Abstract
Genetic alterations have been recognized as an important event in the carcinogenesis of gastric cancer (GC). We conducted high resolution bacterial artificial chromosome array-comparative genomic hybridization, to elucidate in more detail the genomic alterations, and to establish a pattern of DNA copy number changes with distinct clinical variables in GC. Our results showed some correlations between novel amplified or deleted regions and clinical status. Copy-number gains were frequently detected at 1p, 5p, 7q, 8q, 11p, 16p, 20p and 20q, and losses at 1p, 2q, 4q, 5q, 7q, 9p, 14q, and 18q. Losses at 4q23, 9p23, 14q31.1, or 18q21.1 as well as a gain at 20q12 were correlated with tumor-node-metastasis tumor stage. Losses at 9p23 or 14q31.1 were associated with lymph node status. Metastasis was determined to be related to losses at 4q23 or 4q28.2, as well as losses at 4q15.2, 4q21.21, 4q 28.2, or 14q31.1, with differentiation. One of the notable aspects of this study was that the losses at 4q or 14q could be employed in the evaluation of the metastatic status of GC. Our results should provide a potential resource for the molecular cytogenetic events in GC, and should also provide clues in the hunt for genes associated with GC.
Gastric cancer (GC) is one of the most frequently encountered malignancies in the world, and has a high prevalence in Korea. Despite the high incidence of this condition, the genetic events which result in gastric malignancy and the genetic components which are altered during the inception and natural history of the neoplasm have yet to be elucidated in detail (1).
Chromosome-based comparative genomic hybridization (CGH) enables a genome-wide analysis of DNA sequence copy numbers within in a single experimental procedure, and has previously uncovered crucial information regarding the chromosomal changes inherent in a variety of tumors (2,3). Several chromosomal regions in which gains or losses are frequently encountered have been identified in both premalignant gastric lesions and gastric adenocarcinomas, using chromosome-based CGH (4-7), thereby suggesting that complicated genetic alterations are a component of the process of gastric carcinogenesis. However, conventional CGH provides no information which is sufficiently precise to enable the flagging of the exact locations of oncogenes or tumor suppressor genes (1). This may be attributable to the relatively low resolution which is currently achievable by chromosomal CGH assays, and in addition CGH data tend to be less suitable for quantitative analyses, such as genomic tumor profiling (8).
Hybridization to an array of mapped DNA sequences may sometimes alleviate the limitations associated with conventional CGH. Hybridization other advantages over conventional CGH, as well as over other currently-employed screening and diagnostic methods. Copy number alterations detected by array CGH can be related directly to the sequence information, and these results in a dramatic increase in the speed with which novel cancer-causing genes can be identified (9). It also enables the high-throughput quantitative measurement of high-resolution DNA copy number changes throughout the entirety of the genome (10), and constitutes a significant advance in identifying piece of knowledge in the hunt for genes associated with solid tumors (1,11). More recently, several cDNA and oligo-based transcript-centric array-CGH methods have been employed in an attempt to profile a variety of cancers, including gastric cancer (12). The measurement of gene label copy number variations might be achieved through the use of these types of arrays. However, genomic aberrations on the scale of the entire chromosomal structure, including the segmental duplicated, intergenic, subtelomeric, and telomeric regions, all of which may perform critical functions in the development and progression of cancers, may be greatly minimized with the use of these techniques (13). In order to ameliorate these inherent limitations, some researchers have begun to employ bacterial artificial chromosome (BAC)/PAC-based array-CGH methods, enabling them to conduct effective comparisons of clinically relevant genomic aberrations throughout the entirety of the genome (10). There have been a few previous studies which have focused on the characterization of chromosomal alterations in GC by array-CGH, but the molecular events which culminate in gastric malignancy, as well as the clinical correlations between these aberrations and the events comprising these conditions, remain to be clearly elucidated.
The purpose of this study was, firstly, to screen for changes in DNA copy numbers, and to identify smaller chromosomal changes in cases of gastric adenocarcinoma, using high resolution BAC array-CGH and, secondly, to establish patterns of DNA copy number changes which can be associated with distinct clinical variables.
Twenty-eight gastric adenocarcinoma patients were studied for array CGH. Fresh tissues were obtained from the operating room of the Department of General Surgery at Chungnam National University Hospital in Daejon, Korea. All clinical data is shown in Table 1. All the patients were classified according to the WHO histological typing of gastric tumors and the UICC tumor-node-metastasis (TNM) staging system. The demographic and pathological data, including age, gender, the site of the tumor and the tumor stage were obtained by a review of the medical records.
