Abstract
Diabetic nephropathy is characterized by an expansion of the glomerular mesangium, caused by mesangial cell proliferation and an excessive accumulation of extracellar matrix (ECM) proteins, which eventually leading to glomerulosclerosis. TGF-β1 was found to play an important role in the accumulation of ECM in the kidney. In this study, TGF-β1 RNA interference was used as an effective therapeutic strategy. The inhibitory effect of TGF-β1 small interfering RNAs (siRNAs) on the high glucose-induced overexpression of TGF-β1 in rat mesangial ceys (RMCs). A high levels of glucose induces TGF-β1 mRNA and protein, and TGF-β1 siRNAs reduce the ability of high glucose to stimulate their expression. We also examined the inhibitory effect of TGF-β1 siRNAs on the expression of plasminogen activator inhibitor (PAI)-1 and Collagen Type I which are down-regulators of TGF-β1. The expression of TGF-β1, PAI-1 and Collagen Type I was increased in RMCs that were stimulated by 30 mM glucose. TGF-β1 siRNAs reduces high glucose-induced TGF-β1, PAI-1, and Collagen Type I mRNA and protein expression in a dose-dependent manner. In conclusion, the present study demonstrates that TGF-β1 siRNAs effectively inhibits TGF-β1 mRNA and protein expression in RMCs. These suggest that TGF-β1 siRNAs through RNAi may be a useful tool for developing new therapeutic applications for the treatment of diabetic nephropathy.
Diabetic nephropathy is characterized by an expansion of the glomerular mesangium, caused by mesangial cell proliferation and an excessive accumulation of extracellar matrix (ECM) proteins, eventually leading to glomerulosclerosis and renal failure (1,2). Glomerulosclerosis is one of the complications associated with diabetes. Mesangial expansion caused by cell proliferation and glomerular ECM accumulation is one of the earliest renal abnormalities observed at the onset of hyperglycemia in diabetes mellitus (3). Because hyperglycemia is assumed to trigger the glomerulosclerosis that results from an imbalance in the control of glomerular mesangial cells proliferation (4), glomerular mesangial cells cultured in high glucose medium have been used as a model to study unbalanced gene expression in diabetes (5). High levels of glucose induce the production of transforming growth factor (TGF)-β1, plasminogen activator inhibitor (PAI)-1, collagen, fibronectin, protein kinase C, collagen, matrix metalloprotein-2. It also increases the activities of transcription factors such as NF-κB, which regulate the transcription of genes that are involved in glomerulosclerosis (6-8). Investigations of glomerular mesangial cells and proximal tubularcells have demonstrated that high glucose media induces the enhanced expression of TGF-β1 mRNA and protein, the biosynthesis of collagen and other ECM constituents (9-11).
TGF-β1 is a multifunctional cytokine that plays an important role in wound healing, cell proliferation, differentiation, apoptosis, immune response and ECM remodeling. TGF-β1 is a critical cytokine involved in diabetes-induced ECM in both cell cultures and animal models of diabetic kidney disease. TGF-β1 plays a central role in tissue repair by stimulating the balanced expression of ECM such as collagen, fibronectin and matrix proteoglycans. Thus suppression of TGF-β1 could be an effective strategy for the prevention of glomerulosclerosis.
RNAi, a process of sequence-specific gene suppression is a known as a natural gene regulatory mechanism. Small interfering RNAs (siRNAs) mediate RNAi, a process in which target mRNAs are degraded. It has recently been reported that siRNAs technology can be used to produce post-transcriptional gene silencing in mammalian cells. The mechanisms of RNAi have become more defined. When dsRNAs are introduced into an organism, the sequence-specific destruction of endogenous target mRNAs occurs. This process involves the cleavage of dsRNAs into active 21-23 nucleotide (nt) length siRNAs by Dicer (12) or another RNase III-like enzyme (13). These siRNAs are incorporated into a RNAi-induced silencing complex (14) and are then used as a guide to degrade the corresponding mRNA (15).
