Abstract
Mesenchymal stem cells (MSC) are multipotent in nature and believed to facilitate the engraftment of hematopoietic stem cells (HSC) when transplanted simultaneously in animal studies and even in human trials. In this study, we transfected culture-expanded MSC with granulocyte macrophage-colony stimulating factor (GM-CSF) and stem cell factor (SCF) cytokine genes and then cotransplanted with mononuclear cells (MNC) to further promote HSC engraftment. MNC were harvested from cord blood and seeded in long-term culture for ex vivo MSC expansion. A total of 1×107 MNC plus MSC/µL were introduced to the tail vein of nonobese diabetic/severe combined immunodeficiency mice. After 6-8 weeks later, homing and engraftment of human cells were determined by flow cytometry and fluorescence in situ hybridization studies. The total nucleated cell count and the engraftment of CD45+/CD34+ cells and XX or XY positive human cells were significantly increased in cotransplanted mice and even higher with the cytokine gene-transfected MSC (GM-CSF>SCF, p<0.05) than in transplantation of MNC alone. These results suggest that MSC transfected with hematopoietic growth factor genes are capable of enhancing the hematopoietic engraftment. Delivering genes involved in homing and cell adhesions, CXCR4 or VLA, would further increase the efficiency of stem cell transplantation in the future.
The bone marrow stroma contains primitive and potentially self-renewing cells that can differentiate into various mesenchymal cells. These mesenchymal stem cells (MSC) are capable of supporting the expansion and differentiation of hematopoietic stem cells (HSC) in vitro and enhance hematopoietic engraftment in vivo (1-5). Furthermore, MSC express class I, but not class II, histocompatibility antigens, and they are not immunogenic in in vitro assays and in preclinical or clinical models.
Recently, it has been reported that there are mesenchymal precursors in cord blood and peripheral blood of normal individuals. The results obtained demonstrate that after systemic infusion of cord blood MSC into nude mice, human DNA was found in the marrow and in ex vivo expanded stromal cells grown from the marrow of transplanted animals. Several groups also have reported bone and fat differentiation capacity from cord blood-derived nonhematopoietic cells (6-10). Despite the low initial frequency in cord blood, MSC have a tremendous expansion potential in culture. Due to the limited number of HSC in a given cord blood unit, the outcome of HSC transplantation (HSCT) could be improved by coengraftment of the MSC in addition to the hematopoietic precursors.
Several lines of evidence suggest that MSC produce some essential hematopoietic growth factors (1-5). Based on these reports, we hypothesized that exogenously administered MSC with cytokine gene-transfected would show additive or augmented effects on HSC expansion. In this study, mononuclear cells (MNC) were harvested from cord blood and seeded in long-term culture for ex vivo MSC expansion. We transfected culture-expanded MSC with granulocyte macrophage-colony stimulating factor (GM-CSF) and stem cell factor (SCF) cytokine genes and then cotransplanted with cord blood MNC in nonobese diabetic/severe combined immunodeficiency (NOD/SCID) mice to further promote HSC engraftment. Although CD34+ cells can be selected for the preparation of HSC, many cord blood HSCT practically use unselected MNC. Therefore, we chose unselected MNC samples instead of selected samples as the source of stem cells for transplantation. Our results show that human cells were significantly increased in cotransplanted mice, and the number was even higher with the cytokine gene-transfected MSC than with transplantation of MNC alone. These data not only provide direct evidence for the presence of MSC in cord blood, but could also add to therapeutic profile of expanded MSC for clinical use.
Cord blood samples were collected from the umbilical vein after full-term vaginal delivery. Informed consent was obtained from all mothers. The mononuclear cell fractions from cord blood were suspended at a concentration of 1×106 cells/mL in MesenCult medium (StemCell Technologies, Inc., Vancouver, BC, Canada) and seeded in T-25 flasks. Cultures were maintained at 37℃ in humidified air and 5% CO2 with a regular change of medium. After removal of non-adherent cells, the adherent layer was further cultured until 80-90% confluence. At this point, the cell population was expanded by successive passage and resulting adherent cells were used for experiments.
