Abstract
Toxoplasma gondii is an obligate intracellular protozoan parasite that induces antitumor activity against certain types of cancers. However, little information is available regarding the immunologic mechanisms that regulate these effects. For this purpose, C57BL/6 mice were administered either the T. gondii Me49 strain orally or Lewis lung carcinoma (LLC) cells intramuscularly. Survival rates, tumor size, histopathology, and immune responses were determined for each group, and angiogenesis was evaluated by in vivo Matrigel plug assay. Toxoplasma-infected (TG-injected) mice survived the entire experimental period, whereas cancer cell-bearing (LLC-injected) mice died within six weeks. Mice injected with both T. gondii and cancer cells (TG/LLC-injected group) showed significantly increased survival rates, CD8+ T-cell percentages, IFN-γ mRNA expression levels, serum IgG2a titers, and CTL responses as compared to the LLC-injected mice. In addition, angiogenesis in the TG/LLC-injected mice was notably inhibited. These effects in TG/LCC-injected mice were similar or were increased by the addition of an adjuvant, Quil-A. However, TG/LLC-injected mice showed decreased percentages of CD4+ and CD8+ T-cells, IFN-γ mRNA expression levels, and serum IgG1 and IgG2a titers as compared to TG-injected mice. Taken together, our results demonstrate that T. gondii infection inhibits tumor growth in the Lewis lung carcinoma mouse model through the induction of Th1 immune responses and antiangiogenic activity.
Lung cancer is the most prevalent and lethal cancer worldwide, and has been reported in many nations, including the Republic of Korea and many Western countries (1). The high mortality rate attributed to lung cancer may be the result of induced multi-drug resistance and a high frequency of metastatic occurrence. Despite aggressive treatment with surgery, radiation, and chemotherapy, survival rates for lung cancer patients remain low (2). New approaches to the treatment of lung cancer are urgently needed. Immunotherapy may represent one such approach, and several different strategies for lung cancer immunotherapy have recently been developed (3).
The goal of cancer immunotherapy is to augment the weakened host immune response against tumors using specific and/or nonspecific immune stimulants. Treatment may include the administration of cytokines, tumor-specific antibodies, and T-cells (3-5). Toxoplasma gondii is an obligate intracellular parasite; latent infections with T. gondii are common in human populations throughout the world (6). It has been reported that the development of spontaneous mammary tumors, leukemia, and 20-methylcholanthrene-autoinduced tumors is suppressed in animal models following injection of Toxoplasma antigen or viable parasites (7, 8). Both Toxoplasma infection and the cell-free parasite extract are able to reverse the multi-drug resistance of mouse lymphoma and human gastric cancers in vitro (9). Moreover, potent antitumor effects are induced by intralesional injection with formalin-fixed T. gondii organisms in Lewis lung carcinoma (LLC) in Toxoplasma-infected mice (10, 11).
These reports suggest that T. gondii is a powerful agent for cancer immunotherapy, and is useful as a stimulant of the cellular immune responses. Recently, there were many reports that antitumor and antimetastatic actions are associated with inhibition of tumor-induced neovascularization (12-14). However, the immunologic mechanisms of the antitumor activity elicited by T. gondii in lung cancer, as well as the immunological characteristics of LLC, are not well documented. Also, the anti-angiogenic effects of T. gondii have not been determined in the LLC mouse model. In order to determine the antitumor and anti-angiogenic activities of T. gondii infection in LLC-bearing mice, C57BL/6 mice were injected with LLC cells alone or in combination with T. gondii. And then, we evaluated the survival rates, tumor size, histopathology, cell-mediated immune responses, and angiogenesis.
Female inbred C57BL/6 mice were obtained from the Korea Research Institute of Bioscience and Biotechnology, Daejeon, Korea. All mice used were 6-8 weeks old and documented as specific pathogen-free animals. All animal studies were carried out in a pathogen-free barrier zone at the College of Medicine, Chungnam National University, in accordance with the procedures outlined in the Guide for the Care and Use of Laboratory Animals. Two strains of T. gondii were used; the RH strain was used to prepare Toxoplasma lysate antigen (TLA) and mice were orally infected with the Me49 strain to evaluate antitumor activity.
The LLC cell line was obtained from the American Type Culture Collection (ATCC, Rockville, MD, U.S.A.) and was cultured in a humidified atmosphere of 5% CO2 at 37℃ using Dulbecco's modified Eagle's medium (DMEM) (GibcoBRL Co., Grand Island, NY, U.S.A.) that contained 10% heat-inactivated fetal bovine serum (FBS; GibcoBRL), 2 mM glutamine, 100 U/mL penicillin, and 100 µg/mL streptomycin.
