Abstract
Disease relapse after autologous peripheral blood stem cell transplantation (APBSCT) is the main cause of treatment failure in high-risk neuroblastoma (NBL). To reduce relapse, various efforts have been made such as CD34+ selection and double APBSCT. Here the authors reviewed the clinical features and outcomes of high-risk NBL patients and analyzed their survival. The medical records of 36 patients with stage III or IV NBL who underwent APBSCT at Seoul National University Children's Hospital between May 1996 and May 2004 were reviewed. Total 46 APBSCTs were performed in 36 patients. Disease free survival (DFS) and overall survival of all patients were 47.7% and 68.8%, respectively. The patients were allocated to three groups according to the APBSCT type. The DFS of CD34+ non-selected single APBSCT patients (N=13), CD34+ selected single APBSCT patients (N=14), and CD34+ selected double APBSCT patients (N=9) were 55.6%, 40.6%, and 50.0%, respectively, which were not significantly different. Thus the survival was not found to be affected by CD34+ selection or transplantation number. To improve long-term survival, various efforts should be made such as chemotherapy dose intensification, more effective tumor purging, and control of minimal residual disease via the use of differentiating and immune-modulating agents.
High-dose chemotherapy with stem cell rescue has been shown to improve outcome in patients with high-risk neuroblastoma (NBL). In previous studies, patients who underwent consolidation chemoradiotherapy and autologous stem cell transplantation showed significantly higher survival than patients who continued chemotherapy (1-5). However, more than 50% of patients relapse after autologous peripheral blood stem cell transplantation (APBSCT), and this is the major cause of treatment failure in NBL. To increase survival, various efforts have been made, such as tumor purging in the mobilized products during stem cell mobilization, chemotherapy dose intensification, and various new post-transplantation therapies to control minimal residual disease. Some of the therapeutic approaches in combination with 13-cis-retinoic acid (13-cis-RA) have shown promising results in terms of survival improvement. On the other hand, some widely used treatment modalities, such as tandem transplantation and IL-2 therapy, are still controversial.
In this study, we analyzed the clinical features and outcomes of stage III and IV NBL patients who underwent APBSCT at Seoul National University Children's Hospital (SNUCH). Survival was analyzed according to the APBSCT type, especially with respect to the effect of CD34+ selection and transplantation number on survival. Various other prognostic factors, such as age, primary mass location, bone marrow metastasis, and pre-transplantation disease status, were also analyzed.
A retrospective analysis was performed on 36 NBL patients who underwent APBSCT between May 1996 and May 2004. Patients were allocated to three groups according to the APBSCT type, i.e., to the CD34+ nonselected single APBSCT group (13 patients; the nonselected single group), the CD34+ selected single APBSCT group (14 patients; the selected single group), and to the CD34+ selected double APBSCT group (9 patients; the selected double group). One patient in the selected single group underwent APBSCT twice, before and after relapse, and thus 46 transplants were performed in 36 patients. A detailed review of clinical data was performed to ascertain the age at diagnosis, sex, tumor location, extent of tumor, stage, N-myc gene copy number, disease status at transplantation, number of APBSCTs, CD34+ selection, conditioning regimen, number of cells infused, hematopoietic reconstitution, post-transplantation complications, post-transplantation therapy, and outcome.
All patients received chemotherapy before APBSCT. After 4 or 5 cycles of chemotherapy, debulking operation was performed and local radiotherapy and chemotherapy followed. The CCG-321-P2 chemotherapy regimen (6) was used in nearly all patients, which consisted of cisplatin, VP-16, doxorubicin, and cyclophosphamide.
To mobilize stem cells, 10 µg/kg of G-CSF was injected subcutaneously (s.c.) from 7 days after the start of mobilization chemotherapy, which consisted of 1,000 mg/m2 cyclophosphamide and 150 mg/m2 etoposide on days 0, 1, and 2, until the last day of peripheral blood stem cell (PBSC) collection. PBSC collection began from the day when the WBC count exceeded 1,000/µL after a nadir and was continued for 2 or 3 days to obtain ≥6×108/kg of mononuclear cells (MNCs). In most patients stem cells were collected by apheresis, and bone marrow harvest and apheresis were combined in two APBSCTs. Leukapheresis was performed using CS-3000 Plus (Baxter Healthcare, Deerfield, IL, U.S.A.) or COBE Spectra (COBE Laboratories, Lakewood, CO, U.S.A.). In 23 of 36 patients, CD34+ selection was performed using a CliniMACS (Miltenyi Biotec, Bergisch Gladbach, Germany) device to purge tumor cells from mobilized PBSCs.
Various conditioning regimens were used and are summarized in Table 1. The main conditioning regimen was the MEC regimen, which consisted of carboplatin 400 mg/m2 on days -8 to -5, etoposide 200 mg/m2 on days -8 to -5, melphalan 140 mg/m2 on day -7 and 70 mg/m2 on day -6. This regimen was used in 30 transplants. Total body irradiation (TBI) was not performed in any case of APBSCT.
All patients received interleukin-2 (IL-2; aldesleukin; Proleukin, Chiron, Emeryville, CA, U.S.A.) and 13-cis-RA. Recombinant IL-2 at 3×106 IU/m2 was delivered s.c. once daily for 5 consecutive days every other week for 11 weeks (total 30 doses), starting when the absolute neutrophil count (ANC) and the platelet count exceeded 1,000/µL and 50,000/µL, respectively. Patients began to receive 13-cis-RA 100 mg/m2 once daily 28 days after APBSCT, and this was maintained for 1 yr.
The numbers of CD34+ cells infused in two groups were compared using the Mann-Whitney test. The correlation between infused cell doses and hematopoietic reconstitution was analyzed using Pearson's correlation coefficients. Disease-free survival (DFS) and overall survival (OS) were estimated using the Kaplan-Meier curves, and the curves were compared using the log-rank test. Statistical significance was accepted at the p<0.05 level.
