gyrus (153 voxels, Z-max=4.01, coordinatesx,y,z=-50, 10, 4), and the left cingulate gyrus (43 voxels, Z-max=3.62, coordinatesx,y,z=-20, 20, 26) compared to healthy female controls. Female OCD subjects displayed no regional differences in GM density compared to the healthy female controls. Male OCD subjects showed decreased GM density in the medial frontal gyrus bilaterally (891 voxels, Z-max=4.24, coordinatesx,y,z=-6, 40, 24), the right inferior frontal gyrus (87 voxels, Z-max=3.89, coordinatesx,y,z=34, 24, -6), the bilateral anterior cingulate gyrus (310 voxels, Z-max=3.71, coordinatesx,y,z=2, 18, -2), and the bilateral insular gyrus (70 voxels, Z-max=3.73, coordinatesx,y,z=-40, 18, 4; 88 voxels, Z-max=3.57, coordinatesx,y,z=42, 8, -2). Male OCD patients showed increased GM density in the right postcentral gyrus (85 voxels, Z-max=3.94, coordinatesx,y,z=16, -56, 70), the left superior parietal lobule gyrus (65 voxels, Z-max=3.70, coordinatesx,y,z=-20, -66, 58), and the left lentiform nucleus (49 voxels, Z-max=3.44, coordinatesx,y,z=-28, -12, -4), compared to healthy male controls.
We investigated whether GM density is correlated with clinical variables in OCD patients. Obsession and compulsion diagnostic scores were analyzed separately. Obsession scores were positively correlated with voxels in the right insula (47 voxels, Z-max=4.90, coordinatesx,y,z=44, -11, 21) and the precuneus (58 voxels, Z-max=3.94, coordinatesx,y,z=0, -66, 46), but negatively correlated with voxels in the right postcentral gyrus (53 voxels, Z-max=4.19, coordinatesx,y,z=63, -18, 38) and the right transverse temporal gyrus (40 voxels, Z-max=4.00, coordinatesx,y,z=63, -7, 11). We found clusters of significant positive correlation with compulsion scores in the precuneus (286 voxels, Z-max=5.30, coordinatesx,y,z=0, -64, 46), the right cingulate gyrus (45 voxels, Z-max=4.65, coordinatesx,y,z=14, 29, 28), and the left medial frontal gyrus (26 voxels, Z-max=3.25, coordinatesx,y,z=-6, -13, 52). Moreover, three clusters of negative correlation with compulsion scores were found in the left superior temporal gyrus (-63 voxels, Z-max=3.97, coordinatesx,y,z=-63, -19, -2), the superior frontal gyrus (23 voxels, Z-max=3.71, coordinatesx,y,z=-16, 18, 51), and the left anterior cingulate gyrus (38 voxels, Z-max=3.46, coordinatesx,y,z=-2, 39, -1).
The duration of illness and GM density were negatively correlated in several brain regions, primarily in the left hemisphere, including the left postcentral gyrus (217 voxels, Z-max=4.55, coordinatesx,y,z=-44, -28, 44), the left supramarginal gyrus (103 voxels, Z-max=4.25, coordinatesx,y,z=-62, -48, 24), the left precentral gyrus (314 voxels, Z-max=4.15, coordinatesx,y,z=-50, -6, 42), the left middle and superior temporal gyrus (113 voxels, Z-max=4.10, coordinatesx,y,z=-50, 4, -34; 45 voxels, Z-max=3.45, coordinatesx,y,z=-66, -24, 2), the left inferior frontal gyrus (34 voxels, Z-max=3.62, coordinatesx,y,z=-48, 24, -10), and the right inferior parietal lobule (68 voxels, Z-max=3.78, coordinatesx,y,z=60, -40, 48). No cases of significant positive correlation were observed between the duration of the illness and GM density.
We investigated abnormalities in GM density in OCD patients compared to healthy control subjects. OCD patients displayed decreased GM density in the cingulate gyrus, especially in the anterior cingulate gyrus, the frontal gyrus, the insular gyrus, and the temporal gyrus. In contrast, we noted significantly increased GM density in the postcentral gyrus, the right thalamus, and the left putamen.
The regions of the brain that showed significant differences in GM density compose the frontal-subcortical circuits, which may be involved in the pathophysiology of OCD. The anterior cingulate gyrus is hypothesized to produce the symptoms associated with OCD (18). Neuropsychological impairments revealed by the controlled oral word association test in OCD patients may be related to the of the frontaomedial-anterior cingulate dysfunction (19).
The inferior frontal gyrus, particularly the ventral area, and the medial frontal gyrus were designated as part of the orbitofrontal cortex in previous studies (1, 18). Volume reduction in the orbitofrontal cortex has been reported more frequently than that of other regions of the frontal-subcortical circuits (20). The orbitofrontal cortex is thought to play an important role in the pathophysiology of OCD through the recognition of biologically significant stimuli and the mediation of autonomic and goal-directed responses (21). In addition, Savage et al. (22) reported that verbal and nonverbal episodic memories are deficient in OCD patients, and that the orbitofrontal cortex plays a role in mobilizing strategies during novel or ambiguous verbal learning tasks, and in directing the strategic memory process. A decreased GM density in the frontal cortex may be related to the symptoms and neuropsychological impairments observed in OCD patients. The hyperactivity of the orbitofrontal cortex observed in functional imaging studies may be attributable to residual tissues compensating for decreased GM volumes.
We found increased GM density in the parietal cortex, the thalamus, and the putamen in patients with OCD, which may reflect increased neuronal size, hypertrophy, or other pathophysiological processes. Rosenberg et al. (23) suggested that increased cortical volumes in OCD may result from delayed or inefficient pruning of neurons during development. Another possible explanation is that cortical volume increases to compensate for dysfunctional frontal-striatal regions. The patients in our study were relatively young and ill for only a short period of time. These characteristics lead us to speculate that their brains may have some ability to compensate for dysfunctional regions, such as the frontal-striatal regions. This idea is supported by our finding that GM density in the parietal cortex is negatively correlated with symptom severity.
