Abstract
Bronchiolitis is a risk factor for the development of childhood asthma. Eosinophilic inflammation in airways plays an important role in the pathophysiology of both bronchiolitis and asthma. To investigate this inflammation, we measured the eosinophil cationic protein (ECP), regulated on activation normal T-cell expressed and secreted (RANTES) and eotaxin levels in nasopharyngeal secretions (NPS). Twenty-eight patients with RSV bronchiolitis (RSV group), 11 patients with non-RSV bronchiolitis (non-RSV group) and 7 controls were enrolled in this study. ECP, RANTES, and eotaxin levels were measured by enzyme immunoassays. The ECP level in the NPS of the RSV group was significantly higher than that in the NPS of the non-RSV group and controls. RANTES and eotaxin levels in infants with bronchiolitis were significantly higher than those in the controls, but there was no significant difference between the RSV and non-RSV groups. In conclusion, with regard to eosinophilic airway inflammation, as compared with non-RSV bronchiolitis, RSV bronchiolitis may be more similar to childhood asthma.
Respiratory syncytial virus (RSV) is the most common causative agent of lower respiratory tract disease in infants (1). Bronchiolitis, one of the lower respiratory tract diseases in infants, is an important obstructive airway disease in pediatric populations. The clinical manifestations of bronchiolitis include cough, wheezing, and dyspnea; these symptoms are similar to those of bronchial asthma and RSV infection, and can provoke and aggravate bronchial asthma. RSV infection plays a significant role in inducing recurrent wheezing and bronchial asthma. More than 50% of bronchiolitis cases are caused by RSV. Furthermore, as compared to non-RSV bronchiolitis, RSV bronchiolitis is more prone to develop into bronchial asthma (1-7).
The pathogenesis of both bronchial asthma and bronchiolitis involves many cytokines and chemokines, such as interleukin (IL)-5 and IL-8, whose levels are regulated following the activation of normal T cells. They are regulated on activation normally T-cell expressed and secreted (RANTES), eotaxin, and MIP 1-α (macrophage inflammatory protein) that cause allergic inflammatory reactions (1, 2, 5, 7-10). Among these cytokines, RANTES and eotaxin play significant roles in inducing chemotaxis and activating eosinophils that are one of the most important factors in allergic inflammation. Assuming that the clinical manifestations are similar to those of bronchial asthma and have a high possibility of developing into bronchial asthma, the immunopathophysiology of bronchiolitis can be considered to be similar to that of bronchial asthma. In addition, similar chemokine and eosinophil activation can also be observed in bronchiolitis.
It has been known that RSV selectively acts on respiratory epithelial cells in airway diseases such as bronchiolitis and bronchial asthma. However, since RSV infection promotes not only local reactions but also systemic manifestations, the systemic actions of RSV cannot be overlooked. No significant research data has yet been reported to support this concept.
In this study, the very important factors involved in the immunopathophysiology of bronchial asthma such as eosinophilic cationic protein (ECP), RANTES, and eotaxin were examined in the nasopharyngeal secretions (NPS) of patients with bronchiolitis to investigate their possible roles in the pathophysiology of bronchiolitis.
Patients admitted to Kangnam St. Mary's Hospital from January to December 2002, with no evidence of bacterial infections and no specific medical histories were selected as study groups. The study groups comprised patients initially diagnosed with bronchiolitis on the basis of the following symptoms: mild fever, cough, tachypnea, wheezing due to chest auscultation, and hyperinflation detected by chest radiography. Based on the NPS examination, the patients were divided into two groups; 28 patients with RSV bronchiolitis (RSV group) and 11 patients with non-RSV bronchiolitis (non-RSV group). The control group comprised 7 patients who were admitted to the hospital for elective operations without any evidence of respiratory or gastrointestinal infections. All the patient' parents gave their informed consent in accordance with a protocol approved by the Ethics Committee of Kangnam St. Mary's Hospital, The Catholic University of Korea.