The enrolled patients consisted of 25 males and 11 females with a median age at diagnosis of 3 yr (7 months-10 yr 1 month). Five patients were less than 1 yr old. The N-myc gene amplification test was performed in 11 patients, and 5 patients demonstrated amplification. Of 5 patients, 1 patient belonged to the nonselected group, 3 to the selected single group, and 1 patient to the selected double group. Thirty four patients were in stage IV NBL, and only 2 patients were in stage III NBL. To reduce tumor cell contamination, CD34+ selection was performed in 23 patients. In case who underwent double APBSCT, PBSCs were collected before the first transplantation. The median time from diagnosis to transplantation was 10 months (5-46 months), and the median time between the 1st and 2nd transplantation was 3 months (2-3 months). The median follow-up durations from diagnosis and from APBSCT were 42 months (11-103 months) and 27 months (1-93 months), respectively. Patient characteristics are summarized in Table 2.
Of the 46 transplants, CD34+ selected products were used in 30 transplants, and nonselected products were used in 14. In 2 transplants, selected and nonselected mobilized products were infused together, and thus, the analyses concerning the number of cells infused and hematopoietic reconstitution did not include these two transplants. However, in the survival analysis, one of these patients was included in the selected single group because most cells infused were collected by CD34+ selected mobilization. The other patient was included in the selected double group because half of the cells infused in the 1st transplantation and all cells infused in the 2nd transplantation were collected by CD34+ selected mobilization, although selected and nonselected products were infused together in the 1st transplantation. After selection, the mean purity of the CD34+ cell fraction was 92.4% (81.4-99.5%).
In CD34+ selected and nonselected transplants, the median doses of CD34+ cells infused were 4.4×106/kg (0.9-45.8×106/kg) and 14.9×106/kg (3.8-77.1×106/kg), respectively, and the CD34+ cell dose was significantly higher in the nonselected group (p=0.001). In selected transplants, the median number of days required for an ANC higher than 500/µL, or 1,000/µL and a platelet count of higher than 20,000/µL were 11 days (8-20 days), 12 days (9-25 days), and 17 days (10-219 days), respectively. In nonselected transplants, the median number of days required for the same conditions were 11 days (8-15 days), 11 days (8-16 days), and 18 days (8-99 days), respectively. In 3 patients who belonged to the CD34+ selected group, platelet recovery of more than 20,000/µL was not achieved until 100 days after APBSCT. No significant difference was found between the CD34+ selected and nonselected groups, in terms of the number of days required for hematopoietic reconstitution, and infused CD34+ cell doses were not found to be related to hematopoietic reconstitution (p>0.1).
After APBSCT, fever developed in all patients, and venoocclusive disease or hemorrhagic complication (gastroinfestinal weeding or pulmonary hemorrhage) occurred in 7 and 5 patients, respectively. One patient died of disease progression before the 2nd scheduled APBSCT, and 2 patients died of treatment-related conditions, i.e., acute renal failure and pulmonary hemorrhage in both cases. Most patients experienced vomiting, diarrhea, and oral mucositis.
DFS and OS values for all patients were 47.7±9.6% and 68.8±9.1%, respectively (Fig. 1). The DFSs of the nonselected single, the selected single, and the selected double groups were 55.6±14.9%, 40.6±14.7%, and 50.0±20.4%, respectively (Fig. 2A), and OSs of the three groups were 92.3±7.4%, 55.1±13.9%, and 66.7±19.3%, respectively, none of which were significantly different between the three groups. When all patients were dichotomized according to CD34+ selection (selection N=23, nonselection N=13), the DFSs of the selected and nonselected groups were 42.6±12.4% and 55.6±14.9%, respectively (Fig. 2B), and the relapse rates of these two groups were not significantly different (p=0.536).
Various prognostic factors likely to influence DFS were analyzed. Patients younger than 1 yr had a better prognosis than older patients (p=0.041), but prognosis was not significantly affected by the primary mass location, bone marrow metastasis, or pre-transplantation disease status. Moreover, the DFS of patients who underwent double APBSCT was similar to that of patients who underwent single APBSCT (p=0.499).
High-dose chemotherapy with APBSCT has improved the survival of high-risk NBL patients over past decades. However, a large number of patients relapse after APBSCT despite having a complete remission disease status before APBSCT (7-9). Reinfused tumor cells are believed to play an important role in relapse, particularly in patients with no detectable systemic residual disease (10-13). Moreover, increasing numbers of studies show that up to 50% of bone marrow and PBSC harvests from patients with bone marrow remission contain tumor cells (1, 14, 15). Because NBL cell contamination of infused PBSCs is strongly correlated with relapse risk after APBSCT, effective tumor purging is essential to improve survival in the high-risk NBL. The most widely used purging method is based on selection for CD34 antigen, which is expressed on hematopoietic stem cells. In many studies, 2-4 logs of tumor cell removal was observed after CD34+ selection (14, 16-18). This method has been generally used in NBL patients because NBL cells are believed not to express CD34 antigen. However, Hafer et al. revealed that some NBL cell lines do express CD34 antigen and recommended that caution should be taken with transplants obtained by CD34+ selection (19). In addition, EFS was not found to be statistically different between CD34+ selected and nonselected patients in some reports (4, 7, 8). In the present study, the DFSs of CD34+ selected and nonselected groups were not statistically different (p=0.536). Randomized large-scale clinical trials are required to defermine whether NBL patient survival rates can be increased by CD34+ selection, and a more effective tumor purging method is required to prevent the tumor contamination of mobilized products.