Mice were divided into seven experimental groups. Each group was composed of 50 mice; 10 mice to evaluate survival rates, 35 mice to evaluate immunologic characteristics, and 5 mice to check angiogenesis. The seven experimental groups included mice that were administered with: LLC cells (LLC-injected group); T. gondii (TG-injected group); both Toxoplasma parasites and LLC cells (TG/LLC-injected group); Quil-A only (QA-injected group) (Accurate Chemical and Scientific Co., Westbury, NY, U.S.A.); both LLC cells and Quil-A (LLC/QA-injected group); both T. gondii and Quil-A (TG/QA-injected group); and T. gondii, LLC cells and Quil-A (TG/LLC/QA-injected group). To calculate the survival rate for each group, the mice were examined each day for eight weeks following LLC cell injection or T. gondii infection. To evaluate the immunological mechanisms of antitumor activity induced by Toxoplasma, mice were sacrificed weekly and blood, muscle, lung, and spleen tissues were collected. In addition, we checked the antiangiogenic activity and cytotoxic T-lymphocyte responses one week after infection with T. gondii. Untreated age- and sex-matched control mice were treated with equivalent doses of normal saline.
According to the experimental protocol, mice were injected with LLC cells, T. gondii parasites or Quil-A. LLC cells were implanted at a density of 1×105 viable cells into the femoral muscle and Quil-A (20 µg per mouse) was injected intraperitoneally twice weekly for three consecutive weeks. Mice were orally infected with five cysts of the T. gondii Me49 strain. The extent of tumor growth was measured weekly using sterile metric calipers. Tumor volume was calculated using the following formula (13): tumor volume (µL)=tumor width (mm)2×tumor length (mm)×0.5.
The lung and muscle samples from each mouse were removed immediately after anesthesia and placed in 10% buffered neutral formaldehyde (Polyscience Inc., Warrington, PA, U.S.A.). Paraffin-embedded tissues were cut and stained with hematoxylin and eosin (H-E), and the cancer cells were examined using a bright-field microscope.
Serum samples were obtained from each mouse, and the IgG subclasses were quantified. TLA was prepared according to the protocol outlined by Lee et al. (15). Each well of a 96-well plate was coated with TLA (10 µg/mL) and incubated overnight at 4℃. After blocking, serum samples were diluted 1:100 in 0.1% bovine serum albumin/phosphate-buffered saline (BSA/PBS) that contained 0.05% Tween-20, and 100 µL of sample was added to each well. The plates were incubated for 2 hr, and HRP-conjugated goat anti-mouse IgG1 or IgG2a (Southern Biotechnology Associates Inc., Birmingham, AL, U.S.A.) was added. Following the final washing step, freshly prepared O-phenylenediamine dihydrochloride was added, and the reaction was stopped by the addition of 4 N H2SO4. The optical density was read at 492 nm using an automated ELISA reader (Tecan A-5082, Salzburg, Austria).
Following removal, the spleens were homogenized and erythrocytes were lysed in Tris-NH4Cl (pH 7.2). Splenocyte extracts that contained 1×106 cells were incubated with 50 µL of fluorescein isothiocyanate (FITC)-conjugated anti-mouse CD4+ and CD8+ monoclonal antibodies or an isotype-specific control (1:100 dilution in 0.1% BSA/PBS; PharMingen, San Diego, CA, U.S.A.) for 1 hr at 4℃. The cells were washed three times in 0.1% BSA/PBS with centrifugation, and then fixed with 1% paraformaldehyde and analyzed by flow cytometry (FACScan; Becton Dickinson, Franklin Lakes, NJ, U.S.A.). All data were analyzed using the Cell-Quest program (Becton Dickinson).
Splenic cytokine mRNA expression was assessed according to the methods of Lee et al. (15). Each sample was processed for total mRNA isolation using the RNAgent kit (Promega, Madison, WI, U.S.A.). The cDNA was prepared using a starting mixture that contained 5 µg of total RNA, 8 µL of 5×RT buffer, 4 µL of dNTPs, 25 µM oligo (dT), and 1 µL of avian myeloblastosis virus (AMV) reverse transcriptase. PCR was performed using 2-13 µL of the cDNA reaction mixture that contained 10×polymerase buffer, 250 µM dNTPs, 0.4 µM of the 3'-primer and 5'-primer, and 2.5 U Taq polymerase. Each PCR cycle consisted of 94℃ for 1 min, 57℃ for 1 min, and 72℃ for 1 min. The cytokine primer sequences were as follows: for hypoxanthine phosporibosyltransferase (HPRT), 3'-GAGGGTAGGCTGGCCTATGGCT-5' and 5'-TTGGATACAGGCCAGACTTTGTTG-3'; for interferon gamma (IFN-γ), 3'-CTCATGGAATGCATCCTTTTTCG-5' and 5'-ACGCTACACAC-TGCATCTTGG-3'; and for interleukin-10 (IL-10), 3'-TGTCTAGGTCCTGGAGTCCAGCAGACTCAA-5' and 5'-CCAGTTTTACCTGGTAGAAGTGATG-3'. After 35 PCR cycles, the products were separated by electrophoresis on 2% agarose gels. Quantification of mRNA was performed with an imaging densitometer (Bio-Rad, Hercules, CA, U.S.A.).