The adherent cells were detached with 0.05% trypsin-ethylenediamine tetraacetic acid and washed with phosphate-buffered saline (PBS). We observed morphology of detached cells after Wright-Giemsa staining. The phenotype and purity of MSC were assessed by flow cytometry. The cells were washed with PBS containing 2% bovine serum albumin, and incubated with FITC-conjugated CD45 (BD Biosciences, San Jose, CA, U.S.A.), PE-conjugated CD73 (BD Biosciences), CD105 (DiNonA, Inc., Seoul, Korea), and CD166 (BD Biosciences) on ice for 30 min in the dark. Those 3 monoclonal antibodies were chosen because of the popularity and accessibility. After fixation with 1% paraformaldehyde, flow cytometry was performed on the FACSort instrument (Becton Dickinson, San Jose, CA, U.S.A.).
For plasmid transfections, pEGFP-N1 DNA (Clontech, Palo Alto, CA, U.S.A.) that encodes an enhanced green fluorescent protein (EGFP) was used. Expanded MSC were transfected with an EGFP-expressing vectors after cloning with GM-CSF (pEGFP-N1/GM-CSF) or SCF (pEGFP-N1/SCF). To create pEGFP-N1/GM-CSF or pEGFP-N1/SCF, human peripheral blood monocytes were used for reverse transcription-polymerase chain reaction. After sequencing of GM-CSF and SCF genes, EcoRI was used for digestion to insert cDNA3.1 and NotI was treated for SCF. Stable MSC transfectants that expressed high levels of GFP were cloned by limiting dilution and selected using 2 mg/mL neomycin (Invitrogen Corp., Carlsbad, CA, U.S.A.). The GFP-expressing cells were screened by a microplate spectrofluorometer (Shimadzu, Kyoto, Japan) and confirmed by an enzyme-linked immunosorbent assay (ELISA) (R & D Systems, Minneapolis, MN, U.S.A.). Stable clones of MSC with GFP and GM-CSF or SCF were maintained in Dulbecco Modified Eagle Medium (Gibco Laboratories, Grand Island, NY, U.S.A.) in the presence of neomycin until transplantation. GFP-positive MSC were visualized using a fluorescence microscope (Olympus, Japan) and then used for transplantation to NOD/SCID mice.
NOD/SCID mice were purchased from Korea Research Institute of Bioscience and Biotechnology (KRIBB, Daejeon, Korea) and were maintained in sterile microisolator cages. All mice received sublethal total body irradiation with a single dose of 250 cGy. Twelve to 24 hr after irradiation, cells in a final volume of 200 µL were injected through the tail vein. We designed 4 experimental groups, and each group received 1×107 cells as a total: group 1 (n=5) received MNC only, group 2 (n=7) received a mixture of MNC plus MSC, 0.5×107 respectively, group 3 (n=7) received a mixture of MNC plus GM-CSF MSC, 0.5×107 respectively, and group 4 (n=6) received a mixture of MNC plus SCF MSC, 0.5×107 respectively, per mouse. MNC were isolated from cord blood using a density gradient centrifugation. All cells were used fresh without cryopreservation.
Six to 8 weeks later, blood samples were collected from the retroorbital venous plexus of the NOD/SCID mice. An aliquot of the blood was used for complete blood cell counts by Sysmex XE-2100 (Sysmex Corp., Kobe, Japan). Human cell engraftment was detected by flow cytometry, using FITC-conjugated CD45 (BD Biosciences) and PE-conjugated CD34 (BD Biosciences) antibodies. Positive cells were identified by comparison with isotype controls. A part of venous blood was also exposed to a hypotonic solution, and fixed in methanol/acetic acid fixative. Nuclei were then stained with CEP X (DXZ1) alpha satellite probe (Vysis, Inc., Downers Grove, IL, U.S.A.) and CEP Y (DYZ1) satellite III probe (Vysis) for fluorescence in situ hybridization (FISH) identification of human cells. A total of at least 500-700 nuclei were examined in each case under a fluorescent microscope.
The results were expressed as mean±standard deviation. Differences in engraftment percentages between experimental conditions were analyzed by Student-t test. A p value less than 0.05 was considered statistically significant.
Adherent MSC could be expanded from all of the cord blood analyzed. Primary cultures of MSC mainly consisted of colonies of spindle-shaped fibroblastoid cells (Fig. 1A). Confluence was usually reached after 2-3 weeks. Wright-Giemsa staining of detached MSC showed a large cell population characterized by abundant cytoplasms with occasional vacuoles and projections, fine nuclear chromatins, and one or more nucleoli (Fig. 1B). Flow cytometry analyses demonstrated that MSC expressed CD73, CD105, and CD166 in all cases, but were negative for CD45 (Fig. 2). The purity of expanded MSC was 85-97%.