DNA was extracted from fresh frozen tissues of advanced gastric cancer and microdissected cells from early gastric cancer. Tumor cells were separated by manual microdissection of 10 µm frozen tissue sections stained with hematoxyhin-eosin. Tumor areas with an estimated content of 70% or more tumor cells were microdissected using a sharp scalpel under the direction of a pathologist. The genomic DNA was extracted using a Genomic DNA purification kit (Promega, Madison, WI, U.S.A.). The detailed procedure of the DNA extraction in chronological order was digesting the frozen tissue or microdissected cells with a lysis buffer, adding 10 mg/mL proteinase K, incubating the samples overnight at 55℃, removing the RNA with RNAse, precipitating the unnecessary proteins, aliquoting the DNA from the supernatants followed by washing and suspending the DNA.
BAC clones were selected from Macrogen's proprietary BAC library (http://www.macrogen.com). Briefly, pECBAC1 (Frijters et al. 1997) was restricted with HindIII and size selected male DNA were used to generate a BAC library. The vectors for this library were transformed and grown in DH10B.
The clones were first selected bioinformatically to give an average genomic coverage of 2 Mb resolutions. All of the clones were two end-sequenced using Applied Biosystems 3,700 sequencers and their sequences were blasted and mapped according to their positions on the UCSC human genome database (http://www.genome.ucsc.edu). These clones were prepared by conventional alkaline lysis method to obtain BAC DNA. The DNA was sonicated to generate about 3 kb fragments before mixing with 50% DMSO spotting buffer. The arrays were manufactured by GeneMachine's OmniGrid100 using 24 pin format. BAC clones were represented on an array as triplicated spots and each array was pre-scanned using an Axon scanner for a proper spot morphology. The array used in this study consists of 1,440 human BACs, which were spaced approximately 2.3 Mb across the whole genome.
The labeling and hybridization protocols described by Pinkel et al. (15) were used, with some modifications. Briefly, 21 µL of solution containing 500 ng of normal DNA (reference) or tumor DNA (test), 20 µL of BioPrime® DNA Labeling System random primers solution (Invitrogen, San Diego, CA, U.S.A.) and water were combined and incubated for 5 min at 95℃, and subsequently cooled on ice. After the addition of 5 µL of 10× dNTPs labeling mix (1 mM dCTP, 2 mM dATP, 2 mM dGTP, 2 mM dTTP), 3 µL of 1 mM Cy-3 or Cy-5 dCTP (GeneChem, Seoul, Korea) and 40 U of BioPrime® DNA Labeling System Klenow fragment (Invitrogen), the mixture was gently mixed and incubated overnight at 37℃. The addition of 5 µL of BioPrime® DNA Labeling System Stop Buffer (Invitrogen) ended the reaction. After labeling, unincorporated fluorescent nucleotides were removed by use of QIAquick Spin columns (QIAGEN, Valencia, CA, U.S.A.). In one tube, Cy3-labeled sample and Cy5-labeled reference DNAs were mixed together, and 50 µg of human Cot I DNA (Invitrogen) 20 µL of 3 M sodium acetate and 600 µL of cold 100% ethanol were precipitated.
The pellet was resuspended in 40 µL of a hybridization solution containing 50% formamide, 10% dextran sulfate, 2×SSC, 4% SDS and 200 µg yeast tRNA. The hybridization solution was denatured for 10 min at 72℃ and was incubated subsequently for 1 hr at 37℃, to allow blocking of repetitive sequence. Hybridization was performed in slide chambers for 48 hr at 37℃. After post hybridization washes, arrays were rinsed, dried with spin, and scanned into two 16-bit TIFF image files using GenePix4200A two-color fluorescent scanner (Axon Instruments, Sunnyvale, CA, U.S.A.) and quantitated using GenePix software (Axon Instruments, Sunnyvale, CA U.S.A.). The log 2 transformed fluorescent ratios were calculated from background subtracted median intensity values, and these values were normalized according to the intensity normalization method. Chromosomal aberrations were categorized as a gain when the normalized log 2 transformed fluorescent ratio was higher than 0.25 and as a loss when this ratio was below -0.25. These two threshold values were chosen empirically by selecting a 3×SD value calculated from 30 normal male to normal female hybridization experiments.
For validation of microarray data, we conducted real-time quantitative reverse transcription-PCR (RT-PCR) using 28 gastric carcinomas, which had been used in the array-CGH experiment. Two genes were randomly selected from among the group of genes which had significant correlation with clinicopathological parameters. These genes were as follows: MAFB at 20q12, and were used for array-CGH experiment. Two genes were randomly selected from among the group of genes which had been correlated significantly with clinicopathological parameters. These genes were as follows: MAFB at 20q12, TSHR at 14q31.1. The gene copy number of known genes MAFB and TSHR was determined in the tumor samples with the following primers set for the 5'forward primer: GCTAAGCCTCTCACCCTAGGA and the 3'reverse primer: CCCTGAGGTCCCTTCTGAAC and the 5'forward primer: GGATCCCAAAGGGCTCCAT and the 3'reverse primer: AAGATGGTTGAAAACT-GGACTTCTGT respectively according to a previously described protocol with normalizing normal human pooled genomic DNA (Promega, Madison, WI, U.S.A.) as a calibrator sample.