One week after T. gondii (or cancer cell) injection, splenic lymphocytes were isolated from each group and cultured in RPMI 1640 for three days. The splenocytes were then co-cultured with irradiated LLC cells at various effector to target (E:T) ratios for seven days. One µCi of 51Cr (NEN Research Products, Boston, MA, U.S.A.) was added to each well and the plate was allowed to incubate for 3 hr. The plate was then washed several times with RPMI 1640 until the culture supernatant contained <500 counts per minute (CPM) of 51Cr. The plates were incubated for an additional 4 hr at 37℃, followed by removal of 100 µL of each sample, and the CPM were determined by a gamma counter (Beckman, Fullerton, CA, U.S.A.). The percent lysis was calculated as follows: percent lysis=(mean CPM of test sample-mean CPM of spontaneous release)/(mean CPM of maximal release-mean CPM of spontaneous release)×100.
Matrigel angiogenesis assay was performed as described by Passaniti et al. (16). One week after T. gondii (or cancer cell) injection, the C57BL/6 mice were injected subcutaneously near the abdominal mid-line using a 25G needle with 0.5 mL Matrigel (BD Bioscience, Bedford, MA, U.S.A.) that contained 300 ng of basic fibroblast growth factor (bFGF; Sigma Chemical Co., St. Louis, MO, U.S.A.) and 32 U/mL heparin (Sigma). The injected Matrigel rapidly formed a single, solid gel and the mice were sacrificed six days later. The underlying skin of the mouse was removed, and the gels were recovered for hemoglobin (Hb) measurement using the Drabkin reagent kit (Sigma). Hemoglobin concentrations were calculated from a known Hb control, which was run in parallel.
All results are expressed as the mean±standard error (SE) for each group. Kaplan-Meier and Log-Rank tests were performed to analyze the differences in survival rates. To evaluate differences in tumor sizes, antibody titers, phenotype profiles, cytokine mRNA levels, Hb concentrations, and cytotoxicity, Kruskal-Wallis tests were used to check for statistical differences among the groups. Differences between groups were considered significant at p<0.05.
Mice were treated with T. gondii parasites, LLC cells, or Quil-A according to our protocol, and survival rates were evaluated over eight weeks. As shown in Fig. 1, the TG-, QA-, and TG/QA-injected mice survived the entire experimental period. However, the LLC- and LLC/QA-injected mice began dying 28 days after cancer cell injection, and all of these mice died after 39 days. The survival rate of the TG/LLC-injected mice was 20%, and the TG/LLC/QA-injected mice had a 30% survival rate at 8 weeks after cancer cell injection, which were significantly higher than the rates for the LLC- and LLC/QA-injected mice (p<0.05).
Mice infected with T. gondii developed brain cysts within two weeks of infection (data not shown). Cancer cells were observed in the muscle tissues within one week in the LLC- and LLC/QA-injected groups (Fig. 2A, B). However, cancer cells were found in the TG/LLC- and TG/LLC/QA-injected mice by two weeks. In addition, cancer cells were found in the lungs three weeks after LLC cell injection in the LLC- and LLC/QA-injected groups (Fig. 2C, D), whereas cancer they were found within five weeks in TG/LLC- and TG/LLC/QA-injected mice. The skin overlying the tumor began to ulcerate three to four weeks after cancer cell injection in the LLC-injected mice (Fig. 2E), whereas ulceration was observed within five weeks in the TG/LLC- and TG/LLC/QA-injected mice.
Tumor masses were palpable by two to three weeks after cancer cell injection in the cancer-bearing LLC-, LLC/QA-, TG/LLC-, and TG/LLC/QA-injected mice. In the LLC- and LLC/QA-injected mice, tumor masses increased abruptly in size at two to four weeks after cancer cell injection, and the tumor volumes at four weeks were 8,760±465 and 6,740±360 µL, respectively (Fig. 3). The tumor volumes were significantly decreased in the TG/LLC- and TG/LLC/QA-injected mice (2,155±520 µL and 1,980±342 µL, respectively) at four weeks as compared with the LLC- and LLC/QA-injected mice (p<0.05).
Serum samples were assayed for IgG subclasses against TLA by ELISA. The specific IgG1 and IgG2a titers of the LLC-, QA-, and LLC/QA-injected mice were similar to those of control mice. However, the specific IgG1 titers of the TG- and TG/QA-injected mice were significantly increased after one week of T. gondii infection (Fig. 4A). In addition, the IgG1 titers of the TG/LLC- and TG/LLC/QA-injected mice were markedly depressed following cancer cell injection as compared with the TG- and TG/QA-injected mice (p<0.05).
The IgG2a titers of the TG- and TG/QA-injected mice were significantly increased after T. gondii infection as compared to the control mice. In the TG/LLC- and TG/LLC/QA-injected mice, the IgG2a titers following cancer cell injection were significantly depressed until four weeks compared with those of the TG- and TG/QA-injected mice, although the IgG2a titers increased abruptly thereafter (Fig. 4B).
To evaluate changes in T-cell subtypes following either T. gondii infection or cancer cell injection, we analyzed the phenotypic profiles of murine splenocytes. The percentages of CD4+ T-cells in the TG-injected mice were temporarily decreased at one to two weeks post-infection compared to those of the control mice, and these levels returned to baseline shortly thereafter (Table 1). The percentages of CD4+ T-cells in the LLC-, LLC/QA-, TG/LLC-, and TG/LLC/QA-injected mice were significantly decreased following cancer cell injection and remained markedly depressed after three weeks (p<0.05).