Structural and functional imaging studies have implicated the parietal cortex in the pathology of OCD. Valente et al. (11) reported the reduction of GM in the right parietal associative cortex, and Kwon et al. (13) observed hypometabolism in the left inferior parietal cortex in OCD patients. However, little attention has been paid to parietal cortex dysfunction and the pathophysiology of OCD. The parietal cortex is associated with several functions relevant to the clinical expression of OCD, including the dynamic aspects of executive functioning, set shifting, goal setting in planned behavior, inhibition, and visuospatial processing, suggesting that parietal dysfunction may underlie the cognitive deficits observed in patients with OCD (24, 25). Moreover, dysfunction of the parietal cortex may interact with some of the key regions implicated in OCD via direct anatomical connections; these regions may include the lateral OFC (21), the striatum (26), and the mediodorsal thalamic nucleus (27). These findings suggest that the parietal cortex, as well as the frontal-subcortical circuits, play a role in expressing the clinical symptoms of OCD.
We observed gender-dependent differences in GM density between OCD patients and healthy controls. Previous studies have shown gender-related differences in patients with MDD (28), schizophrenia (29), and bipolar disorder (30). These differences may be the result of various factors, including normal sexual dimorphism (31) and hormone effects (32). Further studies are required to determine the relationship between the gender-specific characteristics of OCD and brain morphometry.
We also found that symptom dimensions were associated with different regions, which was previously noted in several functional imaging studies (16, 33). One study used a washing experiment to demonstrate that patients exhibited differences in blood flow, primarily in the ventromedial prefrontal regions. In contrast, differences in blood flow were found primarily in the subthalamic and dorsal cortical regions in checking experiments (32). These results suggest that different OCD symptom dimensions are mediated by relatively distinct, but partially overlapping, neural components of the fronto-striato-thalamic circuits.
We found that symptom scores were positively correlated with decreased GM density in the cingulate gyrus and the medial frontal gyrus. These results appear to be in conflict with each other. However, decreased GM density may be associated with the pathophysiology of OCD but not the symptoms; instead, symptoms may be produced by the remaining functional regions. In our study, lower GM density was associated with an extended duration of illness.
This study had several limitations. First, the majority of OCD patients in this study were taking medication. Several studies have suggested that medication affects regional brain volumes (34, 35). We cannot exclude the possibility that at least some of the changes in GM density observed in OCD patients might have been related to the medication. Second, our findings did not perfectly replicate the results of previous ROI studies (7, 10). Several previous studies have reported differences between the results of VBM studies and those of manual ROI analyses (36, 37). False-positive or false-negative VBM results may arise from changes in the shape or displacement of structures during spatial normalization (37); in addition, ROI analyses may inadvertently include portions of the surrounding white matter (36). Finally, the discrepancies among various structural studies may be attributable to the nature of OCD itself. Several studies have shown that OCD is a multidimensional and etiologically heterogeneous condition. The symptom dimensions of OCD, such as doubt/checking, contamination/washing, and symmetry/ordering, may be useful in genetic, neurobiological, and treatment response studies (38, 39).
In summary, our study found abnormalities in the parietal cortex as well as the frontal-subcortical circuits of patients with OCD, and distinct GM abnormalities associated with the symptom dimensions of OCD. Further studies are required to elucidate the role of the parietal cortex in OCD.
Figures and Tables
![]() | Fig. 1Statistical parametric mapping displaying gray matter (GM) density differences between patients with obsessive-compulsive disorder (OCD) and healthy controls. Significant changes of GM density with cluster of more than 20 voxels at <0.001, uncorrected for multiple comparisons are displayed. Note that significant decreases of GM density are identified in the right cingulate gyrus, bilateral frontal lobe, bilateral insular, and left superior temporal gyrus (A), whereas GM densities of the bilateral postcentral gyrus, right thalamus, and left putamen are significantly increased in patients with OCD (B) The color scale shows t values for each significant voxel. |
Table 1
Demographic and clinical characteristics of patients with obsessive-compulsive disorder (OCD) and control subjects
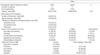
Table 2
Regional gray matter density changes in patients with obsessive-compulsive disorder
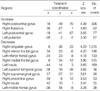
Coordinates (x, y, z) refer to the brain atlas of Talairach and Tournoux (40). Only clusters showing a spatial extent of at least 20 contiguous voxels are reported.
Table 3
Differences in regional gray matter density between healthy controls and OCD patients with the prominent symptom dimensions
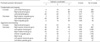
*Prominent symptom dimensions were defined by Mataix-Cols et al. (16) The comparisons were done between patients with and without each prominent symptom. †Coordinates (x, y, z) refer to the brain atlas of Talairach and Tournoux (40). Only clusters showing a spatial extent of at least 20 contiguous voxels are reported. OCD, obsessive-compulsive disorder.
References
1. Kang DH, Kim JJ, Choi JS, Kim YI, Kim CW, Youn T, Han MH, Chang KH, Kwon JS. Volumetric investigation of the frontal-subcortical circuitry in patients with obsessive-compulsive disorder. J Neuropsychiatry Clin Neurosci. 2004. 16:342–349.