RT-PCR reactions were performed on an ABI 7900 HT (Applied Biosystems, Foster City, CA, U.S.A.). Amplification mixtures (20 µL) contained template DNA (approximately 2 ng), 1 × TaqMan PCR MasterMix (Applied Biosystems, Foster City, CA, U.S.A.) and 900 nM of each primer. The cycling conditions comprised 10 min polymerase activation at 95℃, 40 cycles at 95℃ for 15 sec and 60℃ for 1 min. The relative copy numbers of the selected genes were determined in the tumor samples and on three normal control DNAs using the above listed primer pairs according to the manufacturer's protocol and the copy numbers were normalized based on the standard curve generated from those three control samples. For quantitative real time RT-PCR, 1.189 and 0.084 were used as the cutoff for gain and loss, respectively.
Our study aimed to determine the following issues; 1) whether any correlations could be drawn between the mean number of chromosomal alterations (gain or loss) and the TNM cancer stage; 2) whether gene pairs could be significantly correlated with clinicopathological variables (sex, age, lymph node status, metastatis status and TNM cancer stage); and 3) whether gene pairs exhibited concordant behavior, according to the results of categorical analyses.
We utilized several statistical analytical techniques to verify our results. Possible correlations between clinicopathological variables (sex, age, lymph node status, metastasis status and TNM cancer stage) and the copy-number status of each of the spots were evaluated via two-sided Fisher exact tests. We employed one-way ANOVA in our comparisons of the differences in the mean number of chromosomal alterations (gain or loss) between TNM tumor stages. Correlations between array-CGH results and RT-PCR measurements were assessed via Pearson's tests, and the concordance rates between the two tests were determined using conventional formulas. p values of <0.05 were considered to be significant.
Table 1 displays the summary of the results of array CGH analysis and the clinicopathological data obtained from 28 gastric cancer patients. A total of 1,440 qualified array BAC clones were applied for the purpose of data analysis, and we attempted to determine the amount of copy number changes occurring in the DNA sequences. All of our patients (28 of 28 cases) manifested chromosomal alterations (gain and loss) in more than one clone. Copy number gains were determined to be more frequent than losses in the majority of cases. The mean number of gained clones was 73.2 (range, 5-221), and the mean number of lost clones was 52.5 (range, 0-322). Relationships between TNM tumor stage and the mean number of chromosomal alterations (gain or loss) are shown in Fig. 3. The number of chromosomal alterations in the stage IV group (3/28) differed significantly from the number of chromosomal alterations detected in the other cancer stage groups. We determined there to be a statistically significant correlation between the number of lost chromosomal regions, and tumor stage (p=0.000).
The most frequent aberrations in terms of copy number gains (≥30% of patients) were encountered at the following locations: 1p, 5p, 7q, 8q, 11p, 16p, 20p and 20q. The most frequently detected losses (≥25% of patients) occurred at 1p, 2q, 4q, 5q, 7q, 9p, 14q, and 18q, as is shown in Table 2 and 3. An overview of all of the chromosomal aberrations is displayed in Fig. 1. Representative examples of high-resolution analyses of a few of these frequently-detected aberrations are shown in Fig. 2.
We explored the statistical relationship between chromosomal alterations and clinicopathological variables, and the results of these investigations are summarized in Table 4. The array CGH data were correlated with lymph node status (negative or positive), metastasis (negative or positive), differentiation grade (well or moderate/poor), TNM tumor stage (I, II, III, IV), gender (male or female), and age (<70 or 70+ yr). Tumors in which we detected a loss at 9p23 (66.7%) or at 14q31.1 (46.7%) tended to test lymph node metastasis. A significant level of correlation was also determined to exist between a loss at 4q23 or 4q28.2 and metastasis (p=0.038, p=0.036). The number of chromosomal alterations detected was found to be higher in metastatic tumors than in tumors without metastasis. A gain at 20q12, as well as losses at 4q23, 9p23, 14q31.1, or 18q21 were all related to the TNM tumor stage. The number of chromosomal alterations exhibited a tendency to increase directly with TNM stage. Furthermore, losses at chromosomal locations 4q15.2, 4q21.21, 4q28.2 or 14q31.1 were associated significantly with histological grade of differentiation, and a gain at 8q24.3 was significantly correlated with gender (p=0.02; 72.7% tumors showing 8q24 gain were from male patients). A gain at 17q25.3 was significantly associated with age (p=0.039, 50% of tumors exhibiting a gain at 17q25.3 were from patients who were more than 70 yr old).
Results of the array CGH trials were confirmed using quantitative real-time RT-PCR. For the purpose of validation, genes were randomly selected from among the group of genes that correlated significantly with clinicopathological parameters. These selected genes were as follows: MAFB at 20q12 and TSHR at 14q31.1. The MAFB gene was employed as a representative amplified gene, and TSHR was chosen as an example of a deleted gene. We compared the gene expression values generated by the two methods for each gene. In 75.0% of the cases (21 of 28), the array-CGH data were consistent with the quantitative real-time PCR data for the MAFB gene. When using the TSHR gene, the two data sets were concordant in 71.4% (20 of 28) of cases. We also analyzed correlation between real-time RT-PCR and array-CGH. The Pearson's correlation coefficient of each gene was as follows: MAFB 0.750* (p=0.000) and TSHR 0.631* (p=0.000). The comparison of gene expression values determined by both methods for MAFB and TSHR is shown in Fig. 4.