As shown in Table 2, the percentages of CD8+ T-cells in the LLC-, LLC/QA-, TG/LLC-, and TG/LLC/QA-injected mice were significantly decreased three weeks after cancer cell injection. The LLC- and LLC/QA-injected mice maintained these low values, whereas the percentage of CD8+ T-cells in the TG/LLC- and TG/LLC/QA-injected mice recovered slightly at five weeks after T. gondii infection.
As shown in Fig. 5A, the levels of IFN-γ mRNA expression in the LLC- and LLC/QA-injected mice were increased two- to three-fold at three weeks after cancer cell injection as compared to the control (p<0.05). However, the levels of IFN-γ mRNA expression in the TG-, TG/QA-, and TG/LLC/QA-injected mice were increased immediately after T. gondii infection, peaked two to three weeks post-infection (14-19-fold increase), and slowly decreased thereafter. However, IFN-γ mRNA expression in the TG/LLC-injected mice was significantly lower than that in the TG-, TG/QA-, and TG/LLC/QA-injected mice, even though IFN-γ mRNA expression in the TG/LLC-injected mice was significantly increased by one week post-infection.
As compared to the control mice, the levels of IL-10 mRNA expression in the LLC- and LLC/QA-injected mice increased significantly, by 1.3-2.3-fold, three weeks after cancer cell injection. The levels of IL-10 mRNA expression in the TG-, TG/QA-, and TG/LLC-injected mice increased immediately following T. gondii infection, peaking at three weeks post-infection (5.5-7.5-fold increase). However, IL-10 mRNA expression in the TG/LLC/QA-injected mice, which was significantly lower than in the TG-, TG/QA-, and TG/LLC-injected mice from 3 weeks postinfection, peaked at two weeks and subsequently decreased.
An in vitro CTL assay was performed to determine whether the splenic T-cells of Toxoplasma-infected cancer-bearing mice increased cytotoxic activity against LLC cells. As shown in Fig. 6, the LLC-, QA-, and LLC/QA-injected mice did not show CTL responses. However, the splenocyte CTL activities of the TG- and TG/QA-injected mice increased as the E:T ratio increased. The percentages of specific lysis seen in the TG/LLC- and TG/LLC/QA-injected mice were considerably higher than those in the LLC- and LLC/QA-injected mice. However, the CTL activities of the TG/LLC- and TG/LLC/QA-injected mice were significantly lower than those of the TG- and TG/QA-injected mice (p<0.05).
The Matrigel plugs that formed following subcutaneous implantation of Matrigel alone produced little or no local reaction, nor did they produce an angiogenic response. However, the plugs that formed following the implantation of Matrigel that was supplemented with bFGF and heparin caused an angiogenic reaction (Fig. 7). We measured the Hb concentrations inside the Matrigel plugs in order to quantify the extent of angiogenesis. The Hb concentrations in the LLC-, QA-, and LLC/QA-injected mice ranged from 14.6±2.0 to 16.2±1.6 g/dL, whereas those in the TG- and TG/QA-injected mice ranged from 4.1±0.7 to 4.3±0.9 g/dL. The Hb concentrations in the TG/LLC- and TG/LLC/QA-injected mice were significantly lower than those in the LLC- or LLC/QA-injected mice (p<0.05), but were not significantly different from those in the TG- or TG/QA-injected mice.
Tumors frequently interfere with the development and function of immune responses (3-5). The ability of various infections to suppress neoplastic growth has been well documented. In addition, T. gondii is a nonspecific immune stimulant used for cancer immunotherapy (7-11). In the present study, we examined the mechanisms of the antitumor effects of T. gondii infection on lung cancer in vivo. Toxoplasma infection of LLC-bearing mice significantly increased the survival rates, serum IgG2a titers, IFN-γ mRNA expression, CD8+ T-cell percentages, CTL responses, and also inhibited angiogenesis. These values were similar or further improved by the addition of an adjuvant, Quil-A. These results indicate that T. gondii activates Th1 immune responses and inhibits angiogenesis in LLC-bearing mice, leading to the induction of antitumor and antimetastatic activities.