At 24 hr after transfection, increased GFP expression was identified both by spectrofluorometry and ELISA. The transfected MSC showed visible GFP expression as early as 3 hr after transfection. The highest efficiency of the transfection was obtained after 5-7 days of culture both with pEGFP-N1/GM-CSF and pEGFP-N1/SCF (Fig. 3, 4). On average 50-70% of transfected MSC expressed GFP. Detached MSC were then ready for transplantation. ELISA detected increased GM-CSF levels from MSC cultures after transfection (273.78±148.33 pg/mL) compared to those before transfection (34.35±17.06 pg/mL) (p<0.05, n=4). SCF also increased from 1.30±1.05 pg/mL to 24.10±20.21 pg/mL post transfection, however, there were no statistical differences (p>0.05, n=4).
In MNC transplantation alone, the absolute numbers of total nucleated cell (TNC) count was 0.08±0.04 (×103/µL) (n=5) comparable to 0.13±0.05 (×103/µL) (n=7) in mice transplanted with MNC plus MSC (Table 1). However, higher levels of human XX or XY cell by FISH (Fig. 5) were found in transplantation of MNC plus MSC, 0.41±0.41 (×103/µL) vs. 1.52±0.81 (×103/µL) (p<0.05). Cotransplantation of MNC and GM-CSF-transfected MSC most enhanced engraftment. The TNC count, 4.63±0.96 (×103/µL) (n=7), and the percentage of XX or XY cells, 5.68±0.58 (×103/µL), were significantly higher (p<0.05) than those after transplantation of MNC alone. Also, higher levels of TNC count, 2.93±0.33 (×103/µL) (n=6), and XX or XY cells, 3.43±0.49 (×103/µL), were obtained following cotransplantation of MNC plus SCF-transfected MSC (p<0.05). Engraftment study with immunophenotypic analysis of CD45+/CD34+ cells by flow cytometry showed similar results (Table 1, Fig. 6).
Genetic modification of stem cells is an attractive target for gene therapy because of their higher proliferative capacity and long-term survival compared with other somatic cells. MSC have been demonstrated to be able to express exogenous protein for an extended period of time and to maintain this ability after transplantation in vitro (1, 11-14). Gene modified MSC are useful as therapeutic tools for various tumors. MSC with forced expression of interferon beta were shown to inhibit the growth of malignant cells in vivo (11, 12). Gene-modification of MSC with therapeutic cytokines also clearly augmented the antitumor effect and prolonged the survival of tumor-bearing animals (13). Recently more efficient non-viral DNA transfection methods have been introduced to obtain stably transfected human cells (14, 15).
Ex vivo expanded MSC have been infused in several transplantation studies. It is possible that a therapeutic benefit from MSC could be obtained by local production of growth factors and the provision of temporary immunosuppression. Human MSC have a high expansion potential, genetic stability, and reproducible characteristics proven in widely dispersed laboratories (1-3). No adverse events during or after MSC infusion have been reported, and no ectopic tissue formation has been observed.
Based on these results, we hypothesized that exogenously administered MSC with cytokine gene-transfected would show additive or augmented effects on HSC expansion. Among the various cytokines, we have previously demonstrated that the combination of SCF, thrombopoietin, and flt3 ligand is sufficient for ex vivo expansion of cord blood (16). With the addition of granulocyte (G)-CSF, the clonogenic and homing potentials of cord blood were even higher, although statistically significant differences were not observed. Ramirez et al. (17) also have shown that SCF, interleukin (IL)-3, IL-6, and GM-CSF could induce the up-regulation of several adhesion molecules involved in the mobilization and homing of HSC. Currently GM-CSF is one of the most widely used cytokines for the purpose of stem cell mobilization and for the treatment of neutropenic patients. Therefore, we transfected culture-expanded cord blood MSC with GM-CSF and SCF cytokine genes, and then cotransplanted with cord blood MNC in NOD/SCID mice to further promote HSC engraftment. We demonstrated that human cells were significantly increased in cotransplanted mice and even higher with the cytokine gene-transfected MSC than in transplantation of MNC alone. Cotransplantation of MNC and GM-CSF-transfected MSC most enhanced engraftment.
Cotransplantation studies in animal models as well as in humans showed that primary or culture-expanded MSC promote the engraftment of HSC (18-22). Cotransplantation of MSC and cord blood (18, 19, 21) or mobilized peripheral blood (20) CD34+ cells resulted in a significantly higher level of engraftment than with transplantation of CD34+ cells only. Cotransplantation of human leukocyte antigen (HLA)-identical sibling culture-expanded MSC with an HLA-identical sibling HSC was also feasible and safe without immediate infusional or late MSC-associated toxicities (22). The mechanism of the enhancing effect is still unknown. Several lines of evidence suggest that MSC produce several essential hematopoietic growth factors including IL-6, IL-11, SCF, leukemia inhibitory factor, and flt3 ligand and express adhesion molecules and extracellular matrix proteins that are known to play an important role in HSC homing such as VCAM1, E-selectin, collagen I, and fibronectin (1-5, 20, 23). Different studies report that MSC have an immunomodulatory effect and are able to suppress proliferation of T lymphocytes both by cell-cell interaction and via soluble factors (22-25).