NPS was aspirated from the nasal cavities of the patients using an 8-French aseptic catheter, and collected in a 15-mL conical tube containing 2 mL of transfer media (gentamicin 50 µg/mL, fungizone 500 µg/mL, penicillin G 340 U/mL). The collected nasal secretion samples were transferred to the laboratory where they were mixed and centrifuged for 5 min at 1,500 r.p.m.
Primary detection of infection with RSV, adenovirus, parainfluenza virus, or influenza virus A and B was made by carrying out an indirect immunofluorescence assay (IFA) using cells obtained from the nasal cavity. To confirm viral infection, 0.1-0.2 mL of the supernatant was inoculated in 2 sets of test tubes. The first set contained human epidermal carcinoma cells (HEp-2, CCL-23; ATCC) that were cultured as a monolayer in Modified Eagle's Media (MEM, Life Technologies GIBCO BRL, Grand Island, NY, U.S.A.) supplemented with 10% fetal calf serum. The second set of test tubes contained Madin Darby canine kidney cells (MDCK, CCL-34; ATCC). After inoculation, we cultured the cells in a CO2 incubator at 37℃ and observed them for the development of any cytopathologic effect for 10 days. If cytopathologic effects were detected in the HEp-2 cells (RSV, adenovirus) or MDCK cells (parainfluenza virus, influenza virus A and B), an indirect immunofluorescence assay against RSV was performed again to confirm the infection. A part of the nasopharyngeal secretion was stored at 70℃, and frozen until the measurement of chemokine levels.
For secondary detection, the HEp-2 cells showing cytopathologic effects were scraped from the well, rinsed twice with phosphate buffered saline (PBS, pH 7.4), placed on 10-hole slides (10 µL/well), and dried. The dried cells were fixed with acetone at 4℃, mixed with primary antibodies to RSV (Chemicon International, Temecula, CA, U.S.A.) and adenovirus (Chemicon International), and incubated at 37℃ for 1 hr. Primary antibodies to the parainfluenza virus (Chemicon International) and influenza viruses A and B (Chemicon International) were added to the MDCK cells and incubated at 37℃ for 1 hr.
After the reaction, the cells were washed twice with PBS, and FITC-conjugated (Fluorescein isothiocyanate, ICN Pharmaceuticals Inc., OH, U.S.A.) secondary antibodies were added. Subsequently, the cells were incubated at 37℃ for 1 hr, washed twice with PBS, and dried well; following this, mineral oil was added to the cells. An immunofluorescence microscope was used to detect cells that showed definite fluorescence; these were considered to be positive for viral infection.
On the day of admission, the patient's peripheral blood was drawn using a vacuum tube containing heparin (Belliver Industrial Estate, Plymouth, U.K.) and sent to a clinical pathology laboratory for an eosinophil count. Another small volume of blood was collected in a blood separation container (Becton Dickinson Vacutainer SST, Rutherford, NJ, U.S.A.) and centrifuged at 2,500 r.p.m. for 10 min, and then stored frozen at -70℃ until required. The total serum IgE level measured by fluorescent enzyme immunoassay (FEIA, UniCAPTM100, Pharmacia Diagnostics AB, Uppsala, Sweden) ranged from 500 kU/L to 2,000 kU/L.
After heating the frozen NPS, a quantitative sandwich enzymatic immunoassay (R&D Systems, Minneapolis, MN, U.S.A.) was performed to measure the concentration of RANTES and eotaxin. The minimum measurable concentration was 5 pg/mL. The ECP level that was measured by fluorescent enzyme immunoassay (FEIA, UniCAPTM100, Pharmacia Diagnostics AB, Uppsala, Sweden) ranged from 2 µg/L to 200 µg/L.
Comparisons were made among the RSV, non-RSV, and control groups as well as between the bronchiolitis and control groups by using the Mann-Whitney U test using SPSS for Windows version 10.0.
The correlation among ECP, RANTES, and eotaxin levels in the NPS of the RSV group was analyzed by Spearman's rank correlation test, and a p value less than 0.05 was considered to be statistically significant.
There was no significant difference in the age, serum IgE level, and eosinophil count in the peripheral blood between the control and bronchiolitis groups. The patients' ages ranged from 1 month to 24 months (Table 1).
The ECP level in NPS was significantly higher in both the RSV (76±70.9 µg/L, p=0.001) and non-RSV groups (26±32.9 µg/L, p=0.012) when compared to the ECP level of control group (2.5±0.7 µg/L). The ECP level in the NPS of RSV group was significantly higher than one of non-RSV group (p=0.033). The ECP level in NPS from the bronchiolitis group (61.9±66.2 µg/L) was statistically higher than that of the control group (p=0.0013). This was more prominently observed in the case of RSV bronchiolitis, in which a significant difference was observed in the ECP levels between the RSV and non-RSV groups (Fig. 1, Table 2).
