Abstract
The aim of this study was to investigate antimicrobial susceptibilities and macrolide resistance mechanisms of β-hemolytic viridans group streptococci (VGS) in a tertiary Korean hospital. Minimum inhibitory concentrations (MICs) of seven antimicrobials were determined for 103 β-hemolytic VGS isolated from various specimens. The macrolide resistance mechanisms of erythromycin-resistant isolates were studied by the double disk test and polymerase chain reaction (PCR). The overall resistance rates of β-hemolytic VGS were found to be 47.5% to tetracycline, 3.9% to chloramphenicol, 9.7% to erythromycin, and 6.8% to clindamycin, whereas all isolates were susceptible to penicillin G, ceftriaxone, and vancomycin. Among ten erythromycin-resistant isolates, six isolates expressed a constitutive MLSB (cMLSB) phenotype, and each of the two isolates expressed the M phenotype, and the inducible MLSB (iMLSB) phenotype. The resistance rates to erythromycin and clindamycin of β-hemolytic VGS seemed to be lower than those of non-β-hemolytic VGS in our hospital, although cMLSB phenotype carrying erm(B) was dominant in β-hemolytic VGS.
Beta-hemolytic streptococcal isolates from humans can be subdivided into large-colony and small-colony (<0.5 mm in diameter) formers (1). The small colony-forming β-hemolytic strains with Lancefield group A, C, F, or G antigens belong to the anginosus or previously termed 'Streptococcus milleri' species group. Members of the anginosus group (Streptococcus anginosus, Streptococcus constellatus, and Streptococcus intermedius) are considered part of the viridans group streptococci (VGS), the majority of which display α-hemolytic or non-hemolytic reactions. The VGS also include Streptococcus mitis, Streptococcus oralis, Streptococcus mutans, Streptococcus salivarius, Streptococcus sanguis, and Streptococcus bovis (1-3). Although small-colony-forming β-hemolytic VGS are found as commensals whose pathogenic abilities appear to be much more subtle than those of the pyogenic streptococci, they may also participate in various infections such as subacute bacterial endocarditis, catheter-related and neutropenia-related bloodstream infections, purulent abdominal, hepatobiliary, brain, and dental infections (3-5). In addition, they play a significant role as a reservoir of antimicrobial resistance genes, transferring different resistance traits to more pathogenic organisms such as Streptococcus pneumoniae and Streptococcus pyogenes (6).
Beta-lactam agents are the treatment of choice for VGS infections, but increasing resistance to penicillin has recently been reported in many parts of the world (7). Macrolides and related drugs have been suggested as alternatives, but recent studies have shown that macrolide resistance may also be a problem (7). Moreover, Clinical and Laboratory Standards Institute (CLSI) recommended that VGS isolated from normally sterile body sites should be tested for penicillin susceptibility using the minimum inhibitory concentration (MIC) method, and interpretive criteria for the VGS should be used (2). However, laboratory personnel have difficulty in differentiating β-hemolytic VGS from pyogenic streptococci based on colony size. Although many recent studies have elucidated susceptibilities of VGS isolated from clinical samples (mainly from blood cultures) to various anti- microbial agents, such studies on β-hemolytic VGS from the various specimens are scarce.
The objectives of the present study were to determine the incidence and patterns of antimicrobial resistance among β-hemolytic VGS isolated from various clinical specimens in a Korean hospital and to clarify the macrolide resistance phenotypes and genotypes of erythromycin-resistant isolates.
One hundred and three isolates of β-hemolytic VGS isolated from various clinical specimens were collected between January 2003 and December 2004 at Wonju Christian Hospital in South Korea. Isolates were identified by standard methods: Streptex latex agglutination assay (Murex Biotech Limited, Dartford, England) and the API Rapid ID32 STREP system (bioMérieux, Marcy-l'Etoile, France). These were stored in thioglycollate broth plus 20% glycerol at -70℃ until studied.
Susceptibility to penicillin G, erythromycin, clindamycin, ceftriaxone, chloramphenicol, tetracycline (Sigma Chemical Co, St. Louis, MO, U.S.A.), and vancomycin (Daewoong Lilly, Korea) was determined by an agar dilution method (2), and MICs were interpreted using the CLSI criteria (2). Macrolide resistance phenotypes of erythromycin-resistant isolates were determined using a double-disk test with erythromycin (15 µg) and clindamycin (2 µg) disks on Mueller-Hinton agar plates containing 5% sheep blood. Genomic DNA was extracted with an Easy-DNA kit (Invitrogen, Carlsbad, CA, U.S.A.), according to the manufacturer's instructions. The presence of erm and mef class genes was determined by PCR amplification using previously described primers specific for erm(A), erm(B), erm(C), erm(TR), and erm(A) (8). To confirm that PCR reactions were amplifying the target sequences for specific primers, PCR products were sequenced (Macrogen Inc., Seoul, Korea).
Of the 103 β-hemolytic VGS, nine (8.7%), forty (38.8%), and fifty-three (51.5%) isolates were agglutinated with group C, F, and G antisera, respectively. One isolate of β-hemolytic S. intermedius was nongroupable. Using the agar dilution method, the overall resistance (intermediate and resistance) rates of β-hemolytic VGS were measured at 47.5% to tetracycline, 3.9% to chloramphenicol, 9.7% to erythromycin and 6.8% to clindamycin, whereas all isolates were susceptible to penicillin G, ceftriaxone, and vancomycin. One S. intermedius isolated from peritoneal fluid was susceptible to tetracycline, erythromycin, and clindamycin. Resistance rates to tetracycline, erythromycin, and clindamycin of group F and G isolates were 37.5% vs. 52.8%, 17.5% vs. 5.7%, and 15.0% vs. 3.8%, respectively. All of group C β-hemolytic VGS isolates were susceptible to erythromycin, clindamycin, and chloramphenicol, although tetracycline resistance rate (66.7%) of group C was higher than other serogroups (Table 1).