Gastric carcinogenesis is a complex and multifactorial process, and is associated with a multiplicity of etiologies and genetic alterations. Chromosomal instability in cases of gastric carcinoma appears to constitute a principal mechanism of genetic damage, as has been shown in a host of classical CGH studies which revealed a variety of chromosomal abnormalities (2-7). However, conventional CGH is characterized by limited sensitivity and resolution, primarily because of its substantial dependence on the morphology of the metaphase chromosomes. Due to this significant limitation, this method cannot be used to pinpoint the exact site of an amplicon (10).
Microarray-based CGH (array CGH), however, is a more sensitive technique, which was designed specifically for this purpose. In this technique, individual clones in arrays, rather than whole genomes as metaphases, can be hybridized with genomic DNA, allowing for the detection of dosage changes, with a resolution as precise as 0.2 to 0.4 Mb (16). The primary biological value inherent in high-resolution array CGH is its ability to detect small amplicons and deletions, which in many cases, appear to harbor specific oncogenes and tumor suppressor genes (17). In this study, we used a BAC array clone for CGH, in order to screen for high-resolution DNA copy number changes and to precisely identify amplifications across the entirety of the human genome, at an average resolution of 2.3 Mb.
The most frequently-detected gains and losses were determined to occur on chromosome 1, and in particular, 1p (gain: 82%, loss: 86%). Minimal regions of gain were found at 1p36.11-p33. This region includes the p73 gene. Increased p73 expression has been previously noted in cases of gastric carcinoma, and this has been correlated with the progression of tumors (17). Furthermore, the p73 protein often detected in gastric tumor tissue was known to be the wild-type (19). In our present study, we detected the amplification of p73 (1p36.32) in 43% (12/28) of tumors in advanced stages. This would appear to support the hypothesis that increases in the generation of p73 may constitute a passive process, which is secondary to the increased copy numbers of the gene (20). One of the more interesting findings of this study involved the consistent loss of DNA copy numbers at 1p, with a minimal region being detected at 1p12-p31.1. In particular, 24 tumors (86%) manifested a loss at 1p21.1 and a loss at 1p21.3 (32%). Losses at 1p have also been repeatedly detected and reported in previous CGH studies (2,21), but at far lower frequencies than were found in our study. Moreover, patients whose tumors demonstrated LOH at 1p had significantly shorter survival (p=0.017) than those patients whose tumors did not (22). This indicates that aberrations in these regions, may be involved in a nonrandom manner in the cytogenetic progression of gastric carcinomas. However, in this present study, we could not reach any significant differences between the loss of short arm of chromosome 1 and clinopathological variables.
A gain at 7q was the second most frequently encountered aberration, but was not statistically significant when correlated with the clinicopathological factors assessed in our study. Previous analyses have determined that this region contains growth factor-related genes which may be associated with chromosomal instability, and which this could not be statistically significantly correlated with the clinicopathological factors assessed in our study. Previous analyses have determined that this region contains growth factor-related genes which may be associated with chromosomal instability, which may in turn accelerate the progression of a tumor from early to advanced stages (11).
We noted a gain at 20q with fairly high frequency, with a minimal region occurring at 20q11.2-q13.1. In particular, a gain at 20q12 was correlated significantly with the stage of TNM tumors. Multiple regions of low and high-level copy number gains at 20q were significantly correlated with poor clinical prognoses, and appear to contribute to the cancer phenotype, especially with regard to the aspects of immortalization, genome instability, apoptosis, and increased proliferation (23). Several previously-undertaken CGH studies have identified the 20q region as the most frequent site of DNA gains in cases of gastric cancer (5,11). Candidate genes at 20q 12-13 include BTAK (20q13), AIB1 (20q12), TOP1 (20q12-13.1), NABC1 (20q13.2), and CYP24 (20q13.2) (16). The expression of all of these genes at 20q12-13 appears to be regulated not only by amplification, but also by several other mechanisms, including transcriptional activation.
DNA copy number gains at 8q region were also encountered with fairly high frequency. Gains at 8q24.3 were 60% (17/28), and four cases focused on 8q23-24, the region in which the oncogene c-MYC is located. This would appear to suggest that some genes on 8q, most notably 8q23-24, might facilitate the tumorigenesis of gastric cancer (24). In fact, the clones positioning this region have been end-sequenced and we confirmed the exact location using FISH (data not shown). These clones were also used to amplify the c-MYC gene via PCR amplification, followed by direct sequencing, in order to exactly identify the c-MYC locus.