2. Saxena S, Brody AL, Schwartz JM, Baxter LR. Neuroimaging and frontal-subcortical circuitry in obsessive-compulsive disorder. Br J Psychiatry Suppl. 1998. 35:26–37.


3. Swedo SE, Schapiro MB, Grady CL, Cheslow DL, Leonard HL, Kumar A, Friedland R, Rapoport SI, Rapoport JL. Cerebral glucose metabolism in childhood-onset obsessive-compulsive disorder. Arch Gen Psychiatry. 1989. 46:518–523.


4. McGuire PK, Bench CJ, Frith CD, Marks IM, Frackowiak RS, Dolan RJ. Functional anatomy of obsessive-compulsive phenomena. Br J Psychiatry. 1994. 164:459–468.


5. Sachdev PS, McBride R, Loo CK, Mitchell PB, Malhi GS, Croker VM. Right versus left prefrontal transcranial magnetic stimulation for obsessive-compulsive disorder: a preliminary investigation. J Clin Psychiatry. 2001. 62:981–984.
6. Robinson D, Wu H, Munne RA, Ashtari M, Alvir JM, Lerner G, Koreen A, Cole K, Bogerts B. Reduced caudate nucleus volume in obsessive-compulsive disorder. Arch Gen Psychiatry. 1995. 52:393–398.


7. Szeszko PR, Robinson D, Alvir JM, Bilder RM, Lencz T, Ashtari M, Wu H, Bogerts B. Orbital frontal and amygdala volume reductions in obsessive-compulsive disorder. Arch Gen Psychiatry. 1999. 56:913–919.