It has been claimed that there are mesenchymal precursors in cord blood and peripheral blood of normal individuals (6-8, 26, 27). Despite the low initial frequency in cord blood, MSC have a tremendous expansion potential in culture. Compared to bone marrow MSC, cord blood cultures were slower to establish, but once established, the growth was maintained over multiple passages. Using optimized isolation and culture conditions, cells showing a characteristic mesenchymal morphology and immune phenotype were isolated. The frequency of MSC ranged from 0-2.3 clones per 1×108 MNC. Due to the limited number of HSC in a given cord blood unit, the outcome of HSC transplantation could be improved by coengraftment of the MSC in addition to the hematopoietic precursors.
Erices et al. (8) previously investigated the capability of transplanted cord blood MSC to home and survive in the marrow of nude mice. They have detected human DNA after transplantation in the marrow of recipients as well as in ex vivo-expanded stromal cells prepared from the marrow of transplants. In this study we found that cord blood MSC cotransplantation enhances human cell engraftment. This enhancement was greater after cotransplantation of GM-CSF- and SCF-transfected MSC. According to Wagner et al. (26) several genes involved in mesodermal differentiation were differently expressed on MSC from human bone marrow, adipose tissue, or umbilical cord blood. These results implied that potentials of mesodermal development might be different depending on MSC preparations. By upregulating genes involved in proliferation and homing of cord blood MSC, this might contribute to establish a reliable genetic modification system for clinical applications.
MSC are multipotent and believed to facilitate the engraftment of HSC when transplanted simultaneously in animal studies, and recently, even in human trials. In this study, we transfected culture-expanded cord blood MSC with GM-CSF and SCF cytokine genes and then cotransplanted with cord blood HSC to further promote HSC engraftment. The TNC count and the engraftment of CD45+/CD34+ cells and XX or XY positive human cells were significantly increased in cotransplanted mice and even higher with the cytokine gene-transfected MSC (GM-CSF>SCF, p<0.05) than in transplantation of HSC alone. Our results indicate that MSC may serve as a platform to deliver hematopoietic growth factors in clinical stem cell transplantation. Delivering genes involved in homing and cell adhesions, CXCR4 or VLA, would further increase the efficiency of stem cell transplantation in the future.
Figures and Tables
Fig. 1
Inverted phase contrast microscopic findings of cultured cord blood MSC at passage 2, day 14 (A) (×150). Wright-Giemsa stained smear of the harvested MSC (B) (×1,000).
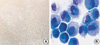
Fig. 2
Representative immunophenotypic analysis of MSC ex vivo cultures from the cord blood. CD45-/CD73+ (95.7%), CD45-/CD 105+ (97.4%), and CD45-/CD166+ cells (94.9%) were evident by flow cytometry.
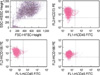
Fig. 3
GM-CSF transfection studies. Microscopic images of phase contrast (A) and fluorescence microscopes (B) before transfection. Micrographs of phase contrast (C) and fluorescence microscopes (D) post transfection day 5. The panel D shows GFP fluorescence, indicating GFP-expressing MSC after transfection (×200).
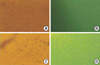
Fig. 4
SCF transfection studies. Microscopic images of phase contrast (A) and fluorescence microscopes (B) before transfection. Micrographs of phase contrast (C) and fluorescence microscopes (D) post transfection day 7. The panel D shows GFP fluorescence, indicating GFP-expressing MSC after transfection (×200).
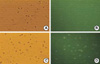
Fig. 5
XY human cells identified from the peripheral blood of NOD/SCID mice 7 weeks after cotransplantation of GM-CSF-transfected cord blood MSC and MNC (×1,000). One orange signal indicates one copy of the X chromosome, and one green signal indicates one copy of the Y chromosome.
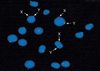
References
1. Kassem M. Mesenchymal stem cells: biological characteristics and potential clinical applications. Cloning Stem Cells. 2004. 6:369–374.