The most striking finding of this study involves the losses detected at the 4q and 14q regions. Losses at 4q23 and 4q28 were associated with increased malignant gastric cancer behavior, and were significantly correlated with advanced clinical stage, metastasis, and poor differentiation. The shared areas of genetic deletion indicate that the genes which are inactivated at these loci normally exert regulatory effects on the behavior of cells in various states of differentiation (25). The loss of the long arm of chromosome 4 has been previously recognized as a common occurrence in tumors occurring in the gastroesophageal junction (25), lung (26), kidney (27), and breast (28). However, no genes have yet been confidently implicated in the deletions in the 4q region, and no consistently encountered genetic defects have been identified which can be used to predict clinical outcomes. Therefore, further study with larger numbers of well-characterized primary tumors will be required in order to determine the target genes which are related to this region.
Losses at 14q31.1 were correlated significantly with TNM cancer staging and differentiation. This result is largely consistent with the findings of previously undertaken molecular studies, in which losses at 14q31-32.1 were frequently encountered in cases of gastric carcinoma (29), and TSHR (14q31) and AKTI (14q32.3) were put forward as candidate tumor suppressor genes (TSGs) for stomach cancer (29). A loss at 14q by LOH has also been reported as one of the early events in the carcinogenesis of both the gastric and biliary tract (30).
Loss at 9p23 has been correlated significantly with lymph nodes invasion and TNM tumor stage. TNM tumor stage has, until now, been recognized as the most relevant prognostic determinant (31), and the loss of chromosome 9p has been reported to occur more frequently in cases of gastric cancers that are relapsed or histologically malignant (32). A potential tumor suppressor gene, p16 (INK4; 9p21), might be involved to some degree in this genomic aberration. Recently, alterations of CDKN2A at 9p21 have been confidently implicated in poorer prognoses in cases of gastric cancer, particularly with regard to benign and borderline cases (33).
According to our results, 18q21.33 (bcl2) and 18q21.1 (DCC) were frequently observed to exhibit losses, 28.6% and 25.0% respectively. Losses at 18q21.1, in particular, were correlated significantly with TNM tumor staging. Several candidate genes on 18q, Smad2 (18q21), Smad4 (18q21.1), and bcl2 (18q21.3) have previously been mapped to this chromosome (2). Our previous study showed that LOH at the DCC locus (18q21.1) is a frequently encountered characteristic of gastric cancer (30). In order to more reliably validate the array-CGH data, we conducted quantitative real-time RT-PCR on selected genes, and our results were concordant within the same specimens.
In summary, this study identified a series of specific amplified or deleted regions which could be clearly correlated with clinical variables which had not been previously emphasized. The most prominent finding of this study involved the loss of 4q and 14q, which were significantly linked to malignant aggravation and metastasis. Additional evaluations of the genes within these regions might provide us with valuable clues in the hunt for genes which can be associated with gastric carcinoma.
Figures and Tables
Fig. 1
Frequency of chromosomal copy number change in 28 gastroesophageal tumors. Gains and losses are shown as green and red bars, respectively, indicating the overall chromosomal copy number aberrations in the examined gastroesophageal tumors.
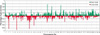
Fig. 2
Examples of microarray CGH results for four of the most frequently observed common regions. Whole genome profiles (A and D) are shown in the upper portion, and individual chromosome profiles (B, C, E and F) are shown in more detail below the profile of the entire genome. A representative chromosome profile, showing high-level amplifications from 3p14.2 (B) and 20q12 (C) in patient 18, and loss at 10q24.32 (E) and 14q31.1 (F) in patient 21.
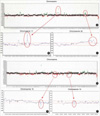
Fig. 3
Relationship between TNM tumor stage and the clone mean number of chromosomal alterations (gains and losses). Tumor staging was determined on the basis of the UICC criteria.
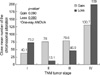
Fig. 4
Comparison of array-CGH data with quantitative real-time RT-PCR of two selected genes in 28 gastroesophageal tumors. (A) MAFB gene expression at 20q12. (B) TSHR gene expression at 14q31.1. Patient numbers are listed on the x-axis. The values of expression for genes with no changes fall between 0.084 and 1.189. Gray columns represent the real-time RT-PCR results, whereas those in black represent the array-CGH results. con, control; TO1-TO28, Tumor 1-28.
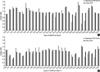
Table 1
Clinico-pathological data and array CGH results of 28 gastric adenocarcinomas
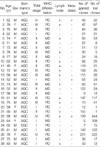
M, male; F, female; EGC, early gastric cancer; AGC, advanced gastric cancer; WD, well differentiated; MD, moderately differentiated; PD, poorly differentiated. Alterations were defined by log 2 ratio thresholds of 0.25 for copy number gain, -0.25 for loss.
*Gained clone No., cases with copy number gain; †Lost clone No., cases with copy number lost.
Table 2
Most frequently gained regions detected by microarray comparative genomic hybridization in 28 gastric adenocarcinomas, together with candidate genes
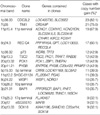
References
1. Takada H, Imoto I, Tsuda H, Sonoda I, Ichikura T, Mochizuki H, Okanoue T, Inazawa J. Screening of DNA copy-number aberrations in gastric cancer cell lines by array-based comparative genomic hybridization. Cancer Sci. 2005. 96:100–110.


