Abstract
As the incidence of nontuberculous mycobacterial infection has been increasing recently in Korea, the importance of drug susceptibility test for clinical isolates of mycobacteria has become larger. In this study we determined the antimicrobial susceptibility patterns of clinical isolates of M. fortuitum and M. abscessus in Korea, and evaluated the efficacy of a modified broth microdilution method using 2,3-diphenyl-5-thienyl-(2)-tetrazolium chloride (STC), in terms of its ability to provide accurate and easy-to-read minimal inhibitory concentration (MIC) endpoints for the susceptibility testing of rapidly growing mycobacteria. Most isolates of M. fortuitum and M. abscessus in Korea are susceptible or intermediately susceptible to amikacin, cefoxitin, ciprofloxacin, and clarithromycin. Many isolates of M. fortuitum are susceptible to doxycycline, sulfamethoxazole, and imipenem, while many M. abscessus isolates are resistant to these drugs. In the present study, the modified broth microdilution method using STC was found to be reliable, easy to read, and inexpensive for M. fortuitum and M. abscessus susceptibility testing. The modified colorimetric MIC testing method using STC was proven to be a useful surrogate for RGM antibiotic susceptibility testing.
Nontuberculous mycobacteria (NTM) are important environmental pathogens that cause a broad spectrum of diseases (1). NTM infections have been reported worldwide with varying frequencies (2-4), while the isolation rates and the incidences of diseases caused by NTM are increasing in Korea (5-8). In a nationwide survey from 1981 to 1994, the incidences of Mycobacterium avium-intracellulare complex (MAC), M. fortuitum, and M. chelonae were 65.2%, 12.7%, and 9.5%, respectively, among all NTM infections (5). And also, another report confirmed that MAC isolates were the most frequent in clinical specimens in mid-1990s, followed by M. abscessus and M. fortuitum (8). Rapidly growing mycobacteria (RGM) such as M. fortuitum, M. abscessus, and M. chelonae cause several forms of clinical diseases of varying severity, most commonly skin and soft tissue infections, but they also cause skeletal, pulmonary, and disseminated diseases (4, 9). In particular, MAC, M. fortuitum, and M. abscessus have been reported to be associated with clinical pulmonary diseases (6, 10-12).
NTM cause infections more commonly in the presence of predisposing factors and/or underlying diseases (4, 13) and are often considered contaminants. Because these organisms are notably resistant to the antimicrobial agents commonly used to treat tuberculosis (4, 14), susceptibility testing is indicated for any RGM that is considered clinically significant (e.g., isolates from blood, tissue, skin, and soft tissue lesions) (14, 15). The statement on the diagnosis and treatment of diseases caused by NTM issued by the American Thoracic Society suggests that a minimum of seven drugs (amikacin, cefoxitin, ciprofloxacin, clarithromycin, doxycycline, imipenem, and a sulfonamide) should be tested against clinically significant RGM (14), and the Clinical and Laboratory Standards Institute (CLSI) recommends that the standard broth microdilution method be used for the susceptibility testing of M. fortuitum, M. chelonae, and M. abscessus (15). Although the broth microdilution procedure is widely used for the susceptibility testing of several antibiotics on a large number of bacterial isolates, several difficulties concerning MIC interpretation for RGM interfere with the susceptibility testing of these isolates (16, 17). Moreover, because the incidence of NTM infection is not high, only a few reference centers are able to offer the susceptibility testing of clinically significant strains as a service. Actually no comparative data on the susceptibility testing of these organisms is available in Korea. Thus, a more convenient and easy-to-interpret method of susceptibility testing seems important. As a modification of the CLSI standard method, the colorimetric or spectrophotometric MIC endpoint determination could be applied using color indicators. 2,3-diphenyl-5-thienyl-(2)-tetrazolium chloride (STC) (TCI, Tokyo, Japan) is an oxidation-reduction indicator that changes from colorless to red as the organisms grow, and has been applied in mycobacterial or fungal susceptibility testing (18-20). The purpose of this study was to determine the antimicrobial susceptibility patterns of clinical isolates of M. fortuitum and M. abscessus in Korea, and to evaluate the broth microdilution method using STC in terms of its ability to provide accurate and easy-to-read minimum inhibitory concentration (MIC) endpoint results for the antimycobacterial susceptibility testing of RGM.