Cancer immunotherapy attempts to harness the exquisite power and specificity of the immune system for the treatment of malignancy. Although cancer cells are less immunogenic than pathogens, the immune system is clearly capable of recognizing and eliminating tumor cells. Lung cancer immunotherapy may represent one new approach that has low toxicity and high specificity, but implementation has been a challenge due to poor antigenic characterization and the ability of lung cancers to escape immune responses (3-5, 17). Several different immunotherapeutic treatment strategies and mouse models have been developed. In this study, we utilized LLC cells, which remain highly tumorigenic in C57BL/6 mice and produce primary tumors and lung metastases histologically indistinguishable from the original tumor line (18). In the LLC- and LLC/QA-injected mice, tumor masses were initially palpable in the muscle tissues located at the site of cancer cell injection from two weeks after cancer cell injection, and cancer cells were found in the lungs three weeks after LLC cell injection. Thus, it was confirmed that the LLC cell line is useful for studying the mechanisms of cancer chemotherapeutic agents. After T. gondii infection, tumor volumes were significantly decreased in the TG/LLC- and TG/LLC/QA-injected mice as compared with the LLC- and LLC/QA-injected mice. However, the mechanisms of antitumor activity induced by T. gondii infection are not easily introduced in LLC, so we suggested the mechanisms of antitumor effects in this study.
Infection with T. gondii results in a strong cell-mediated immune response, especially the T-cell-mediated response (19). In the present study, the percentages of CD8+ and CD4+ T-cells were significantly decreased in cancer-bearing LLC- and LLC/QA-injected mice. Following infection with T. gondii, the percentages of CD8+ T-cells in TG/LLC- or TG/LLC/QA-injected mice were increased from 5 weeks post-infection, but those of CD4+ T-cells were not. Collectively, these data indicate that T-cell-mediated immune responses were severely suppressed in Lewis lung carcinoma, and T. gondii alone or in combination with Quil-A may provide an appropriate epitope for MHC-1 presentation (17, 19), and therefore, the percentages of CD8+ T-cells were recovered. This phenomenon is confirmed by the results of a cytotoxic T lymphocyte assay. Namely, Toxoplasma-infected TG/LLC- and TG/LLC/QA-injected mice demonstrated higher CTL responses of CD8+ T-cells than the cancer-bearing LLC- or LLC/QA-injected mice. Previous studies have also demonstrated that strong antitumor responses are induced by either abundant infiltration of both CD4+ and CD8+ T-cells and tumor-specific CTLs in LLC-bearing mice after treatment with IL-2 and IL-12 (17) or increases in the numbers of apoptotic tumor cells, CD8+ T-cells, and natural killer (NK) cells that invade the tumors after injection with ergosterol (14).
Cytokines serve as critical regulators of cell-mediated immunity by both enhancing and limiting immune responses (20). The CD4+ T-cells are divided into two classes on the basis of the range of cytokines produced. Th1 cells produce IL-2 and IFN-γ, and control the production of IgG2a, whereas Th2 cells produce IL-4, IL-5, and IL-10, and control the production of IgG1 and IgE. Because of these interleukin secretion patterns, Th1 cells promote cell-mediated immunity. In contrast, Th2 cells induce B-cell differentiation and inhibit cell-mediated immunity and antitumor responses. In the present study, we found that IFN-γ mRNA expression in TG/LLC-injected mice was significantly higher than that in LLC-injected mice and significantly lower than that in TG-injected mice. These results indicate that Th1 immune responses were depressed in Lewis lung carcinoma, and show that the production of cytokines such as IFN-γ, TNF-α, IL-12, and IL-2 can arrest proliferation of malignant cells and prevent the angiogenesis necessary for tumor growth (3, 5, 17, 21, 22). Similar results were reported in melanoma, liver cancer, and thyroid cancer (22-24). And these phenomena were also confirmed in the titrations of the IgG subclasses. The IgG2a titer, which is the primary indicator of a Th1 immune response (25), was significantly increased in the TG/LLC- and TG/LLC/QA-injected mice four weeks after infection, while the IgG1 titer, an indicator of the Th2 immune response, remained unchanged.
The development of a vascular supply is essential for the growth, maturation, and maintenance of normal tissues. It is also required for wound healing and rapid growth of solid tumors, and is involved in various other pathologic conditions. Tumor angiogenesis is regulated by the production of angiogenic stimulators, including members of the fibroblast growth factor and the vascular endothelial growth factor families (13, 14, 26, 27). In the present study, a combination of bFGF and heparin recruited vessels from the surrounding tissues into the Matrigel. The Hb concentrations in T. gondii-infected TG-, TG/QA-, TG/LLC-, and TG/LLC/QA-injected mice were significantly decreased by three- to four-fold as compared to uninfected QA-, LLC-, and LLC/QA-injected mice. In a similar study, Hunter et al. (28) showed that T. gondii infection is accompanied by strong systemic suppression of angiogenesis. This suppression results in severe hypoxia and avascular necrosis, which are incompatible with progressive neoplastic growth. Beside this, heyneanol A obtained from an Oriental medicinal herb, sodium pyroglutamate isolated from Agaricus blazei, and glycosaminoglycan isolated from the African giant snail were also reported as potent antitumor and anti-angiogenic substances in LLC-bearing mice (13, 14, 26, 27).