2. Kimura Y, Noguchi T, Kawahara K, Kashima K, Daa T, Yokoyama S. Genetic alterations in 102 primary gastric cancers by comparative genomic hybridization: gain of 20q and loss of 18q are associated with tumor progression. Mod Pathol. 2004. 17:1328–1337.


3. Koo SH, Kwon KC, Shin SY, Jeon YM, Park JW, Kim SH, Noh SM. Genetic alterations of gastric cancer: comparative genomic hybridization and fluorescence in situ hybridization studies. Cancer Genet Cytogenet. 2000. 117:97–103.
4. Koizumi Y, Tanaka S, Mou R, Koganei H, Kokawa A, Kitamura R, Yamauchi H, Ookubo K, Saito T, Tominaga S, Matsumura K, Shimada H, Tsuchida N, Sekihara H. Changes in DNA copy number in primary gastric carcinomas by comparative genomic hybridization. Clin Cancer Res. 1997. 3:1067–1076.
5. Okada K, Sugihara H, Bamba M, Bamba T, Hattori T. Sequential numerical changes of chromosomes 7 and 18 in diffuse-type stomach cancer cell lines: combined comparative genomic hybridization, fluorescence in situ hybridization, and ploidy analyses. Cancer Genet Cytogenet. 2000. 118:99–107.
6. Wu MS, Chang MC, Huang SP, Tseng CC, Sheu JC, Lin YW, Shun CT, Lin MT, Lin JT. Correlation of histologic subtypes and replication error phenotype with comparative genomic hybridization in gastric cancer. Genes Chromosomes Cancer. 2001. 30:80–86.