The antimicrobial agents and their concentrations (in serial 2-fold dilutions) tested against RGM for MIC determinations were amikacin (0.5-64 µg/mL), cefoxitin (1-128 µg/mL), ciprofloxacin (0.03-4 µg/mL), clarithromycin (0.06-8 µg/mL), doxycycline (0.125-16 µg/mL), imipenem (0.25-32 µg/mL), and sulfamethoxazole (1-128 µg/mL). The drugs were purchased from Sigma Chemical (St. Louis, MO, U.S.A.), except clarithromycin (Abbott Laboratories, North Chicago, IL, U.S.A.) and imipenem (Choongwae, Seoul, Korea). Antibiotic stock solutions were made by dissolving powders in distilled water, methanol or phosphate buffer, and were divided into 1 mL aliquots and frozen at -70℃ until required, except imipenem, which was used immediately after preparation. On the day of testing, antibiotic stock solutions were thawed and diluted in cation-supplemented Mueller-Hinton broth (Becton Dickinson, Sparks, MD, U.S.A.).
STC was dissolved in distilled water at 5 g/L at room temperature. This solution was filtered through a 0.20 µm filter (Corning, Corning, NY, U.S.A.) and then stored at -70℃ until needed. The solubilizing agent used to dissolve reduced tetrazolium (STC) precipitates was prepared by dissolving 20% (w/v) sodium dodecyl sulfate (Bio-Rad, Hercules, CA, U.S.A.) in 50% (v/v) N,N-dimethylformamide (Sigma Chemical), and was stored unfiltered at room temperature.
Fifty-five M. fortuitum and 48 M. abscessus strains isolated from clinical specimens were collected from Ulsan University Hospital (UUH) and Korean Institute of Tuberculosis (KIT). Species identification was carried by high-performance liquid chromatography (strains isolated in UUH) or by polymerase chain reaction (PCR)-restriction fragment length polymorphism analysis of the rpoB gene (strains provided by KIT) (14, 21-23). Strains were maintained on Lowenstein-Jensen media slants at room temperature until required for testing. To prepare the inoculum suspension for broth microdilution tests, strains were subcultured in cation-supplemented Mueller-Hinton broth and incubated at 35℃ for 2 to 4 days to a turbidity of ≥0.5 McFarland standard by visual examination.
Antimicrobial susceptibility testing was performed by the broth microdilution method using cation-supplemented Mueller-Hinton broth, according to CLSI guidelines (CLSI broth microdilution method) (15, 17). Inoculum suspensions were prepared by diluting broth culture supernatant in sterile distilled water to a density of 0.5 McFarland standards using a nephelometer (BioMérieux Vitek Inc, Hazelwood, MO, U.S.A.). The final concentrations of mycobacteria ranged from 1×105 to 5×105 CFU/mL. Suspensions were mixed well on a vortex mixer and dispensed using a multichannel pipette into each well of a 96-well microplate (SPL, Hwasung, Korea) containing broth medium and antimicrobial drugs at serial concentrations. Final inoculums were between 1×104 and 5×104 CFU per well in a volume of 0.2 mL. Inoculated plates were covered and incubated at 35℃. A 5% sheep blood agar plate was also inoculated with a loopful of the final inoculum suspension to check for purity. If quality control results were not acceptable, or contamination or mixed culture was suspected, all data were discarded. For M. fortuitum, growth endpoints were read visually after 3 days of incubation. For M. abscessus, trays were re-incubated and read daily for up to 5 days until bacterial growth in the control well was good enough for reading. MIC values were defined as the lowest concentration of drug that inhibited visible growth, except for sulfamethoxazole, for which the concentration that inhibited growth by 80 to 90% versus a control well with no drug was used, according to the CLSI guideline (15). MIC readings for imipenem were taken at 72 hr for all strains. All measurements were performed in duplicate for each strain. To assess potential impact on patient management, MIC results were interpreted based on the RGM interpretive category using the breakpoints suggested by CLSI.
The colorimetric broth microdilution method with STC (STC broth microdilution) was identical to the broth microdilution method described by CLSI in terms of reagents, medium preparation, inoculum, drug concentrations, and incubation time, with one exception, namely, that STC was added to the cation-supplemented Mueller-Hinton broth with antimicrobial agents to a final concentration of 50 µg/mL. After bacterial growth, dark-colored precipitates were observed in wells containing STC-added media. After adding a solubilizing agent and incubating for 2 hr, wells showing microbial growth were pink-colored. Color changes corresponding to microbial growth and each strain-drug-MIC endpoint were recorded visually at 72 hr. The broth microdilution method using STC was checked by comparing growth in each test well with that in control wells. Wells containing the lowest drug concentration that showed a prominent reduction in color change were considered MICs. For sulfamethoxazole, wells colored light pink were considered to reflect MIC endpoints, and these showed growth inhibition of 80 to 90% versus control wells.