Chemotherapy dose is also intensified to reduce relapse. Today, myeloablative therapy has become the standard treatment for high-risk NBL since the Children's Cancer Group reported the result of a randomized trial which compared myeloablative therapy and autologous bone marrow transplantation with chemotherapy alone (2). Three-year event-free survival (EFS) was found to be significantly better in patients randomized to the transplantation arm than in patients randomized to continuous chemotherapy alone (34±4% vs. 22±4%, respectively; p=0.034). Moreover, the suggestion that high doses of chemotherapy might improve OS in patients with high-risk NBL has led some investigators to explore tandem transplantation, which is a novel way of increasing dose intensity for the treatment of chemosensitive tumors. The theoretical advantages of this include the exposure of tumors to multiple effective agents at maximum-tolerated doses and the possible modulation of the tumor microenvironment during the first transplantation, thus potentiating cell killing during the second transplantation (20). Tandem transplantation has been studied mainly in adults with breast cancer, lymphoma, and multiple myeloma (21-23), and few studies have been conducted in children. The earliest work used a double harvest/double graft approach with purged autologous bone marrow support (24). In this study, OS was encouraging, but when bone marrow was used for stem cell support, delayed engraftment and a high toxic death rate after the second graft were identified as potential major problems. In 2000, Grupp et al. first reported the results of tandem transplantation using PBSC in a pediatric cohort (20). Thirty-three high-risk NBL patients completed two myeloablative therapies with stem cell rescue and achieved a 3-yr EFS of 58%. These investigators demonstrated that PBSC-supported tandem transplantation in high-risk NBL is feasible with acceptable toxicity and adequate hematopoietic recovery. Subsequently, several investigators found that PBSC-supported double/triple tandem cycles of high-dose chemotherapy achieved higher EFS than single transplantation (4, 25, 26). Nevertheless, 3- and 5-yr EFSs were still below 60%. According to our results, DFS is not dependent on the number of transplantations. However, whether tandem (double or triple) transplantations with PBSC support have an impact on long-term EFS in high-risk NBL remains controversial. A phase III randomized study on the effect of tandem transplantation with PBSC rescue in patients with high-risk NBL is currently under way by the Children's Oncology Group.
The eradication of minimal residual disease by post-transplantation therapy is now considered important for the improvement of survival in the high-risk NBL. Various post-transplantation therapies have been used including IL-2 and 13-cis-RA (isotretinoin), the latter of which is derived from naturally occurring all-trans-retinoic acid (ATRA) by terminal carboxyl group modification. When NBL cell lines were exposed to 13-cis-RA in vitro, it was found to decrease proliferation and N-myc oncogene expression, and to induce the morphologic differentiation of tumor cells (27-29). In 1995, a phase I study of children with high-risk NBL showed that 13-cis-RA administered after bone marrow transplantation had minimal toxicity and that it cleared NBL cells from bone marrow (30). In 1999, a nationwide phase III trial (CCG-3891) was conducted, and found that the 3-yr EFS was significantly better in patients who received 13-cis-RA after transplantation than in those who did not (46±6% vs. 29±5%, p=0.027) (2). Moreover, the effect of 13-cis-RA was found to be the most pronounced in a setting of truly minimal residual disease because the positive benefit of 13-cis-RA was not evident in children with histologically proven residual disease. Today, maintenance therapy with 13-cis-RA after myeloablative therapy has become a standard part of high-risk NBL therapy.
Immune system modulation to stimulate an antitumor response is considered a therapeutic alternative, and the potent immunostimulatory cytokine IL-2 has been investigated in this context in a number of tumor models (31, 32). In some clinical trials, an antitumor effect has been demonstrated in children with NBL (33-35), but the effect of IL-2 remains controversial (36, 37). Further studies are needed before IL-2 is widely used as a standard post-transplantation therapy.
In conclusion, although significant survival improvements have been observed in high-risk NBL patients, high relapse rates after transplantation remain a challenge. It is evident that more complete local tumor control is essential to reduce relapse rates, and that more aggressive surgical resection followed by more intensive chemotherapy is needed. In addition, the effectiveness of tandem transplantation should be confirmed using intensified regimens with tolerable toxicities. During mobilization, much effort should be made to reduce tumor contamination. It is important to develop a more effective tumor purging method as well as to confirm the effectiveness of CD34+ selection. In addition, the control of minimal residual disease after transplantation may contribute substantially to long-term survival. Drugs that accelerate NBL cell differentiation and modulate immune response should be developed, and various approaches to improve survival are possible by adopting pre- and post-transplantation therapies. Our study is limited because the number of patients was small, and risk factors, such as N-myc gene copy number, were not thoroughly examined, which made it difficult to evaluate the true effect of the type of APBSCT on survival. However, it does provide a worthwhile comparison of the survival rates of various treatment cohorts according to the type of APBSCT. A prospective study involving a larger population would provide a better overview of the comparative effects of treatment modalities.
Figures and Tables
Fig. 1
Kaplan-Meier probabilities of DFS and OS for high-risk neuroblastoma patients after APBSCT (N=36). DFS was 47.7±9.6% and OS 68.8±9.1%. Ticks indicate censored data.
DFS, disease-free survival; OS, overall survival; APBSCT, autologous peripheral blood stem cell transplantation; PBSCT, peripheral blood stem cell transplantation.
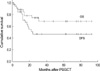
Fig. 2
DFSs of patients according to (A) the type of APBSCT and (B) CD34+ selection. Survival curves indicated no statistical differences between the various groups. Ticks indicate censored data.
DFS, disease-free survival; APBSCT, autologous peripheral blood stem cell transplantation; PBSCT, peripheral blood stem cell transplantation.
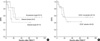
References
1. Wagner LM, Guichard SM, Burger RA, Morton CL, Straign CM, Ashmun RA, Harris LC, Houghton PJ, Potter PM, Danks MK. Efficacy and toxicity of a virus-directed enzyme prodrug therapy purging method: preclinical assessment and application to bone marrow samples from neuroblastoma patients. Cancer Res. 2002. 62:5001–5007.
2. Matthay KK, Villablanca JG, Seeger RC, Stram DO, Harris RE, Ramsay NK, Swift P, Shimada H, Black CT, Brodeur GM, Gerbing RB, Reynolds CP. Treatment of high-risk neuroblastoma with intensive chemotherapy, radiotherapy, autologous bone marrow transplantation, and 13-cis-retinoic acid. Children's Cancer Group. N Engl J Med. 1999. 341:1165–1173.
3. Stram DO, Matthay KK, O'Leary M, Reynolds CP, Haase GM, Atkinson JB, Brodeur GM, Seeger RC. Consolidation chemoradiotherapy and autologous bone marrow transplantation versus continued chemotherapy for metastatic neuroblastoma: a report of two concurrent Children's Cancer Group studies. J Clin Oncol. 1996. 14:2417–2426.