8. Scarone S, Colombo C, Livian S, Abbruzzese M, Ronchi P, Locatelli M, Scotti G, Smeraldi E. Increased right caudate nucleus size in obsessive-compulsive disorder: detection with magnetic resonance imaging. Psychiatry Res. 1992. 45:115–121.


9. Kellner CH, Jolley RR, Holgate RC, Austin L, Lydiard RB, Laraia M, Ballenger JC. Brain MRI in obsessive-compulsive disorder. Psychiatry Res. 1991. 36:45–49.


10. Riffkin J, Yucel M, Maruff P, Wood SJ, Soulsby B, Olver J, Kyrios M, Velakoulis D, Pantelis C. A manual and automated MRI study of anterior cingulate and orbito-frontal cortices, and caudate nucleus in obsessive-compulsive disorder: comparison with healthy controls and patients with schizophrenia. Psychiatry Res. 2005. 138:99–113.


11. Valente AA Jr, Miguel EC, Castro CC, Amaro E Jr, Duran FL, Buchpiguel CA, Chitnis X, McGuire PK, Busatto GF. Regional gray matter abnormalities in obsessive-compulsive disorder: a voxel-based morphometry study. Biol Psychiatry. 2005. 58:479–487.


12. Pujol J, Soriano-Mas C, Alonso P, Cardoner N, Menchon JM, Deus J, Vallejo J. Mapping structural brain alterations in obsessive-compulsive disorder. Arch Gen Psychiatry. 2004. 61:720–730.


13. Kwon JS, Kim JJ, Lee DW, Lee JS, Lee DS, Kim MS, Lyoo IK, Cho MJ, Lee MC. Neural correlates of clinical symptoms and cognitive dysfunctions in obsessive-compulsive disorder. Psychiatry Res. 2003. 122:37–47.


14. First M, Spitzer R, Bibbon M, Williams J. Structured Clinical Interview for DSM-IV Axis I disorders. 1996. New York, NY: New York State Psychiatric Institute, Biometrics Research.
15. Goodman WK, Price LH, Rasmussen SA, Mazure C, Fleischmann RL, Hill CL, Heninger GR, Charney DS. The Yale-Brown Obsessive Compulsive Scale. I. Development, use, and reliability. Arch Gen Psychiatry. 1989. 46:1006–1011.
16. Alonso P, Menchon JM, Pifarre J, Mataix-Cols D, Torres L, Salgado P, Vallejo J. Long-term follow-up and predictors of clinical outcome in obsessive-compulsive patients treated with serotonin reuptake inhibitors and behavioral therapy. J Clin Psychiatry. 2001. 62:535–540.


17. Good CD, Johnsrude IS, Ashburner J, Henson RN, Friston KJ, Frackowiak RS. A voxel-based morphometric study of ageing in 465 normal adult human brains. Neuroimage. 2001. 14:21–36.


18. Rauch SL, Jenike MA, Alpert NM, Baer L, Breiter HC, Savage CR, Fischman AJ. Regional cerebral blood flow measured during symptom provocation in obsessive-compulsive disorder using oxygen 15-labeled carbon dioxide and positron emission tomography. Arch Gen Psychiatry. 1994. 51:62–70.


19. Kim MS, Park SJ, Shin MS, Kwon JS. Neuropsychological profile in patients with obsessive-compulsive disorder over a period of 4-month treatment. J Psychiatr Res. 2002. 36:257–265.


20. Choi JS, Kang DH, Kim JJ, Ha TH, Lee JM, Youn T, Kim IY, Kim SI, Kwon JS. Left anterior subregion of orbitofrontal cortex volume reduction and impaired organizational strategies in obsessive-compulsive disorder. J Psychiatr Res. 2004. 38:193–199.


21. Zald DH, Kim SW. Anatomy and function of the orbital frontal cortex, II: Function and relevance to obsessive-compulsive disorder. J Neuropsychiatry Clin Neurosci. 1996. 8:249–261.
22. Savage CR, Deckersbach T, Wilhelm S, Rauch SL, Baer L, Reid T, Jenike MA. Strategic processing and episodic memory impairment in obsessive compulsive disorder. Neuropsychology. 2000. 14:141–151.