The aim of the present study was to investigate the effect of TGF-β1 siRNAs on the high glucose-induced overexpression of TGF-β1 in the rat mesangial cells (RMCs). We also examined the inhibitory effect of TGF-β1 siRNAs on the expression of PAI-1 and Collagen Type I which are down-regulators of TGF-β1. We applied the TGF-β1 siRNAs strategy to suppress the proliferation and gene expression of RMCs induced by high glucose levels.
The selection of TGF-β1 siRNAs for TGF-β1 was performed using the GenBank database BLAST program. The sequences of siRNAs against rat TGF-β1 mRNA (GenBank, accession TGF-β1 site NM_021578) are as follows:
5'AACCAAGGAGACGGAATACAGttcaagagaCTGTATTCCGTCTCCTTGGTTTTTTT-3'. TGF-β1 siRNAs sequences 9-mer insert of hairpin construction with siRNAs consisting of 21-mer sense and antisense. The DNA fragment was digested with EcoRI and XbaI, and then cloned into the EcoRI and XbaI site of pUC-U6-extra. The 21-mer TGF-β1 siRNAs were custom-synthesized by BIONEER(Seoul, Korea).
RMCs were obtained from a culture of glomeruli isolated from male Sprague-Dawley (SD) rats weighing 100 to 150 g using differential sieving methods as described previously (16). Isolated glomeruli were cultured in RPMI 1640 (GIBCO, Grand Island, NY, U.S.A.) complete medium containing 100 U/mL penicillin, 100 µg/mL streptomycin, 0.25 µg/mL amphotericin B, 10 mg/mL insulin, 6.7µg/L sodium selenite, 5.5 mg/L transferrin, and 20% heat-inactivated fetal calf serum (FCS; South Logan, UT, U.S.A.) at 37℃ in a humidified 5% CO2atmosphere. The identity of the RMCs were confirmed by phase contrast microscopy according to the morphologic criteria (stellate-shaped cell bodies with irregular cytoplasmic projections (17), RMCs from passage 10-15 were used in the cell studies.
RMCs were seeded at 2×104cells/well in 2-well chamber slides and cultured in RPMI 1640 complete medium for and cultured in RPMI 1640 complete medium for 24 hr. The cells were serum-starved for 24 hr in RPMI 1640 containing 0.5% FBS and transfected with fluorescein-labeled-TGF-β1 siRNAs. TGF-β1 siRNAs were labeled using the Label IT Fluorescein Nucleic Acid Labeling Kit (Panvera, Madison, WI, U.S.A.) were used to facilitate the transfection of TGF-β1 siRNAs into RMCs. Briefly, 0.5 µg of TGF-β1 siRNAs in DMEM (GIBCO, Grand Island, NY, U.S.A.) were mixed with the Plus reagent and incubated at room temperature for 15 min. The siRNAs were mixed with 2µg of Lipofectamine Pluse Reagent and subsequently incubated at room temperature for 15 min. The cells were washed with serum-free DMEM and 0.03 mL/well of serum-free DMEM was then added to the cells. The siRNAs-liposome complexes were added drop by drop to each well and incubated at 37℃ for 24 hr. Finally, the RMCs were washed three times with phosphate buffered saline (PBS) and fixed in methanol at 4℃ for 10 min. The cells were mounted and observed through a fluorescence microscope. The effect of TGF-β1 siRNAs on TGF-β1 mRNA expression in RMCs was investigated.
The cells were lysed in buffer (50 mM Tris, 150 mM NaCl, 5 mM EDTA, 0.5% NP-40, 100 mM PMSF, 1 M DTT, 10 g leupeptin, 10 g aprotinin (Sigma, St. Louis, MO, U.S.A.) and centrifuged at 12,000 g for 10 min after storing them on ice for 30 min. The protein content of the supernatants was determined using the Bradford protein assay (BioRad, Hercules, CA, U.S.A.) and boiled with 4×SDS sample buffer for 10 min. 10 g of protein was loaded in each well of 8-12% SDS-PAGE and electrophoresis was then performed at 15 V for 12 hr. The proteins were transferred onto a nitrocellulose filter and probed with TGF-β1, PAI-1 antibodies (Santa Cruz Biotechnology, Santa Cruz, CA, U.S.A.), Collagen Type I (Oncogene, Cambridge, MA, U.S.A.) followed by a secondary antibody conjugated to horseradish peroxidase and detected with enhanced chemiluminescence reagents (Amersham, Piscataway, NJ, U.S.A.).