7. Kallioniemi A, Kallioniemi OP, Sudar D, Rutovitz D, Gray JW, Waldman F, Pinkel D. Comparative genomic hybridization for molecular cytogenetic analysis of solid tumors. Science. 1992. 258:818–821.


8. Weiss MM, Kuipers EJ, Postma C, Snijders AM, Pinkel D, Meuwissen SG, Albertson D, Meijer GA. Genomic alterations in primary gastric adenocarcinomas correlate with clinicopathological characteristics and survival. Cell Oncol. 2004. 26:307–317.
9. Wilhelm M, Veltman JA, Olshen AB, Jain AN, Moore DH, Presti JC Jr, Kovacs G, Waldman FM. Array-based comparative genomic hybridization for the differential diagnosis of renal cell cancer. Cancer Res. 2002. 62:957–960.
10. Man TK, Lu XY, Jaeweon K, Perlaky L, Harris CP, Shah S, Ladanyi M, Gorlick R, Lau CC, Rao PH. Genome-wide array comparative genomic hybridization analysis reveals distinct amplifications in osteosarcoma. BMC Cancer. 2004. 4:45.


11. Peng DF, Sugihara H, Mukaisho K, Tsubosa Y, Hattori T. Alterations of chromosomal copy number during progression of diffuse-type gastric carcinomas: metaphase-and array-based comparative genomic hybridization analyses of multiple samples from individual tumours. J Pathol. 2003. 201:439–450.
12. Kawaguchi K, Honda M, Yamashita T, Shirota Y, Kaneko S. Differential gene alteration among hepatoma cell lines demonstrated by cDNA microarray-based comparative genomic hybridization. Biochem Biophys Res Commun. 2005. 329:370–380.