23. Rosenberg DR, Keshavan MS. A.E. Bennett Research Award. Toward a neurodevelopmental model of of obsessive--compulsive disorder. Biol Psychiatry. 1998. 43:623–640.
24. Clark CR, Egan GF, McFarlane AC, Morris P, Weber D, Sonkkilla C, Marcina J, Tochon-Danguy HJ. Updating working memory for words: a PET activation study. Hum Brain Mapp. 2000. 9:42–54.


25. Huettel SA, Misiurek J, Jurkowski AJ, McCarthy G. Dynamic and strategic aspects of executive processing. Brain Res. 2004. 1000:78–84.


26. Yeterian EH, Pandya DN. Striatal connections of the parietal association cortices in rhesus monkeys. J Comp Neurol. 1993. 332:175–197.


27. Giguere M, Goldman-Rakic PS. Mediodorsal nucleus: areal, laminar, and tangential distribution of afferents and efferents in the frontal lobe of rhesus monkeys. J Comp Neurol. 1988. 277:195–213.


28. Frodl T, Meisenzahl EM, Zetzsche T, Born C, Groll C, Jager M, Leinsinger G, Bottlender R, Hahn K, Moller HJ. Hippocampal changes in patients with a first episode of major depression. Am J Psychiatry. 2002. 159:1112–1118.


29. Pearlson GD, Marsh L. Structural brain imaging in schizophrenia: a selective review. Biol Psychiatry. 1999. 46:627–649.


30. Lochhead RA, Parsey RV, Oquendo MA, Mann JJ. Regional brain gray matter volume differences in patients with bipolar disorder as assessed by optimized voxel-based morphometry. Biol Psychiatry. 2004. 55:1154–1162.


31. Goldstein JM, Seidman LJ, Horton NJ, Makris N, Kennedy DN, Caviness VS Jr, Faraone SV, Tsuang MT. Normal sexual dimorphism of the adult human brain assessed by in vivo magnetic resonance imaging. Cereb Cortex. 2001. 11:490–497.


32. McEwen BS. Permanence of brain sex differences and structural plasticity of the adult brain. Proc Natl Acad Sci USA. 1999. 96:7128–7130.


33. Rauch SL, Wedig MM, Wright CI, Martis B, McMullin KG, Shin LM, Cannistraro PA, Wilhelm S. Functional magnetic resonance imaging study of regional brain activation during implicit sequence learning in obsessive-compulsive disorder. Biol Psychiatry. 2007. 61:330–336.


34. Adler CM, DelBello MP, Jarvis K, Levine A, Adams J, Strakowski SM. Voxel-based study of structural changes in first-episode patients with bipolar disorder. Biol Psychiatry. 2007. 61:776–781.


35. Molina V, Reig S, Sanz J, Palomo T, Benito C, Sanchez J, Sarramea F, Pascau J, Desco M. Increase in gray matter and decrease in white matter volumes in the cortex during treatment with atypical neuroleptics in schizophrenia. Schizophr Res. 2005. 80:61–71.


36. Job DE, Whalley HC, McConnell S, Glabus M, Johnstone EC, Lawrie SM. Voxel-based morphometry of grey matter densities in subjects at high risk of schizophrenia. Schizophr Res. 2003. 64:1–13.


37. Kubicki M, Shenton ME, Salisbury DF, Hirayasu Y, Kasai K, Kikinis R, Jolesz FA, McCarley RW. Voxel-based morphometric analysis of gray matter in first episode schizophrenia. Neuroimage. 2002. 17:1711–1719.


38. Leckman JF, Grice DE, Boardman J, Zhang H, Vitale A, Bondi C, Alsobrook J, Peterson BS, Cohen DJ, Rasmussen SA, Goodman WK, McDougle CJ, Pauls DL. Symptoms of obsessive-compulsive disorder. Am J Psychiatry. 1997. 154:911–917.
39. Summerfeldt LJ, Richter MA, Antony MM, Swinson RP. Symptom structure in obsessive-compulsive disorder: a confirmatory factor-analytic study. Behav Res Ther. 1999. 37:297–311.


40. Talairach J, Tournoux P. Co-planar Sterotaxic Atlas of hte Human Brain. 1988. New York, NY: Thieme Meical Publishers.