Total RNA was extracted from cultured RMCs with RNAzol B (TEL-TEST, Friendswood, TX, U.S.A.) according to the manufacturer's instructions. The purity and quantity of the RNA preparation were determind by measuring the optical densities at 260 and 280 nm. Total RNA was reverse transcribed with oligo-d (T) primers and M-MLV reverse transcriptase (Promega, Madison, WI, U.S.A.). cDNA was amplified by PCR using primer sets specific to rat TGF-β1, PAI-1, Collagen Type I and a constitutively expressed house keeping gene, glyceraldehydes-3-phosphate-dehydrogenase (GAPDH) as the control. The primers used for PCR areas follows:
Rat TGF-β1 sense 5'-CCTGCTGCTTTCTCCCTCAACC-3'
antisense 5'-CTGGCACTGCTTCCCGAAATGTC-3'
Rat PAI-1 sense 5'-CACGAGTACGACATCCTGGAA-3'
antisense 5'-CCCTCTGAGCTCCACTTCAGT-3'
Rat Collagen Type I sense 5'-CTGACTGGAAGAGCGGAGAG-3
antisense 5'-TGAGTTTGGGTTGTTGGTCT-3'
Rat GAPDH sense 5'-GTGGACATTGTTGCCATCAACG-3'
antisense 5'-GAGGGAGTTGTCATATTTCTCG-3'
The reaction was run for 30 cycles with a cycling parameter of 50 sec at 95℃, 60 sec at (TGF-β1: 60℃, PAI-1: 56℃ and Collagen Type I: 50℃), 60 sec at 72℃ PCR products were visualized on a 1% agarose gel. The amounts of TGF-β1, PAI-1 and Collagen Type I mRNA were measured by densitometry and expressed relative to the densities of GAPDH.
RMCs cultured in glucose at a normal concentration (5.5 mM) were removed from the culture flasks by incubation with PBS containing 0.25% trypsin and 5 mM EDTA. Equal numbers of cells were seeded on tissue culture plates. At 20-50% confluence, the RMCs were rendered quiescent by incubation for 24 hr in growth medium deficient in insulin and serum. To evaluate the effect of TGF-β1 siRNAs on RMC proliferation, Lipofectamin Plus Reagent: TGF-β1 siRNAs (100 nM) was added to the wells, and the cells were incubated at 37℃ for an additional 5 hr. After incubation, the cells were exposed to medium containing 1% serum and normal glucose (5.5 mM) or high glucose (30 mM). The index of cell proliferation was determined with a WST cell counting kit (Wako, Osaka, Japan) after 48 hr.
For the induction of TGF-β1 expression by high glucose mesangial cells were exposed to 5.5 mM glucose, 20 mM glucose, 30 mM glucose and 40 mM glucose in DMEM media for 24 hr. TGF-β1 mRNA expression was determined by RT-PCR. The expression level of TGF-β1 increased by about 1.8 fold in 30 mM glucose (Fig. 1).
To determine the transfection efficiency of TGF-β1 siRNAs, fluorescein-labeled-TGF-siRNAs were transfected to RMCs. The cells were then evaluated by fluorescence microscopy. Most of the RMCs exhibited strong fluorescence in the cytoplasm and nucleus (Fig. 2).
To examine the possibility of dose dependence in the suppression effects of TGF-β1 siRNAs (2, 4, and 8 µg) on the protein expression of TGF-β1, PAI-1 and Collagen Type I, they were transfected into primary cultured RMCs. Translational expression was confirmed by Western blot analysis (Fig. 3A) and the result were measured by means of an LAS-3000 image analyzer (Fig. 3B). Intracellular TGF-β1, PAI-1 and Collagen Type I protein expressions were significantlyincreased by about 1.6, 2.1, 1.6 fold by high levels of glucose. Compared with the high glucose, transfection of TGF-β1 siRNAs (2, 4, and 8 µg) reduced TGF-β1, PAI-1 and Collagen Type I protein levels in a dose-dependant manner. In addition, the expression was significantly inhibited in cells treated with TGF-β1 siRNAs (8µg).
To examine the possibility of dose dependence on the suppression effects of TGF-β1 siRNAs (1, 2, and 4 µg) on the mRNA expression of TGF-β1, they were transfected into primary cultured RMCs. Total RNA was isolated from the transfected cells and RT-PCR was performed (Fig. 4A) and the result were measured by means of an LAS-3000 image analyzer (Fig. 4B). Intracellular TGF-β1, PAI-1 and Collagen Type I mRNA expressions were significantly increased by about 1.5, 1.5 and 1.5 fold by high glucose levels. Compared with the high glucose and the transfection of TGF-β1 siRNAs (1, 2, and 4 µg) TGF-β1, PAI-1 and Collagen Type I mRNA levels were reduced in a dose-dependant manner. In addition, the expression was significantly inhibited in TGF-β1 siRNAs (4 µg) treated .