4. George RE, Li S, Medeiros-Nancarrow C, Neuberg D, Marcus K, Shamberger RC, Pulsipher M, Grupp SA, Diller L. High-risk neuroblastoma treated with tandem autologous peripheral-blood stem cell-supported transplantation: long-term survival update. J Clin Oncol. 2006. 24:2891–2896.


5. Kim JY, Baek HJ, Han DK, Sung JS, Nam TK, Yoon MS, Kook H, Hwang TJ. Improved survival in neuroblastoma by autologous peripheral blood stem cell transplantation: a single institution experience. Clin Pediatr Hematol-Oncol. 2006. 13:9–21.
6. O'leary MC, Sather HN, Ramsay NK, Harris R, Strandjord S, Romansdy S, Hasse G, Shimada H, Novak L, Siegel S, Hann HW, Brodeur G, Seeger R. Intensive chemotherapy for poor prognosis neuroblastoma. Proc Am Assoc Cancer Res. 1990. 31:201.
7. Pole JG, Casper J, Elfenbein G, Gee A, Gross S, Janssen W, Koch P, Marcus R, Pick T, Shuster J, Spruce W, Thomas P, Yeager A. High-dose chemoradiotherapy supported by marrow infusions for advanced neuroblastoma: a Pediatric Oncology Group study. J Clin Oncol. 1991. 9:152–158.