Levels of agreement between the two broth microdilution methods with or without STC were calculated for each strain-drug-MIC endpoint and were expressed as percentages at the same MIC or as MICs within one or two 2-fold dilutions. The interpretive category results were also analyzed by comparing the results of two methods so as to determine whether the performance was acceptable according to the CLSI guideline (24). The discrepancy cases were classified to very major error (resistant by CLSI method but susceptible by STC method), major error (susceptible by CLSI method, resistant by STC method), and minor error (intermediate result was obtained by one method but not by the other). The rates of agreement were calculated as the percentage of isolates with MICs in the range of two 2-fold dilution.
The MIC results determined by using the CLSI broth microdilution method are shown in Table 1 and 2 for seven drugs and a number of strains. M. fortuitum strains achieved maximum growth at 72 hr for the broth microdilution test methods with or without STC. For most strains of M. abscessus, maximum growth in broth also occurred at 72 hr, but some strains (10 of 48) showed maximum growth at 96 hr. M. fortuitum showed consistently low MICs to amikacin and ciprofloxacin, and a wide range of MICs to the other five drugs. Isolates of M. abscessus showed a wide range of MICs to all drugs. MIC results converted to interpretive categories using the breakpoints suggested are shown in Table 3. For M. fortuitum, all strains were fully susceptible to amikacin, and most strains were susceptible or intermediately susceptible to cefoxitin, ciprofloxacin, clarithromycin, and imipenem. For M. abscessus, most strains were susceptible or intermediately susceptible to amikacin, cefoxitin, ciprofloxacin, and clarithromycin. Doxycycline, imipenem, and sulfamethoxazole showed lower MICs for M. fortuitum isolates and higher MICs for M. abscessus.
Comparisons of the growth of a strain of M. fortuitum on the broth media are shown in Fig. 1, 2 in 96-well microplates, which demonstrate an obvious advantage of the STC broth microdilution method. The MICs of 7 antimicrobial agents obtained using the STC broth microdilution method correlated well with those obtained using the CLSI broth dilution method (Table 4). Agreements by interpretative category for the two methods are presented in Table 5. In 85 of 103 bacterial strains (44 of 55 M. fortuitum strains and 41 of 48 M. abscessus strains), the MICs of all 7 antimicrobial agents tested were within two 2-fold dilutions of those obtained by the CLSI method. For each of these agents, the overall agreement at±2 log2 dilution ranged from 95.8% to 100.0% between the two methods except for the result of M. fortuitum to sulfamethoxazole (48 of 55 strains, 87.3%). Agreements of CLSI and STC broth microdilution methods by interpretative category results to amikacin, cefoxitin, clarythromycin, and doxycycline were excellent without major errors. Because the MIC results of M. fortuitum for cefoxitin were clustered at 16-32 µg/mL, the breakpoint of the susceptible and intermediate categories, 15 minor errors were identified. Similarly, the MICs of M. abscessus for ciprofloxacin were clustered at 1-2 µg/mL, which corresponded to 19 minor and one very major errors. In total 721 organism-antimicrobial agent combinations were analyzed, 9 of 18 MIC discrepancies (out of two 2-fold dilution), 5 of 6 major errors, and 8 of 9 very major errors originated uniquely from sulfamethoxazole test results (Table 5).
In general, the MIC results of M. fortuitum and M. abscessus isolates to antimicrobial drugs in this study were similar to those previously reported by other investigators (1, 14, 25-28). Most of the tested strains of M. fortuitum were found to be susceptible or intermediately susceptible to amikacin (100%), cefoxitin (100%), ciprofloxacin (100%), clarithromycin (93%), doxycycline (84%), and imipenem (100%). In addition, most strains of M. abscessus were susceptible or intermediately susceptible to amikacin (96%), cefoxitin (94%), and clarithromycin (96%). Ciprofloxacin and imipenem results for M. abscessus showed more susceptible than the results by other researchers (1, 14, 26).
It is known that results for RGM susceptibility to imipenem show a lack of reproducibility, so in a routine laboratory setting, it is recommended to repeat the test if the MIC for M. fortuitum is >8 µg/mL, and to report the MIC result with the comment 'MIC greater than expected for this species' if the repeat result is >8 µg/mL (15). In this study, no strain was found to have an MIC higher than 8 µg/mL. The MIC results of M. abscessus to imipenem were mainly clustered in the range 8-16 µg/mL, but the CLSI guideline suggests that MIC results should not be reported because imipenem MICs for this species tend not to be reproducible, which results in major susceptibility category changes (15, 28).
Susceptibility testing is indicated for any RGM that is considered clinically significant (e.g., isolates from blood, tissue, skin, and soft tissue lesions) (14, 15), and clinical laboratories require standardized methods for determining the antimicrobial susceptibilities of RGM. Broth microdilution is convenient and widely used for the rapid susceptibility testing to several antibiotics of large numbers of bacterial isolates. However, MICs as determined by the broth microdilution are obtained by taking visual turbidity readings, which makes the test subjective and variable (15, 17). Moreover, the growth of RGM in microtiter trays frequently does not result in a well-defined button in the bottom of a well, as occurs with rapidly growing aerobic and facultative bacteria. In addition, when testing clarithromycin susceptibility in broth, some rapidly growing mycobacteria, particularly M. fortuitum, had trailing end points with certain drugs.