The RANTES level was significantly elevated in the bronchiolitis group (77.5±3.9 pg/mL) compared to that of the control group (3.9±1.9 pg/mL). There was no significant difference in RANTES levels between the RSV (89.1±134.3 pg/mL) and non-RSV groups (48.17±70.60 pg/mL). It was significantly higher in the RSV group than in the control group (p=0.013) (Fig. 2, Table 2).
The eotaxin level was significantly higher (p=0.010, Fig. 3) in the bronchiolitis group (60.0±45.4 pg/mL) than in the control group (22.0±22.9 pg/mL). The increase in eotaxin levels was more prominent in the RSV group (60.07±47.07 pg/mL) than in the non-RSV group (59.64±43.08 pg/mL) (p=0.049). However, there was no significant difference in the eotaxin levels between the RSV and non-RSV groups (Fig. 3, Table 2).
The clinical manifestation of bronchiolitis is similar to that of bronchial asthma, and RSV infection that causes bronchiolitis can induce or aggravate an asthmatic attack and the symptoms of asthma. Since it is highly likely that RSV bronchiolitis may later develop into bronchial asthma, there may be a high degree of similarity between RSV bronchiolitis and bronchial asthma with regard to inducing airway inflammation (1, 11, 12).
If the clinical symptoms of RSV bronchiolitis are similar to those of bronchial asthma and if there exists a high possibility of developing bronchial asthma from bronchiolitis, at least a part of the immunopathophysiology of the two diseases may appear to be similar with regard to eosinophilic activation in airway inflammatory reactions, RANTES, and eotaxin secretion during cellular activation in asthma. These occurrences may also be observed in bronchiolitis, particularly RSV bronchiolitis.
ECP, an indicator of eosinophilic activation (13), RANTES, and eotaxin were significantly higher in the NPS of patients with bronchiolitis than in the NPS of patients in the control group. This was more prominently observed in patients with RSV bronchiolitis. ECP is one of the most potent toxic proteins secreted by eosinophils, and participates in powerful toxic reactions (8, 11). Furthermore, it is known to act protectively against parasitic infections and participate in airway inflammatory diseases such as bronchiolitis caused by RSV infection (11, 14). In addition, ECP in the nasal secretions of bronchiolitic children has been extremely useful in predicting the future development of persistent asthma (12, 15).
From our study, the ECP level was higher in NPS of the bronchiolitis group than in the control group. This was more prominently observed in the RSV bronchiolitis group than in the non-RSV bronchiolitis group. According to Sung et al. (14), the ECP level in the nasal secretions of patients with lower airway diseases, such as RSV infection and influenza, was significantly higher in patients with wheezing. According to Oh and coworkers (16), the ECP level in nasal secretions in patients with bronchial asthma, RSV, or influenza virus-induced bronchiolitis was significantly higher than that in the control group. In particular, the ECP level in patients with bronchial asthma was significantly high, yet there were no significant differences among the different virus groups of bronchiolitis patients. In our study, we did not differentiate the degree of increase in ECP level among each virus group; however, a significant difference was observed in the ECP levels between the RSV and non-RSV bronchiolitis groups. In summary, the eosinophil count increases in RSV bronchiolitis, this is similar to bronchial asthma; however, further investigation is required to determine the presence of similar reactions in other respiratory diseases. The significant difference in ECP levels is attributed more to the localized effect of RSV on the target tissue rather than to the systemic effect of RSV. This correlates with the report that performing eosinophil and ECP counts using a patient's sputum, rather than peripheral blood, is a more accurate measure of the airway inflammatory reaction (17, 18).
RANTES and eotaxin are CC chemokine equivalent. RANTES induces chemotactic activity in eosinophils, basophils, monocytes and memory T cells (1, 2, 8, 9, 15), while eotaxin selectively induces chemotactic activity in eosinophils (20, 21). Respiratory viruses, particularly RSV, activate the formation of RANTES in airway epithelial cells (7, 9, 15).
Eotaxin is produced in endothelial cells, mast cells, fibroblasts, airway epithelial cells, smooth muscle cells, chondrocytes, eosinophils, and various other cells. It is activated by inflammatory cytokines such as IL-1, TNF-α, and IFN-γ in a manner similar to that of RANTES activation.