13. Albertson DG, Pinkel D. Genomic microarrays in human genetic disease and cancer. Hum Mol Genet. 2003. 12:145–152.


14. Frijters AC, Zhang JZ, van Damme M, Wang GL, Ronald PC, Michelmore RW. Construction of a bacterial artificial chromosome library containing large Eco RI and Hin dIII genomic fragments of lettuce. Theor Appl Genet. 1997. 94:390–399.


15. Pinkel D, Segraves R, Sudar D, Clark S, Poole I, Kowbel D, Collins C, Kuo WL, Chen C, Zhai Y, Dairkee SH, Ljung BM, Gray JW, Albertson DG. High resolution analysis of DNA copy number variation using comparative genomic hybridization to microarrays. Nat Genet. 1998. 20:207–211.


16. Shaw CJ, Shaw CA, Yu W, Stankiewicz P, White LD, Beaudet AL, Lupski JR. Comparative genomic hybridisation using a proximal 17p BAC/PAC array detects rearrangements responsible for four genomic disorders. J Med Genet. 2004. 41:113–119.


17. Veltman JA, Fridlyand J, Pejavar S, Olshen AB, Korkola JE, DeVries S, Carroll P, Kuo WL, Pinkel D, Albertson D, Cordon-Cardo C, Jain AN, Waldman FM. Array-based comparative genomic hybridization for genome-wide screening of DNA copy number in bladder tumors. Cancer Res. 2003. 63:2872–2880.
18. Schneider-Stock R, Boltze C, Lasota J, Miettinen M, Peters B, Pross M, Roessner A. High prognostic value of p16INK4 alterations in gastrointestinal stromal tumors. J Clin Oncol. 2003. 21:1688–1697.


19. Kang MJ, Park BJ, Byun DS, Park JI, Kim HJ, Park JH, Chi SG. Loss of imprinting and elevated expression of wild-type p73 in human gastric adenocarcinoma. Clin Cancer Res. 2000. 6:1767–1771.
20. Chan WY, Liu Y, Li CY, Ng EK, Chow JH, Li KK, Chung SC. Recurrent genomic aberrations in gastric carcinomas associated with Helicobacter pylori and Epstein-Barr virus. Diagn Mol Pathol. 2002. 11:127–134.


21. Derre J, Lagace R, Terrier P, Sastre X, Aurias A. Consistent DNA losses on the short arm of chromosome 1 in a series of malignant gastrointestinal stromal tumors. Cancer Genet Cytogent. 2001. 127:30–33.
22. O'Leary T, Ernst S, Przygodzki R, Emory T, Sobin L. Loss of heterozygosity at 1p36 predicts poor prognosis in gastrointestinal stromal/smooth muscle tumors. Lab Invest. 1999. 79:1461–1467.
23. Hodgson JG, Chin K, Collins C, Gray JW. Genome amplification of chromosome 20 in breast cancer. Breast Cancer Res Treat. 2003. 78:337–345.


24. Wang Q, Wang B, Guan X, Gao H, Cheng H, Zhang Q, Huang C, Li P. Analysis of comparative genomic hybridization and loss of heterozygosity in 43 primary gastric carcinomas. Chin Med J. 2003. 116:517–523.
25. Rumpel CA, Powell SM. Mapping of genetic deletions on the long arm of chromosome 4 in human esophageal adenocarcinomas. Am J Pathol. 1999. 154:1329–1334.


26. Yan WS, Song LY, Wei WD, Li A, Liang QW, Liu JH, Fang Y. Chromosomal imbalance in primary lung squamous cell carcinoma and their relationship with smoking. Ai Zheng. 2005. 24:47–52.
27. Kallio JP, Mahlamaki EH, Helin H, Karhu R, Kellokumpu-Lehtinen P, Tammela TL. Chromosomal gains and losses detected by comparative genomic hybridization and proliferation activity in renal cell carcinoma. Scand J Urol Nephrol. 2004. 38:225–230.
28. Jones C, Ford E, Gillett C, Ryder K, Merrett S, Reis-Filho JS, Fulford LG, Hanby A, Lakhani SR. Molecular cytogenetic identification of subgroups of grade III invasive ductal breast carcinomas with different clinical outcomes. Clin Cancer Res. 2004. 10:5988–5997.


29. Van Dekken H, Geelen E, Dinjens WN, Wijnhoven BP, Tilanus HW, Tanke HJ, Rosenberg C. Comparative genomic hybridization of cancer of the gastroesophageal junction: deletion of 14Q31-32. 1 discriminates between esophageal (Barrett's) and gastric cardia adenocarcinomas. Cancer Res. 1999. 59:748–752.
30. Koo SH, Jeong TE, Kang J, Kwon KC, Park JW, Noh SM. Prognostic implications for gastric carcinoma based on loss of heterozygosity genotypes correlation with clinicopathologic variables. Cancer Genet Cytogenet. 2004. 153:26–31.
31. Nishizuka S, Tamura G, Terashima M, Satodate R. Loss of heterozygosity during the development and progression of differentiated adenocarcinoma of the stomach. J Pathol. 2000. 185:38–43.