8. Ryu KH, Ahn HS, Koo HH, Kook H, Kim MK, Kim HK, Ghim T, Moon HN, Seo JJ, Sung KW, Shin HY, Yoo ES, Lyu CJ, Lee YH, Lee H, Cho B, Cho HS, Choi HS, Hah JO, Hwang TJ. Autologous stem cell transplantation for the treatment of neuroblastoma in Korea. J Korean Med Sci. 2003. 18:242–247.


9. Imaizumi M, Watanabe A, Kikuta A, Takano T, Ito E, Shimizu T, Tsuchiya S, Iinuma K, Konno T, Ohi R, Hayashi Y. Improved survival of children with advanced neuroblastoma treated by intensified therapy including myeloablative chemotherapy with stem cell transplantation: a retrospective analysis from the Tohoku Neuroblastoma Study Group. Tohoku J Exp Med. 2001. 195:73–83.


10. Shimoni A, Korbling M. Tumor cell contamination in re-infused stem cell autografts: does it have clinical significance? Crit Rev Oncol Hematol. 2002. 41:241–250.


11. Rill DR, Santana VM, Roberts WM, Nilson T, Bowman LC, Krance RA, Heslop HE, Moen RC, Ihle JN, Brenner MK. Direct demonstration that autologous bone marrow transplantation for solid tumors can return a multiplicity of tumorigenic cells. Blood. 1994. 84:380–383.