Among the β-hemolytic VGS, 68 (66.0%) and 25 (24.3%) were identified as S. anginosus and S. constellatus, respectively. The remaining isolates included Gemella haemolysans (six isolates), S. intermedius (one isolate), S. oralis (one isolate), S. salivarius (one isolate), and Streptococcus alactolyticus (one isolate). The serogroup proportions of S. anginosus were G (63.2%), F (33.8%), and C (2.9%), in decreasing order, whereas those of S. constellatus were F (44.0%), G (36.0%), and C (20.0%). Among the S. anginosus isolates, group G was frequently isolated from the lower respiratory tract specimens such as bronchial washing and sputum (55.8%), and wound cultures (37.2%), whereas group F was somewhat prevalent in genitourinary tract specimens (52.2%). As contrasted with S. anginosus, the specimen distribution of S. constellatus by serogroups had little or no difference. Of the nine erythromycin-resistant S. anginosus strains, six and three were group F and G, respectively. Among ten erythromycin-resistant isolates, six isolates expressed a constitutive MLSB (cMLSB) phenotype, and each of the two isolates expressed an M phenotype and an inducible MLSB (iMLSB) phenotype, respectively. All cMLSB and M phenotypes carried the erm(B) and mef(A) genes, respectively. Of the two iMLSB phenotypes, each one carried erm(B) and erm(TR) gene, respectively. One isolate with the cMLSB phenotype carried both of erm(B) and erm(TR) genes (Table 2).
Although most strains of S. anginosus group have been known to be non-hemolytic or β-hemolytic, it is estimated that 25% are β-hemolytic (9). Lawrence et al. (10) reported that a majority (84%) of the S. milleri strains possessed Lancefield group antigen (3 A, 27 C, 41 F, and 5 G), whereas 16% were nongroupable. In this study, however, group G was the most commonly isolated serogroup among β hemolytic VGS, and only one strain was nongroupable. Many authors (1, 3) have reported that: S. anginosus strains may possess Lancefield group A, C, F, or G antigens; S. constellatus strains are more β-hemolytic than S. anginosus or S. intermedius, and possess mainly Lancefield group F antigens; and that isolates of S. intermedius strains are rarely β-hemolytic. Some authors (1, 5) have documented that there tends to be an association between the clinical specimen sources and three species of anginosus group organisms. In addition, β-hemolytic group C strains of S. constellatus constitute two subspecies: S. constellatus subsp. constellatus, which is isolated from a relatively broad clinical background, and S. constellatus subsp. pharyngis, which shows a close association with the human throat and pharyngitis. S. anginosus isolates are commonly isolated from urogenital and gastrointestinal sources, and strains of S. constellatus have been isolated relatively frequently from the respiratory tract and many other sources, while S. intermedius strains are often isolated among the polymicrobial flora of deep-seated abscesses, notably in the liver and brain. Our results are also consistent with above findings.
Identification to the species level among β-hemolytic VGS should not be ignored because β-hemolytic VGS differ from large-colony-forming β-hemolytic streptococci in clinical significance and interpretive criteria of susceptibility. Additionally, anginosus group organisms recovered from abscess or wound specimens, even when other organisms are present, are likely to be pathogens rather than contaminants. Careful evaluation of the significance of β-hemolytic VGS according to specimen types is needed, and a clinical laboratory setting for the precise identification of β-hemolytic VGS should be a priority.
Until the 1980s, VGS were considered uniformly susceptible to β-lactam antibiotics, but resistance had spread rapidly in the 1990s. VGS that are not susceptible to penicillin also showed reduced susceptibility to ceftriaxone, erythromycin, and clindamycin. The resistance rates to penicillin, clindamycin, erythromycin, and ceftriaxone of VGS isolated from blood cultures in our previous study (8) were 57.6%, 33.9%, 17.9%, and 9%, respectively. In this study, however, any of β-hemolytic VGS isolates did not show resistance to either penicillin or to ceftriaxone. In our previous study, the overall resistance rates of β-hemolytic streptococci were found to be 80.0% to tetracycline, 22.8% to chloramphenicol, 20.2% to erythromycin and 19.1% to clindamycin, whereas all isolates were susceptible to penicillin G, ceftriaxone and vancomycin (11). These results suggested that susceptibilities of β-hemolytic VGS to β-lactams are similar to pyogenic β-hemolytic streptococci rather than non-β-hemolytic VGS. The rate of clindamycin resistance in VGS has been determined mainly by the distribution of MLSB resistance phenotypes. In this study, all of erythromycin-susceptible isolates were susceptible to clindamycin, and the cMLSB phenotype was the most frequently observed mechanism among erythromycin-resistant isolates. The proportion of the M phenotype and iMLSB was equal. However, the numbers of erythromycin-resistant strains were too small to draw a conclusion. As for the non-β-hemolytic VGS, the distribution of MLSB phenotypes of β-hemolytic VGS may also vary with many factors such as geography, specimen sources, species identified, and so on (8, 12).
We think that susceptibility testing of β-lactams for treatment of β-hemolytic VGS is not necessary for clinical purpose, and may not be done routinely in the hospitals, because, as with vancomycin, resistant strains have not been detected in this study. The resistance rates to erythromycin and clindamycin of β-hemolytic VGS seemed to be relatively lower than non-β-hemolytic VGS in our hospital, although cMLSB phenotype-carrying erm(B) was dominant in β-hemolytic VGS. Further epidemiologic studies are needed to confirm whether or not our susceptibility data on β-hemolytic VGS are restricted to our area.
Figures and Tables
Table 2
Distribution of erythromycin-resistant β-hemolytic viridans group streptococci according to specimen type and serogroup
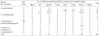
*cMLSB phenotype with erm(B) gene; †cMLSB phenotype with erm(B) gene (1), iMLSB phenotype with erm(TR) gene (1), and iMLSB phenotype with erm(B) gene (1); ‡cMLSB phenotype with erm(B) and erm(TR) gene; §M phenotype with mef(A) gene; ∥Peritoneal fluid (1), suction tip (3), bile (1), gall bladder (1), gastric washing (1). BW, bronchial washing; NG, nongroupable.
References
1. Ruoff KL, Whiley RA, Beighton D. Murray PR, Baron EJ, Jorgensen JH, Pfaller MA, Yolken RH, editors. Streptococcus. Manual of clinical microbiology. 2003. 8th ed. American Society for Microbiology, Washington, D.C: ASM.
2. Clinical and Laboratory Standards Institute. CLSI document M100-S16. Performance Standards for Antimicrobial Susceptibility Testing; Sixteenth Informational Supplement. 2006. Wayne, PA: CLSI.
3. Facklam R. What happened to the streptococci: overview of taxonomic and nomenclature changes. Clin Microbiol Rev. 2002. 15:613–630.