Because of variabilities in the appearances of the growths of M. fortuitum and M. abscessus in microdilution trays, interpretation of MICs may be difficult. To avoid these drawbacks, the colorimetric redox indicator STC was used in the broth microdilution method in the present study. Results could be read visually or by spectrophotometry, and the use of a tetrazolium compound as an indicator made reading straight-forward because of the obvious color change (19, 20), which overcomes the problems frequently encountered in the conventional broth microdilution test, such as, inoculum sedimentation or scant or transparent growth (20, 29). Several attempts have been made to use colorimetric determinations of broth microdilution endpoints. These methods are attractive in that they generate clear-cut endpoints based on visual color changes. STC is an oxidation-reduction indicator which, in the presence of growing organisms, changes from colorless to pink. Furthermore, it has been used to test the susceptibilities of M. tuberculosis to antimycobacterial drugs (18, 19). Moreover, it has been shown to have some benefits when compared with other colorimetric redox indicators, such as 3-(4,5-dimethyl-2-thiazy)-2,5-diphenyl-2H-tetrazolium bromide (MTT) or 2,3-bis (2-methoxy-4-nitro-5-sulfophenyl)-5-[(phenyl-amino) carbonyl]-2H-tetrazolium hydroxide (XTT) (30, 31). However, the use of these tetrazolium derivatives has several drawbacks. In MTT assays, a large number of cells are necessary (32), and XTT is expensive for routine use in clinical laboratories. Additionally for MTT and XTT methods, substrate should be added after growth has begun, and the time of this addition is not well defined (33), and in our experience the developed color disappears within a day. In the modified susceptibility testing method using STC, the substrate can be added to media at the time of preparing media before inoculating bacteria. The plate agitation process can be eliminated, which is necessary in the MTT and XTT methods and may cause reading errors. In the present study, an excellent correlation was obtained between the results of the CLSI and STC methods. Especially the MIC and interpretive category results obtained from sulfamethoxazole susceptibility tests showed some discrepancies. We consider that the lack of an intermediate category of interpretation criteria causes major or very major errors even for a 2-fold dilution MIC difference. The CLSI guideline recommends that MIC results of sulfamethoxazole should be read at the concentration in which there is 80% or greater reduction in growth as compared to the control because antagonists in the medium may allow some slight growth (15, 28). Actually small growth of organism in microwell could be skipped in the CLSI broth microdilution method while more easily detected by color change of media in the STC method. Thus sulfamethoxazole susceptibility results should be cautiously interpreted in both broth microdilution methods.
In this study, we describe the general susceptibility patterns of M. fortuitum and M. abscessus in Korea. In addition, it was demonstrated that the STC broth microdilution method is reliable, easy to perform, and inexpensive for susceptibility testing of isolates of M. fortuitum and M. abscessus to 7 antimicrobial agents. In comparison with the broth microdilution method approved by CLSI, the colorimetric MIC testing by the modified broth microdilution method using STC could provide a useful means for the antibiotic susceptibility testing for RGM.
Figures and Tables
![]() | Fig. 1Growth of M. fortuitum in Mueller-Hinton broth. (A) Media without STC, and (B) STC-containing media. |
![]() | Fig. 2Growth of M. fortuitum in Mueller-Hinton broth. (A) Media without STC, (B) solubilizing agent added in STC-containing media, and (C) STC-contatining media, in which dark precipitates were observed before solubilization. |
Table 1
Minimal inhibitory concentrations (MICs) of 7 antimicrobial agents for 55 strains of M. fortuitum by CLSI broth microdilution
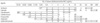
Table 2
Minimal inhibitory concentrations (MICs) of 7 antimicrobial agents for 48 strains of M. abscessus by CLSI broth microdilution
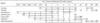
Table 3
Susceptibility results of rapidly growing nontuberculous mycobacteria by CLSI broth microdilution
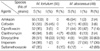
Table 4
Agreement by MICs of antimycobacterial agents among CLSI and STC broth microdilution methods
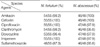
References
1. Wagner D, Young LS. Nontuberculous mycobacterial infections: a clinical review. Infection. 2004. 32:257–270.