RMCs transfected with TGF-β1 siRNAs were cultured in medium containing normal glucose or high glucose for 24 hr, and the proliferation was determined by the WST-1 test (Fig. 5). To examine the possibility of dose dependence on the suppression effects of TGF-β1 siRNAs (0.1, 0.2 and 0.4 µg) on the cell growth. They were transfected into primary cultured RMCs. Cell growth were significantly increased by about 2.1 fold by high glucose levels. Compared with the high glucose and the transfection of TGF-β1 siRNAs (0.1, 0.2 and 0.4 µg) cell growth were reduced in a dose-dependant manner. In addition, the expression was significantly inhibited in TGF-β1 siRNAs (0.4 µg) treated.
Diabetic nephropathy is characterized by an expansion of the glomerular mesangium, caused by mesangial cell proliferation and an excessive accumulation of ECM proteins, eventually leading to glomerulosclerosis and renal failure. The manifestation of diabetic nephropathy may be a consequence of the actions of certain cytokines and growth factors. Prominent among these is TGF-β1 because it promotes mesangial cell expansion and stimulates ECM accumulation, in diabetic renal disease (18).
TGF-β1 has been implicated as an etiologic cause in its progression. TGF-β1 is a key mediator of the hyperglycemia-induced panoramic effects on cell growth and ECM (19-21). In addition, this cytokine has powerful fibrogenic potential because of its capability for simultaneous actions such as the stimulation of matrix synthesis, the inhibition of matrix degradation, and the modulation of matrix receptor expression to facilitate cell matrix interactions (22). High levels of glucose increases TGF-β1 expression, and the cell expansion and matrix-stimulatory effects of high glucose are prevented by anti-TGF-β1 therapy. Thus, TGF-β1 is considered to be a therapeutic target in diabetic nephropathy and other chronic kidney diseases, and anti-TGF-β1 therapies have also been performed (23-25).
The aim of gene therapy is to replace defective genes in target tissue by delivering correct versions of them in the form of an expression vector. Recent progress in molecular biology has provided new techniques to inhibit target gene expression. Gene therapies have been attempted by antisense ODN, decoy, ribozyme, and RNAi. More recently, gene therapy using RNAi as one of the anti-TGF-β1 therapies has been investigated and have been applied to renal disease.
The TGF-β1 expression was increased by 1.8 fold in RMCs stimulated by 30 mM glucose. In addition, they effectively inhibited the expression of TGF-β1 mRNA and protein in RMCs stimulated by 30 mM glucose. These results are consistent with the notion that the transfection of TGF-β1 siRNAs siRNAs is effective in reducing TGF-β1 expression. The purpose of this study was to evaluate the efficiency of TGF-β1 siRNAs, to accomplish this fluorescein-labeled-TGF-β1 siRNAs were introduced into RMCs using the Mirus Transfer IT. Fluorescence microscopy of RMCs that had been transfected with Fluorescein- labeled-TGF-β1 siRNAs were observed in both the nuclei and the cytoplasm. The effect of TGF-β1 siRNAs on the endogenous gene expression of PAI-1 and Collagen Type I was also examined in RMCs. High glucose levels significantly stimulates RMCs to increase the endogenous translation of PAI-1 and Collagen Type I. The transfection of RMCs with the TGF-β1 siRNAs resulted in a significant attenuation of high glucose stimulation-induced gene expression. This suggests that TGF-β1 siRNAs effectively suppress the translation of endogenous genes that are involved in ECM formation. High glucose levels significantly stimulate RMCs to increase the endogenous transcription of PAI-1 and Collagen Type I. The transfection of RMCs with the TGF-β1 siRNAs resulted in a significant attenuation of high glucose stimulation-induced gene expression. This suggests that TGF-β1 siRNAs effectively suppress the transcription of endogenous genes that are involved in ECM formation (26-28).
To evaluate the influence of hyperglycemia on the proliferation of primary RMCs, we investigated the ability of TGF-β1 siRNAs to inhibit cell growth. High levels of glucose significantly stimulated the growth of primary RMCs. The transfection of RMCs with TGF-β1 siRNAs resulted in a significant suppression of the cell growth stimulated by high glucose. These results suggest that TGF-β1 siRNAs effectively suppresses the proliferation of RMCs, as well as the expression of genes that are involved in the accumulation of ECM proteins.