RANTES and eotaxin can be assumed to be closely associated with the pathophysiology of bronchiolitis, particularly RSV bronchiolitis, since eosinophil plays important role in the pathophysiology of bronchiolitis. There is also a report that the production of RANTES differs with the degree of RSV proliferation and airway epithelial cell infection. Therefore, the RANTES level in nasal secretions is related to the severity of RSV bronchiolitis (1, 9). In lower respiratory diseases, local inflammation is caused by the cytopathic effect of RSV on the airway epithelial cells and the host's systemic inflammatory reactions (1). RANTES level in the NPS of the bronchiolitis group was significantly higher than that of the control group. This was more prominent in the RSV group, suggesting that the RANTES level in NPS may be influenced to a greater extent by RSV.
According to Olszewska-Pazdrak et al. (7), RSV infection promotes RANTES production in the airway epithelial cells. RANTES also promotes eosinophilic entry into the airway; the eosinophils then produce RANTES following RSV infection. Therefore, compared to non-RSV bronchiolitis an increased level of RANTES is observed in RSV bronchiolitis; this is consistent with our experiment. In this study, the RANTES level is significantly higher in RSV bronchiolitis than in the NPS of patients of the control group. In non-RSV bronchiolitis, the RANTES level was higher than that of the control group; however, the increase was not statistically significant.
Compared to the number of reports on RANTES, there have been few reports on eotaxin in RSV bronchiolitis. In our study the eotaxin level in NPS from the bronchiolitis group was increased. However, there was no significant difference observed between the RSV and non-RSV groups.
The ECP, RANTES, and eotaxin levels in NPS of the bronchiolitic group were significantly higher than that in the control group. In particular, the ECP level was significantly different between RSV bronchiolitis and non-RSV bronchiolitis. When considering reports that the serum ECP is not significantly increased in RSV bronchiolitis or infant wheezers, NPS may be a more predictable material for measuring inflammatory reactions in lower airway diseases such as bronchiolitis and asthma.
Unlike significant correlations between the levels of ECP, RANTES, eotaxin in RSV group, the significances between RSV and non-RSV group are different. One of the reasons for the different significances, may be that many inflammatory cells including eosinophil and their mediators interact with complexity in the airway inflammation in each group.
In conclusion, the pathophysiologic mechanism in bronchiolitis may be similar to that in childhood asthma with regard to airway eosinophilic inflammation. In particular, a few differences were evident between RSV and non-RSV bronchiolitis. This similarity may be associated with asthma inception later in life due to RSV bronchiolitis. Further investigation is required on this subject.
Figures and Tables
Fig. 1
ECP levels in nasopharyngeal secretion are significantly different between group (p<0.05).
RSV (+), RSV bronchiolitis; RSV (-), non-RSV bronchiolitis.
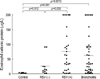
Fig. 2
RANTES levels in nasopharyngeal secretion of infants with RSV bronchiolitis are significantly higher than those of controls (p<0.05).
RSV (+), RSV bronchiolitis; RSV(-), non-RSV bronchiolitis.
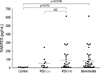
Fig. 3
Eotaxin levels in nasopharyngeal secretion of infants with RSV bronchiolitis as well as those of infants with non-RSV bronchiolitis are significantly higher than those of controls (p<0.05).
RSV (+), RSV bronchiolitis; RSV (-), non-RSV bronchiolitis.
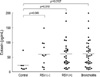
Fig. 4
ECP level in nasopharyngeal secretion of infants with RSV bronchiolitis show highly correlated with eotaxin level (p=0.02, r=0.562) than with RANTES level (p=0.0497, r=0.36).
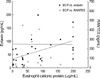
Fig. 5
RANTES and eotaxin levels in nasopharyngeal secretion of infants with RSV bronchiolitis show significant correlation (r=0.4392, p=0.039).
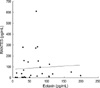
References
1. Sheeran P, Jafri H, Carubelli C, Saavedra J, Johnson C, Krisher K, Sandros PJ, Ramilo O. Elevated cytokine concentrations in the nasopharyngeal and tracheal secretions of children with respiratory syncytial virus disease. Pediatr Infect Dis J. 1999. 18:115–122.