2. Le Blanc K, Pittenger MF. Mesenchymal stem cells: progress toward promise. Cytotherapy. 2005. 7:36–45.


3. Kan I, Melamed E, Offen D. Integral therapeutic potential of bone marrow mesenchymal stem cells. Curr Drug Targets. 2005. 6:31–41.


4. Jiang Y, Jahagirdar BN, Reinhardt RL, Schwartz RE, Keene CD, Ortiz-Gonzalez XR, Reyes M, Lenvik T, Lund T, Blackstad M, Du J, Aldrich S, Lisberg A, Low WC, Largaespada DA, Verfaillie CM. Pluripotency of mesenchymal stem cells derived from adult marrow. Nature. 2002. 418:41–49.


5. Jones EA, Kinsey SE, English A, Jones RA, Straszynski L, Meredith DM, Markham AF, Jack A, Emery P, McGonagle D. Isolation and characterization of bone marrow multipotential mesenchymal progenitor cells. Arthritis Rheum. 2002. 46:3349–3360.


6. Erices A, Conget P, Minguell JJ. Mesenchymal progenitor cells in human umbilical cord blood. Br J Haematol. 2000. 109:235–242.


7. Goodwin HS, Bicknese AR, Chien SN, Bogucki BD, Oliver DA, Quinn CO, Wall DA. Multilineage differentiation activity by cells isolated from umbilical cord blood: expression of bone, fat, and neural markers. Biol Blood Marrow Transplant. 2001. 7:581–588.


8. Erices AA, Allers CI, Conget PA, Rojas CV, Minguell JJ. Human cord blood-derived mesenchymal stem cells home and survive in the marrow of immunodeficient mice after systemic infusion. Cell Transplant. 2003. 12:555–561.


9. Huss R, Lange C, Weissinger EM, Kolb HJ, Thalmeier K. Evidence of peripheral blood-derived, plastic-adherent CD34(-/low) hematopoietic stem cell clones with mesenchymal stem cell characteristics. Stem Cells. 2000. 18:252–260.
10. Villaron EM, Almeida J, Lopez-Holgado N, Alcoceba M, Sanchez-Abarca LI, Sanchez-Guizo FM, Alberca M, Perez-Simon JA, San Miguel JF, Del Canizo MC. Mesenchymal stem cells are present in peripheral blood and can engraft after allogeneic hematopoietic stem cell transplantation. Haematologica. 2004. 89:1421–1427.
11. Studeny M, Marini FC, Champlin RE, Zompetta C, Fidler IJ, Andreeff M. Bone marrow-derived mesenchymal stem cells as vehicles for interferon-β delivery into tumors. Cancer Res. 2002. 62:3603–3608.
12. Studeny M, Marini FC, Dembinski JL, Zompetta C, Cabreira-Hansen M, Bekele BN, Champlin RE, Andreeff M. Mesenchymal stem cells: potential precursors for tumor stroma and targeted-delivery vehicles for anticancer agents. J Natl Cancer Inst. 2004. 96:1593–1603.


13. Hamada H, Kobune M, Nakamura K, Kawano Y, Kato K, Honmou O, Houkin K, Matsunaga T, Niitsu Y. Mesenchymal stem cells (MSC) as therapeutic cytoreagents for gene therapy. Cancer Sci. 2005. 96:149–156.


14. Nakashima S, Matsuyama Y, Nitta A, Sakai Y, Ishiguro N. Highly efficient transfection of human marrow stromal cells by nucleofection. Transplant Proc. 2005. 37:2290–2292.