In summary, in this study, we applied TGF-β1 siRNAs strategy to suppress TGF-β1 overexpression the cell proliferation of RMCs induced by a high glucose concentrations. The suppressive effect of TGF-β1 siRNAs was also applied to the expression of PAI-1 and Collagen Type I which are down-regulators of TGF-β1. Our results demonstrate that TGF-β1 siRNAs efficiently suppressed the expression of mesangium matrix component genes and inhibited high glucose-induced mesangial cell proliferation. This siRNAs mechanism invented by nature to protect the genome provides us with ideal tools to analyse gene function and for targeting any transcript in any cell with a high efficiency and specificity. These findings suggest that TGF-β1 siRNAs may be a useful tool for developing new therapeutic applications for the prevention of progressive diabetic nephropathy.
Figures and Tables
Fig. 1
TGF-β1 stimulated by 5.5, 20, 30 and 40 mM glucose in RMCs. After incubation of RMCs with control (5.5 mM), high (20 mM), high (30 mM), high (40 mM) glucose for 24 hr, total RNA was isolated, reverse transcripted and amplified by the polymerase chain reaction (A). TGF-β1 levels for each conditions were measured by means of an LAS-3000 image analyzer (B). The expression level of TGF-β1 increases in 30 mM high glucose.
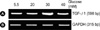
Fig. 2
Fluorescence microscopy photograph of RMCs transfected with TGF-β1 siRNAs in cationic liposomes. The fluorescein labeled TGF-β1 siRNAs are transfected to RMCs (×200).
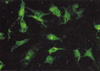
Fig. 3
The effect of TGF-β1 siRNAs on high glucose induced TGF-β1, PAI-1 and Collagen Type I protein upregulation in RMCs (Western blot).
(A) Lane 1: control (5.5 mM), Lane 2: stimulated with high glucose (30 mM), Lane 3: 2 µg/µL TGF-β1 siRNAs transfection plus high glucose (30 mM) stimulation for 24 hr. Lane 4: 4µg/µL TGF-β1 siRNAs transfection plus high glucose (30 mM) stimulation for 24 hr. Lane 5: 8 µg/µL TGF-β1 siRNAs transfection plus high glucose (30 mM) stimulation for 24 hr. (B) Values are presented as means ±SEM of triplicate experiments.*p<0.05 compared to normal glucose;†p<0.05 compared to high glucose.
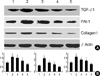
Fig. 4
The effect of TGF-β1 siRNAs on the high glucose-induced mRNA expression of TGF-β1, PAI-1 and Collagen Type I. RMCs transfected with TGF-β1 siRNAs were cultured in medium containing 5.5 or 30 mM glucose for 24 hr and total RNA was extracted from the RMCs. mRNA expression of TGF-β1, PAI-1 and Collagen Type I was determined by RT-PCR (A). TGF-β1, PAI-1 and Collagen Type I levels for each condition was measured by means of an LAS-3000 image analyzer (B).
Lane 1: control (5.5 mM), Lane 2: stimulated with high glucose (30 mM), Lane 3: 1 µg/µL TGF-β1 siRNAs transfection plus high glucose (30 mM) stimulation for 24 hr. Lane 4: 2 µg/µL TGF-β1 siRNAs transfection plus high glucose (30 mM) stimulation for 24 hr. Lane 5: 4 µg/µL TGF-β1 siRNAs transfection plus high glucose (30 mM) stimulation for 24 hr.
Values are presented as means±SEM of triplicate experiments.
*p<0.05 compared to normal glucose;†p<0.05 compared to high glucose.
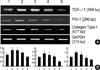
Fig. 5
TGF-β1 siRNAs suppress high glucose-induced RMC proliferation. RMCs transfected with TGF-β1 siRNAs were cultured in medium containing normal glucose or high glucose for 24 hr, and the proliferation was determined by the WST-1 test.
N: control (5.5 mM), H: stimulated with high glucose (30 mM), 0.5X: 0.1µg/µL TGF-β1 siRNAs transfection plus high glucose (30 mM) stimulation for 24 hr. 1X: 0.2 µg/µL TGF-β1 siRNAs transfection plus high glucose (30 mM) stimulation for 24 hr. 2X: 0.4 µg/µL TGF-β1 siRNAs transfection plus high glucose (30 mM) stimulationfor 24 hr.
Values are presented as means±SEM of triplicate experiments.
*p<0.05 compared to normal glucose;†p<0.05 compared to high glucose.
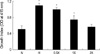
References
1. Wolf G, Ziyadeh FN. Molecular mechanisms of diabetic renal hypertrophy. Kidney Int. 1999. 56:393–405.