12. Moss TJ, Cairo M, Santana VM, Weinthal J, Hurvitz C, Bostrom B. Clonogenicity of circulating neuroblastoma cells: implications regarding peripheral blood stem cell transplantation. Blood. 1994. 83:3085–3089.


13. Brenner MK, Rill DR, Moen RC, Krance RA, Mirro J Jr, Anderson WF, Ihle JN. Gene-marking to trace origin of relapse after autologous bone-marrow transplantation. Lancet. 1993. 341:85–86.


14. Handgretinger R, Greil J, Schurmann U, Lang P, Gonzalez-Ramella O, Schmidt I, Fuhrer R, Niethammer D, Klingebiel T. Positive selection and transplantation of peripheral CD34+ progenitor cells: feasibility and purging efficacy in pediatric patients with neuroblastoma. J Hematother. 1997. 6:235–242.


15. Lode HN, Handgretinger R, Schuermann U, Seitz G, Klingebiel T, Niethammer D, Beck J. Detection of neuroblastoma cells in CD34+ selected peripheral stem cells using a combination of tyrosine hydroxylase nested RT-PCR and anti-ganglioside GD2 immunocytochemistry. Eur J Cancer. 1997. 33:2024–2030.


16. Donovan J, Temel J, Zuckerman A, Gribben J, Fang J, Pierson G, Ross A, Diller L, Grupp SA. CD34 selection as a stem cell purging strategy for neuroblastoma: preclinical and clinical studies. Med Pediatr Oncol. 2000. 35:677–682.


17. Kanold J, Halle P, Tchirkov A, Berger M, Giarratana MC, Kobari L, Boiret N, Paillard C, Demeocq F, Douay L. Ex vivo expansion of autologous PB CD34+ cells provides a purging effect in children with neuroblastoma. Bone Marrow Transplant. 2003. 32:485–488.


18. Berkahn L. Immunologic methods of purging in autologous stem cell transplantation. J Hematother Stem Cell Res. 2000. 9:147–159.
19. Hafer R, Voigt A, Gruhn B, Zintl F. Neuroblastoma cells can express the hematopoietic progenitor cell antigen CD34 as detected at surface protein and mRNA level. J Neuroimmunol. 1999. 96:201–206.
20. Grupp SA, Stern JW, Bunin N, Nancarrow C, Ross AA, Mogul M, Adams R, Grier HE, Gorlin JB, Shamberger R, Marcus K, Neuberg D, Weinstein HJ, Diller L. Tandem high-dose therapy in rapid sequence for children with high-risk neuroblastoma. J Clin Oncol. 2000. 18:2567–2575.


21. Ayash LJ, Elias A, Schwartz G, Wheeler C, Ibrahim J, Teicher BA, Reich E, Warren D, Lynch C, Richardson P, Schnipper L, Frei E 3rd, Antman K. Double dose-intensive chemotherapy with autologous stem-cell support for metastatic breast cancer: no improvement in progression-free survival by the sequence of high-dose melphalan followed by cyclophosphamide, thiotepa, and carboplatin. J Clin Oncol. 1996. 14:2984–2992.


22. Fitoussi O, Simon D, Brice P, Makke J, Scrobohaci ML, Bibi Triki T, Hennequin C, Ferme C, Gisselbrecht C. Tandem transplant of peripheral blood stem cells for patients with poor-prognosis Hodgkins's disease or non-Hodgkin's lymphoma. Bone Marrow Transplant. 1999. 24:747–755.


23. Barlogie B, Jagannath S, Vesole DH, Naucke S, Cheson B, Mattox S, Bracy D, Salmon S, Jacobson J, Crowley J, Tricot G. Superiority of tandem autologous transplantation over standard therapy for previously untreated multiple myeloma. Blood. 1997. 89:789–793.


24. Philip T, Ladenstein R, Zucker JM, Pinkerton R, Bouffet E, Louis D, Siegert W, Bernard JL, Frappaz D, Coze C, Wyss M, Beck D, Soulliet G, Michon J, Philip I, Chauvin F, Favrot M, Biron P. Double megatherapy and autologous bone marrow transplantation for advanced neuroblastoma: the LMCE2 study. Br J Cancer. 1993. 67:119–127.