2. Choudhri S, Manfreda J, Wolfe J, Parker S, Long R. Clinical significance of nontuberculous mycobacteria isolates in a Canadian tertiary care center. Clin Infect Dis. 1995. 21:128–133.


3. Hosker HS, Lam CW, Ng TK, Ma HK, Chan SL. The prevalence and clinical significance of pulmonary infection due to non-tuberculous mycobacteria in Hong Kong. Respir Med. 1995. 89:3–8.


4. Marras TK, Daley CL. Epidemiology of human pulmonary infection with nontuberculous mycobacteria. Clin Chest Med. 2002. 23:553–567.
5. Scientific Committee in Korean Academy of Tuberculosis and Respiratory diseases. National survey of mycobacterial diseases other than tuberculosis in Korea. Tuberc Respir Dis. 1995. 42:277–294.
6. Lee JY, Choi HJ, Lee HY, Joung EY, Huh JW, Oh YM, Lee SD, Kim WS, Kim DS, Kim WD, Shim TS. Recovery rate and characteristics of nontuberculous mycobacterial isolates in a university hospital in Korea. Tuberc Respir Dis. 2005. 58:385–391.


7. Koh WJ, Kwon OJ, Lee KS. Nontuberculous mycobacterial pulmonary diseases in immunocompetent patients. Korean J Radiol. 2002. 3:145–157.


8. Lew WJ. Clinical manifestations and epidermiology of nontuberculous mycobacterial infection in Korea. Tuberc Respir Dis. 2002. 53:83–87.
9. Griffith DE. Mycobacteria as pathogens of respiratory infection. Infect Dis Clin North Am. 1998. 12:593–611.


10. Lee HW, Kim MN, Shim TS, Bia GH, Pai CH. Nontuberculous mycobacterial pulmonary infection in immunocompetent patients. Tuberc Respir Dis. 2002. 53:173–182.


11. Koh WJ, Kwon OJ, Jeon K, Kim TS, Lee KS, Park YK, Bai GH. Clinical significance of nontuberculous mycobacteria isolated from respiratory specimens in Korea. Chest. 2006. 129:341–348.


12. Yim JJ, Han SK. Diagnosis and treatment of nontuberculous mycobacterial pulmonary diseases. J Korean Med Assoc. 2005. 48:563–570.


13. Falkinham JO 3rd. Epidemiology of infection by nontuberculous mycobacteria. Clin Microbiol Rev. 1996. 9:177–215.


14. American Thoracic Society. Diagnosis and treatment of disease caused by nontuberculous mycobacteria. This official statement of the American Thoracic Society was approved by the Board of Directors, March 1997. Medical Section of the American Lung Association. Am J Respir Crit Care Med. 1997. 156:S1–S25.
15. Clinical and Laboratory Standards Institute. CLSI document M24-A. Susceptibility testing of mycobacteria, Norcardiae, and Other Aerobic Actinomycetes; Approved Standard. 2003. Wayne, Pa. USA: CLSI.
16. Clinical and Laboratory Standards Institute. CLSI document M100-S15. Performance standards for antimicrobial susceptibility testing; fifteenth informational supplement. 2005. Wayne, Pa. USA: CLSI.
17. Clinical and Laboratory Standards Institute. CLSI document M7-A6. Methods for dilution antimicrobial susceptibility tests for bacteria that grow aerobically; Approved standard-sixth edition. 2003. Wayne, Pa. USA: CLSI.
18. Yamane N, Oiwa T, Kiyota T, Saitoh H, Sonoda T, Tosaka M, Nakashima M, Fukunaga H, Masaki T, Miyagawa K, Miyagoe M, Okazawa Y. Multicenter evaluation of a colorimetric microplate antimycobacterial susceptibility test: comparative study with the NCCLS M24-P. Rinsho Byori. 1996. 44:456–464.
19. Lee S, Kong DH, Yun SH, Lee KR, Lee KP, Franzblau SG, Lee EY, Chang CL. Evaluation of a modified antimycobacterial susceptibility test using Middlebrook 7H10 agar containing 2,3-diphenyl-5-thienyl-(2)-tetrazolium chloride. J Microbiol Methods. 2006. 66:548–551.