2. Noah TL, Ivins SS, Murphy P, Kazachkova I, Moats-Staats B, Henderson FW. Chemokines and inflammation in the nasal passages of infants with respiratory syncytial virus bronchiolitis. Clin Immunol. 2002. 104:86–95.


3. Schwarze J, Hamelmann E, Bradley KL, Takeda K, Gelfand EW. Respiratory syncytial virus infection results in airway hyperresponsiveness and enhanced airway sensitization to allergen. J Clin Invest. 1997. 100:226–233.


4. Greenough A. Respiratory syncytial virus infection: clinical features, management, and prophylaxis. Curr Opin Pulm Med. 2002. 8:214–217.


5. Wang SZ, Forsyth KD. The interaction of neutrophils with respiratory epithelial cells in viral infection. Respirology. 2000. 5:1–10.


6. Byington CL, Castillo H, Gerber K, Daly JA, Brimley LA, Adams S, Christenson JS, Pavia AT. The effect of rapid respiratory viral diagnostic testing on antibiotic use in a children's hospital. Arch Pediatr Adolesc Med. 2002. 156:1230–1234.


7. Olszewska-Pazdrak B, Casola A, Saito T, Alam R, Crowe SE, Mei F, Ogra PL, Garofalo RP. Cell-specific expression of RANTES, MCP-1, and MIP-1-alpha by lower airway epithelial cells and eosinophils infected with respiratory syncytial virus. J Virol. 1998. 72:4756–4764.
8. Harrison AM, Bonville CA, Rosenberg HF, Domachowske JB. Respiratory syncytial virus-induced chemokine expression in the lower airways. Am J Respir Crit Care Med. 1999. 159:1918–1924.
9. Hornsleth A, Loland L, Larsen LB. Cytokines and chemokines in respiratory secretion and severity of disease in infants with respiratory syncytial virus (RSV) infection. J Clin Virol. 2001. 21:163–170.


10. Garofalo R, Dorris A, Ahlstedt S, Welliver RC. Peripheral blood eosinophil counts and eosinophil cationic protein content of respiratory secretions in bronchiolitis: relationship to severity of disease. Pediatr Allergy Immunol. 1994. 5:111–117.


11. Colocho Zelaya EA, Orvell C, Strannegard O. Eosinophil cationic protein in nasopharyngeal secretions and serum of infants infected with respiratory syncytial virus. Pediatr Allergy Immunol. 1994. 5:100–106.


12. Pifferi M, Ragazzo V, Caramella D, Baldini G. Eosinophil cationic protein in infants with respiratory syncytial virus bronchiolitis: predictive value for subsequent development of persistent wheezing. Pediatr Pulmonol. 2001. 31:419–424.


13. Venge P. Role of eosinophils in childhood asthma inflammation. Pediatr Pulmonol Suppl. 1995. 11:34–35.


14. Sung RY, Hui SH, Wong CK, Lam CW, Yin J. A comparison of cytokine responses in respiratory syncytial virus and inflenza A infections in infants. Eur J Pediatr. 2001. 160:117–122.
15. Chung HL, Kim SG. RANTES may be predictive of later recurrent wheezing after respiratory syncytial virus bronchiolitis in infants. Ann Allergy Asthma Immunol. 2002. 88:463–467.


16. Oh JW, Lee HB, Park IK, Kang JO. Interleukin-6, interleukin-8, interleukin-11, and interferon-gamma levels in nasopharyngeal aspirates from wheezing children with respiratory syncytial virus or influenza A virus infection. Pediatr Allergy Immunol. 2002. 13:350–356.
17. Pizzichini E, Pizzichini MM, Efthimiadis A, Dolovich J, Hargreave FE. Measuring airway inflammation in asthma: eosinophils and eosinophilic cationic protein in induced sputum compared with peripheral blood. J Allergy Clin Immunol. 1997. 99:539–544.


18. Rosi E, Ronchi MC, Grazzini M, Duranti R, Scano G. Diagnostic accuracy of sputum outcomes in chronic stable asthma. Clin Exp Allergy. 2000. 30:577–584.


19. Shields MD, Brown V, Stevenson EC, Fitch PS, Schock BC, Turner G, Taylor R, Ennis M. Serum eosinophil cationic protein and blood eosinophil counts for the prediction of the presence of airways inflammation in children with wheezing. Clin Exp Allergy. 1999. 29:1382–1389.
20. Ponath PD, Qin S, Ringler DJ, Clark-Lewis I, Wang J, Kassam N, Smith H, Shi X, Gonzalo JA, Newman W, Gutierrez-Ramos JC, Mackay CR. Cloning of the human eosinophil chemoattractant, eotaxin. Expression, receptor binding, and functional properties suggest a mechanism for the selective recruitment of eosinophils. J Clin Invest. 1996. 97:604–612.