The antitumor activity of cancer immunotherapy has been explored using advanced molecular and immunological methods. Host immunity is likely related to various factors, including the route of challenge, mouse strain, antigen preparation, and adjuvant (29). Our present results suggest that Toxoplasma infection in LLC-bearing mice induces antitumor activity against lung cancer through the induction of a Th1-type immune response and antiangiogenic activity. These effects were similar or further increased by the modulation of Quil-A. Quil-A, a saponin derived from the bark of Quillaia saponaria Molina, induces antigen presentation, cytokine production, and T-lymphocyte cytotoxicity (29, 30). However, the molecular mechanisms underlying Quil-A activity have not yet been completely described. Infection with T. gondii leads to the rapid induction of a strong cell-mediated immune response in the experimental murine host. These facts suggest the possibility that new immunostimulating molecules could be found in Toxoplasma organisms.
Figures and Tables
Fig. 1
Survival rates of C57BL/6 mice injected with Toxoplasma gondii, Lewis lung carcinoma (LLC) cells or Quil-A. Mice were checked daily for eight weeks following cancer cell injection, to measure survival rates. The groups (n=10 per group) were as follows: LLC, injected in the femoral muscle with 1×105 viable LLC cells; TG, infected with five cysts of the Me49 strain of T. gondii; TG/LLC, received both Toxoplasma parasites and LLC cells; QA, received 20 µg/mouse of Quil-A twice weekly for 3 weeks; LLC/QA, received both LLC cells and Quil-A; TG/QA, received both T. gondii and Quil-A; TG/LLC/QA, received T. gondii, LLC cells, and Quil-A.
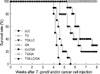
Fig. 2
Histopathologic findings for the muscles and lungs of C57-BL/6 mice injected with T. gondii, LLC cells or Quil-A. Paraffin-embedded tissues were stained with hematoxylin and eosin, and visualized under a microscope at ×400 magnification. (A) Normal muscle; (B) cancerous changes in the muscle at cancer cell-injected sites; (C) normal lung; (D) cancerous changes in the lung (cancer metastasis to the lungs); (E) tumor masses of mice injected with LLC cells (left and middle) and injected with both T. gondii and LLC cells (right). Ulceration was observed in cancer-bearing mice (arrow).
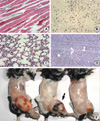
Fig. 3
Sizes of tumors in C57BL/6 mice injected with T. gondii, LLC cells or Quil-A. Tumor growth was measured weekly using sterile metric calipers. Tumor volume (µL)=tumor width (mm)2×tumor length (mm)×0.5. Data are presented as the means±SE of five mice (*, p<0.05 compared with LLC- or LLC/QA-injected group).
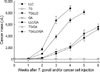
Fig. 4
Serum IgG1 (A) and IgG2a (B) titers of C57BL/6 mice injected with T. gondii, LLC cells or Quil-A, as measured by ELISA. Serum samples were obtained from mice at the indicated time-points, and the IgG subclasses against Toxoplasma lysate antigen were quantified. Data are presented as the mean±SE of five mice (*, p<0.05 compared with LLC- or LLC/QA-injected group; †, p<0.05 compared with TG- or TG/QA-injected group).
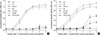
Fig. 5
Relative IFN-γ (A) and IL-10 (B) mRNA expression in splenocytes from C57BL/6 mice injected with T. gondii, LLC cells or Quil-A. The transcript levels are relative to those in splenocytes obtained from control mice (designated value of 1). Splenocytes were harvested at the time-points indicated and the expression of IFN-γ and IL-10 mRNAs was assayed by RT-PCR. Data are presented as mean±SE of five mice.
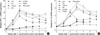
Fig. 6
Cytotoxic T-cell responses of C57BL/6 mice injected with T. gondii, LLC cells or Quil-A. One week after T. gondii (or cancer cell) injection, splenic lymphocytes were isolated from each mouse, labeled with 51Cr, and cultured with irradiated LLC cells at different cell ratios. Data are presented as mean±SE of five mice.
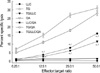
Fig. 7
Angiogenesis in C57BL/6 mice injected with T. gondii, LLC cells or Quil-A. One week after T. gondii (or cancer cell) injection, a Matrigel that was supplemented with heparin and basic fibroblast growth factor was injected into the mice. The gels were recovered on day 6 postinjection for hemoglobin measurement using the Drabkin reagent kit. Data are presented as the mean±SE of five mice. (A) Hemoglobin content of each group; (B) Matrigel plugs were removed 6 days after injection and photographed (arrows): LLC-bearing mice (left), Toxoplasma-infected mice (middle), and Matrigel alone (right).
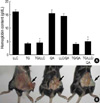
Table 1
Percentages of CD4+ T cell subsets in splenocytes from mice injected with T. gondii, Lewis lung carcinoma cells, or Quil-A
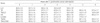
ACKNOWLEDGMENT
This study was financially supported by Research Institute for Medical Science, Chungnam National University in 2006.
References
1. Jemal A, Siegel R, Ward E, Murray T, Xu J, Smigal C, Thun MJ. Cancer statistics, 2006. CA Cancer J Clin. 2006. 56:106–130.