20. Shin JH, Choi JC, Lee JN, Kim HH, Lee EY, Chang CL. Evaluation of a colorimetric antifungal susceptibility test by using 2,3-diphenyl-5-thienyl-(2)-tetrazolium chloride. Antimicrob Agents Chemother. 2004. 48:4457–4459.


21. Lee H, Park HJ, Cho SN, Bai GH, Kim SJ. Species identification of mycobacteria by PCR-restriction fragment length polymorphism of the rpoB gene. J Clin Microbiol. 2000. 38:2966–2971.
22. Butler WR, Jost KC Jr, Kilburn JO. Identification of mycobacteria by high-performance liquid chromatography. J Clin Microbiol. 1991. 29:2468–2472.


23. Butler WR, Floyd MM, Silcox V, Cage G, Desmond E, Duffey PS, Guthertz LS, Gross WM, Kenneth C, Jost J, Ramos LS, Thibert L, Warren N. Mycolic acid pattern standards for HPLC identification of mycobacteria. 1999. Atlanta: Centers for Deasese Control and Prevention.
24. Clinical and Laboratory Standards Institute. Development of in vitro susceptibility testing criteria and quality controls parameters, Approved guideline M23-A2. 2001. Wayne: CLSI.
25. Brown BA, Wallace RJ Jr, Onyi GO, De Rosas V, Wallace RJ 3rd. Activities of four macrolides, including clarithromycin, against Mycobacterium fortuitum, Mycobacterium chelonae, and M. chelonaelike organisms. Antimicrob Agents Chemother. 1992. 36:180–184.


26. Wallace RJ Jr, Bedsole G, Sumter G, Sanders CV, Steele LC, Brown BA, Smith J, Graham DR. Activities of ciprofloxacin and ofloxacin against rapidly growing mycobacteria with demonstration of acquired resistance following single-drug therapy. Antimicrob Agents Chemother. 1990. 34:65–70.


27. Woods GL. Susceptibility testing for mycobacteria. Clin Infect Dis. 2000. 31:1209–1215.
28. Woods GL, Bergmann JS, Witebsky FG, Fahle GA, Wanger A, Boulet B, Plaunt M, Brown BA, Wallace RJ Jr. Multisite reproducibility of results obtained by the broth microdilution method for susceptibility testing of Mycobacterium abscessus, Mycobacterium chelonae, and Mycobacterium fortuitum. J Clin Microbiol. 1999. 37:1676–1682.
29. Rahman M, Kuhn I, Rahman M, Olsson-Liljequist B, Mollby R. Evaluation of a scanner-assisted colorimetric MIC method for susceptibility testing of gram-negative fermentative bacteria. Appl Environ Microbiol. 2004. 70:2398–2403.


30. Mosmann T. Rapid colorimetric assay for cellular growth and survival: application to proliferation and cytotoxicity assays. J Immunol Methods. 1983. 65:55–63.


31. Roehm NW, Rodgers GH, Hatfield SM, Glasebrook AL. An improved colorimetric assay for cell proliferation and viability utilizing the tetrazolium salt XTT. J Immunol Methods. 1991. 142:257–265.


32. Garn H, Krause H, Enzmann V, Drossler K. An improved MTT assay using the electron-coupling agent menadione. J Immunol Methods. 1994. 168:253–256.


33. Hawser SP, Norris H, Jessup CJ, Ghannoum MA. Comparison of a 2,3-bis (2-methoxy-4-nitro-5-sulfophenyl)-5-[(phenylamino)carbonyl]-2H-t etrazolium hydroxide (XTT) colorimetric method with the standardized National Committee for Clinical Laboratory Standards method of testing clinical yeast isolates for susceptibility to antifungal agents. J Clin Microbiol. 1998. 36:1450–1452.