4. Bert F, Bariou-Lancelin M, Lambert-Zechovsky N. Clinical significance of bacteremia involving the "Streptococcus milleri" group: 51 cases and review. Clin Infect Dis. 1998. 27:385–387.


5. Clarridge JE 3rd, Osting C, Jalali M, Osborne J, Waddington M. Genotypic and phenotypic characterization of "Streptococcus milleri" group isolates from a Veterans Administration hospital population. J Clin Microbiol. 1999. 37:3681–3687.


6. Bryskier A. Viridans group streptococci: a reservoir of resistant bacteria in oral cavities. Clin Microbiol Infect. 2002. 8:65–69.


7. Diekema DJ, Beach ML, Pfaller MA, Jones RN. Antimicrobial resistance in viridans group streptococci among patients with and without the diagnosis of cancer in the USA, Canada and Latin America. Clin Microbiol Infect. 2001. 7:152–157.


8. Uh Y, Shin DH, Jang IH, Hwang GY, Lee MK, Yoon KJ, Kim HY. Antimicrobial susceptibility patterns and macrolide resistance genes of viridans group streptococci from blood cultures in Korea. J Antimicrob Chemother. 2004. 53:1095–1097.


9. Ball LC, Parker MT. The cultural and biochemical characters of Streptococcus milleri strains isolated from human sources. J Hyg (Lond). 1979. 82:63–78.
10. Lawrence J, Yajko DM, Hadley WK. Incidence and characterization of beta-hemolytic Streptococcus milleri and differentiation from S. pyogenes (group A), S. equisimilis (group C), and large-colony group G streptococci. J Clin Microbiol. 1985. 22:772–777.