15. Martinet W, Schrijvers DM, Kockx MM. Nucleofection as an efficient nonviral transfection method for human monocytic cells. Biotechnol Lett. 2003. 25:1025–1029.
16. Lee YH, Han JY, Seo SY, Kim KH, Lee YA, Lee YS, Lee HS, Hur WJ, Han H, Kwon HC, Kim JS, Kim HJ. Stem cells expressing homing receptors could be expanded from cryopreserved and unselected cord blood. J Korean Med Sci. 2001. 19:635–639.


17. Ramirez M, Segovia JC, Benet I, Arbona C, Guenechea G, Blaya C, Garcia-Conde J, Bueren JA, Prosper C. Ex vivo expansion of umbilical cord blood (UCB) CD34+ cells alters the expression and function of α 4 β 1 and α 5 β 1 integrins. Br J Haematol. 2001. 115:213–221.
18. Noort WA, Kruisselbrink AB, in't Anker PS, Kruger M, van Bezooijen RL, de Paus RA, Heemskerk MH, Lowik CW, Falkenburg JH, Willemze R, Fibbe WE. Mesenchymal stem cells promote engraftment of human umbilical cord blood-derived CD34+ cells in NOD/SCID mice. Exp Hematol. 2002. 30:870–878.


19. in't Anker PS, Noort WA, Kruisselbrink AB, Scherjon SA, Beekhuizen W, Willemze R, Kanhai HH, Fibbe WE. Nonexpanded primary lung and bone marrow-derived mesenchymal cells promote the engraftment of umbilical cord blood-derived CD34+ cells in NOD/SCID mice. Exp Hematol. 2003. 31:881–889.
20. Angelopoulou M, Novelli E, Grove JE, Rinder HM, Civin C, Cheng L, Krause DS. Cotransplantation of human mesenchymal stem cells enhances human myelopoiesis and megakaryocytopoiesis in NOD/SCID mice. Exp Hematol. 2003. 31:413–420.


21. Bensidhoum M, Chapel A, Francois S, Demarquay C, Mazurier C, Fouillard L, Bouchet S, Bertho JM, Gourmelon P, Aigueperse J, Charbord P, Gorin NC, Thierry D, Lopez M. Homing of in vitro expanded Stro-1- or Stro-1+ human mesenchymal stem cells into the NOD/SCID mouse and their role in supporting human CD34 cell engraftment. Blood. 2004. 103:3313–3319.


22. Lazarus HM, Koc ON, Devine SM, Curtin P, Maziarz RT, Holland HK, Shpall EJ, McCarthy P, Atkinson K, Cooper BW, Gerson SL, Laughlin MJ, Loberiza FR Jr, Moseley AB, Bacigalupo A. Cotransplantation of HLA-identical sibling culture-expanded mesenchymal stem cells and hematopoietic stem cells in hematologic malignancy patients. Biol Blood Marrow Transplant. 2005. 11:389–398.


23. Majumdar MK, Thiede MA, Haynesworth SE, Bruder SP, Gerson SL. Human marrow-derived mesenchymal stem cells (MSCs) express hematopoietic cytokines and support long-term hematopoiesis when differentiated toward Stromal and osteogenic lineages. J Hematother Stem Cell Res. 2000. 9:841–848.


24. Chung NG, Jeong DC, Park SJ, Choi BO, Cho B, Kim HK, Chun CS, Won JH, Han CW. Cotransplantation of marrow stromal cells may prevent lethal graft-versus-host disease in major histocompatibility complex mismatched murine hematopoietic stem cell transplantation. Int J Hematol. 2004. 80:370–376.


25. Maitra B, Szekely E, Gjini K, Laughlin MJ, Dennis J, Haynesworth SE, Koc ON. Human mesenchymal stem cells support unrelated donor hematopoietic stem cells and suppress T-cell activation. Bone Marrow Transplant. 2004. 33:597–604.


26. Wagner W, Wein F, Seckinger A, Frankhauser M, Wirkner U, Krause U, Blake J, Schwager C, Eckstein V, Ansorge W, Ho AD. Comparative characteristics of mesenchymal stem cells from human bone marrow, adipose tissue, and umbilical cord blood. Exp Hematol. 2005. 33:1402–1416.


27. Bieback K, Kern S, Kluter H, Eichler H. Clinical parameters for the isolation of mesenchymal stem cells from umbilical cord blood. Stem Cells. 2004. 22:625–634.