2. Collins LG, Haines C, Perkel R, Enck RE. Lung cancer: diagnosis and management. Am Fam Physician. 2007. 75:56–63.
4. Berzofsky JA, Terabe M, Oh S, Belyakov IM, Ahlers JD, Janik JE, Morris JC. Progress on new vaccine strategies for the immunotherapy and prevention of cancer. J Clin Invest. 2004. 113:1515–1525.


5. Blattman JN, Greenberg PD. Cancer immunotherapy: a treatment for the masses. Science. 2004. 305:200–205.


7. Hibbs JB Jr, Lambert LH Jr, Remington JS. Resistance to murine tumors conferred by chronic infection with intracellular protozoa, Toxoplasma gondii and Besnoitia jellisoni. J Infect Dis. 1971. 124:587–592.


8. Miyahara K, Yokoo N, Sakurai H, Igarashi I, Sakata Y, Yoshida Y, Saito A, Hirose T, Suzuki N. Antitumor activity of Toxoplasma lysate antigen against methylcholanthrene-induced tumor-bearing rats. J Vet Med Sci. 1992. 54:221–228.


9. Varga A, Sokolowska-Kohler W, Presber W, Von Baehr V, Von Baehr R, Lucius R, Volk D, Nacsa J, Hever A. Toxoplasma infection and cell free extract of the parasites are able to reverse multidrug resistance of mouse lymphoma and human gastric cancer in vitro. Anticancer Res. 1999. 19:1317–1324.
10. Suzuki Y, Kobayashi A. Antitumor effect of intralesional injection with formalin-fixed Toxoplasma gondii organisms on Lewis lung carcinoma in Toxoplasma-infected mice. Cancer Lett. 1985. 25:247–254.


11. Suzuki Y, Muto M, Kobayashi A. Antitumor effect of formalin-fixed Toxoplasma gondii organisms on EL4 lymphoma in Toxoplasma-infected mice. J Biol Response Mod. 1986. 5:288–293.
12. Lee HJ, Lee HJ, Song GY, Li G, Lee JH, Lü J, Kim SH. 6-(1-Oxobutyl)-5,8-dimethoxy-1,4-naphthoquinone inhibits lewis lung cancer by antiangiogenesis and apoptosis. Int J Cancer. 2007. 120:2481–2490.