25. Sung KW, Yoo KH, Chung EH, Jung HL, Koo HH, Shin HJ, Lee SK, Lim DH, Kim DW, Park HK, Cho EJ, Kim SW. Successive double high-dose chemotherapy with peripheral blood stem cell rescue collected during a single leukapheresis round in patients with high-risk pediatric solid tumors: a pilot study in a single center. Bone Marrow Transplant. 2003. 31:447–452.


26. Kletzel M, Katzenstein HM, Haut PR, Yu AL, Morgan E, Reynolds M, Geissler G, Marymount MH, Liu D, Kalapurakal JA, Shore RM, Bardo DM, Schmoldt J, Rademaker AW, Cohn SL. Treatment of high-risk neuroblastoma with triple-tandem high-dose therapy and stem-cell rescue: results of the Chicago Pilot II Study. J Clin Oncol. 2002. 20:2284–2292.


27. Sidell N. Retinoic acid-induced growth inhibition and morphologic differentiation of human neuroblastoma cells in vitro. J Natl Cancer Inst. 1982. 68:589–596.
28. Thiele CJ, Reynolds CP, Israel MA. Decreased expression of N-myc precedes retinoic acid-induced morphological differentiation of human neuroblastoma. Nature. 1985. 313:404–406.


29. Abemayor E. The effects of retinoic acid on the in vitro and in vivo growth of neuroblastoma cells. Laryngoscope. 1992. 102:1133–1149.


30. Villablanca JG, Khan AA, Avramis VI, Seeger RC, Matthay KK, Ramsay NK, Reynolds CP. Phase I trial of 13-cis-retinoic acid in children with neuroblastoma following bone marrow transplantation. J Clin Oncol. 1995. 13:894–901.


31. Mier JW, Vachino G, van der Meer JW, Numerof RP, Adams S, Cannon JG, Bernheim HA, Atkins MB, Parkinson DR, Dinarello CA. Induction of circulating tumor necrosis factor (TNF alpha) as the mechanism for the febrile response to interleukin-2 (IL-2) in cancer patients. J Clin Immunol. 1988. 8:426–436.
32. Oppenheim MH, Lotze MT. Interleukin-2: solid-tumor therapy. Oncology. 1994. 51:154–169.
33. Pardo N, Marti F, Fraga G, Illa J, Badell I, Peiro M, Bertran E, Garcia J, Rueda F, Cubells J. High-dose systemic interleukin-2 therapy in stage IV neuroblastoma for one year after autologous bone marrow transplantation: pilot study. Med Pediatr Oncol. 1996. 27:534–539.


34. Toren A, Nagler A, Rozenfeld-Granot G, Levanon M, Davidson J, Bielorai B, Kaplinsky C, Meitar D, Mandel M, Ackerstein A, Ballin A, Attias D, Biniaminov M, Rosenthal E, Brok-Simoni F, Rechavi G, Kaufmann Y. Amplification of immunological functions by subcutaneous injection of intermediate-high dose interleukin-2 for 2 years after autologous stem cell transplantation in children with stage IV neuroblastoma. Transplantation. 2000. 70:1100–1104.


35. Kim MK, Lyu CJ, Park SH, Cho HS, Yang CH, Kim HM, Kang SH, Kim KY. Effects of low dose interleukin-2 therapy after high dose chemotherapy and autologous PBSCT in childhood AML and stage 4 neuroblastoma. Korean J Pediatr Hematol-Oncol. 2000. 7:82–91.
36. Bauer M, Reaman GH, Hank JA, Cairo MS, Anderson P, Blazar BR, Frierdich S, Sondel PM. A phase II trial of human recombinant interleukin-2 administered as a 4-day continuous infusion for children with refractory neuroblastoma, non-Hodgkin's lymphoma, sarcoma, renal cell carcinoma, and malignant melanoma. A Childrens Cancer Group study. Cancer. 1995. 75:2959–2965.
37. Vlk V, Eckschlager T, Kavan P, Kabickova E, Koutecky J, Sobota V, Bubenik J, Pospisilova D. Clinical ineffectiveness of IL-2 and/or IFN alpha administration after autologous PBSC transplantation in pediatric oncological patients. Pediatr Hematol Oncol. 2000. 17:31–44.