13. Lee EO, Lee HJ, Hwang HS, Ahn KS, Chae C, Kang KS, Lu J, Kim SH. Potent inhibition of Lewis lung cancer growth by heyneanol A from the roots of Vitis amurensis through apoptotic and anti-angiogenic activities. Carcinogenesis. 2006. 27:2059–2069.


14. Kimura Y, Kido T, Takaku T, Sumiyoshi M, Baba K. Isolation of an anti-angiogenic substance from Agaricus blazei Murill: its antitumor and antimetastatic actions. Cancer Sci. 2004. 95:758–764.


15. Lee YH, Kasper LH. Immune responses of different mouse strains after challenge with equivalent lethal doses of Toxoplasma gondii. Parasite. 2004. 11:89–97.
16. Passaniti A, Taylor RM, Pili R, Guo Y, Long PV, Haney JA, Pauly RR, Grant DS, Martin GR. A simple, quantitative method for assessing angiogenesis and antiangiogenic agents using reconstituted basement membrane, heparin, and fibroblast growth factor. Lab Invest. 1992. 67:519–528.
17. Tanaka M, Saijo Y, Sato G, Suzuki T, Tazawa R, Satoh K, Nukiwa T. Induction of antitumor immunity by combined immunogene therapy using IL-2 and IL-12 in low antigenic Lewis lung carcinoma. Cancer Gene Ther. 2000. 7:1481–1490.


18. Bertram JS, Janik P. Establishment of a cloned line of Lewis Lung Carcinoma cells adapted to cell culture. Cancer Lett. 1980. 11:63–73.


19. Denkers EY. T lymphocyte-dependent effector mechanisms of immunity to Toxoplasma gondii. Microbes Infect. 1999. 1:699–708.


20. Male DK, Brostoff J, Roth DB, Roitt I. Immunology. 2006. 7th ed. Canada: Mosby Elsevier.
21. Sharma S, Stolina M, Luo J, Strieter RM, Burdick M, Zhu LX, Batra RK, Dubinett SM. Secondary lymphoid tissue chemokine mediates T cell-dependent antitumor responses in vivo. J Immunol. 2000. 164:4558–4563.


22. Dietrich A, Becherer L, Brinckmann U, Hauss J, Liebert UG, Gütz A, Aust G. Particle-mediated cytokine gene therapy leads to antitumor and antimetastatic effects in mouse carcinoma models. Cancer Biother Radiopharm. 2006. 21:333–341.


23. Komita H, Homma S, Saotome H, Zeniya M, Ohno T, Toda G. Interferon-gamma produced by interleukin-12-activated tumor infiltrating CD8+T cells directly induces apoptosis of mouse hepatocellular carcinoma. J Hepatol. 2006. 45:662–672.


24. Choi Y, Jeon YH, Kang JH, Chung JK, Schmidt M, Kim AC. MIDGE/hNIS vaccination generates antigen-associated CD8+IFN-gamma+ T cells and enhances protective antitumor immunity. Int J Cancer. 2007. 120:1942–1950.
25. Nguyen TD, Bigaignon G, Van Broeck J, Vercammen M, Nguyen TN, Delmee M, Turneer M, Wolf SF, Coutelier JP. Acute and chronic phases of Toxoplasma gondii infection in mice modulate the host immune responses. Infect Immun. 1998. 66:2991–2995.


26. Ramirez BS, Pestana ES, Hidalgo GG, Garcia TH, Rodriguez RP, Ullrich A, Fernandez LE. Active antimetastatic immunotherapy in Lewis lung carcinoma with self EGFR extracellular domain protein in VSSP adjuvant. Int J Cancer. 2006. 119:2190–2199.
27. Lee YS, Yang HO, Shin KH, Choi HS, Jung SH, Kim YM, Oh DK, Linhardt RJ, Kim YS. Suppression of tumor growth by a new glycosaminoglycan isolated from the African giant snail Achatina fulica. Eur J Pharmacol. 2003. 465:191–198.


28. Hunter CA, Yu D, Gee M, Ngo CV, Sevignani C, Goldschmidt M, Golovkina TV, Evans S, Lee WF, Thomas-Tikhonenko A. Cutting edge: systemic inhibition of angiogenesis underlies resistance to tumors during acute toxoplasmosis. J Immunol. 2001. 166:5878–5881.


29. Dumont AR, Kalfayan LH, Sekaly RP. Modulation of immune responses-strategies for optimising vaccines. Expert Opin Biol Ther. 2004. 4:627–630.