2. Steffes MW, Bilous RW, Sutherland DE, Mauer SM. Cell and matrix components of the glomerular mesangium in type I diabetes. Diabetes. 1992. 41:679–684.


3. Klahr S, Schreiner G, Ichikawa I. The progression of renal disease. N Engl J Med. 1988. 318:1657–1666.


4. Jensen T. Pathogenesis of diabetic vascular disease; evidence for the role of reduced heparan sulfate proteoglycan. Diabetes. 1997. 2:98–100.


5. Grishok A, Mello CC. RNAi (Nematodes: Caenorhabditis elegans). Adv Genet. 2002. 46:339–360.
6. Ziyadeh FN, Sharma K, Ericksen M, Wolf G. Stimulation of collagen gene expression and protein synthesis in murine mesangial cells by high glucose is mediated by autocrine activation of transforming growth factor-beta. J Clin Invest. 1994. 93:536–542.


7. Nahman NS Jr, Leonhart KL, Cosio FG, Herbert CL. Effects of high glucose on cellular proliferation and fibronectin production by cultured human mesangial cells. Kidney Int. 1992. 41:396–402.


8. Koya D, Jirousek MR, Lin YW, Ishii H, Kuboki K, King GL. Characterization of protein kinase C beta isoform activation on the gene expression of transforming growth factor-beta, extracellular matrix components, and prostanoids in the glomeruli of diabetic rats. J Clin Invest. 1997. 100:115–126.


9. Wolf G, Sharma K, Chen Y, Ericksen M, Ziyadeh FN. High glucose-induced proliferation in mesangial cells is reversed by autocrine TGF-β1. Kidney Int. 1992. 42:647–656.
10. Ziyadeh FN, Sharma K, Ericksen M, Wolf G. Stimulation of collagen gene expression and protein synthesis in murine mesangial cells by high glucose is mediated by autocrine activation of transforming growth factor-β. J Clin Invest. 1994. 93:536–542.
11. Hoffman BB, Sharma K, Zhu Y, Ziyadeh FN. Transcriptional activation of transforming growth factor-β1 in mesangial cell culture by high glucose concentration. Kidney Int. 1998. 54:1107–1116.


12. Zamore PD, Tuschl , Sharp PA, Bartel DP. RNAi: double-stranded RNA directs the ATP-dependent cleavage of mRNA at 21 to 23 nucleotide intervals. Cell. 2000. 101:25–33.
13. Bernstein E, Caudy AA, Hammond SM, Hannon GJ. Role for a bidentate ribonuclease in the initiation step of RNA interference. Nature. 2001. 409:363–366.


14. Hammond SM, Boettcher S, Caudy AA, Kobayashi R, Hannon GJ. Argonaute2, a link between genetic and biochemical analyses of RNAi. Science. 2001. 293:1146–1150.


15. Elbashir SM, Harborth J, Weber K, Tuschl T. Analysis of gene function in somatic mammalian cells using small interfering RNAs. Methods. 2002. 26:199–213.


16. Leehey DJ, Song RH, Alari N, Singh AK. Decreased degradative enzymes in mesangial cells cultured in high glucose media. Diabetes. 1995. 44:929–935.


17. Kaname S, Uchida S, Ogata E, Kurokawa K. Autocrine secretion of transforming growth factor-β in cultured rat mesangial cells. Kidney Int. 1992. 42:1319–1327.


18. Chen S, Jim B, Ziyadeh FN. Diabetic nephropathy and transforming growth factor-beta: transforming our view of glomerulosclerosis and fibrosis build-up. Semin Nephrol. 2003. 6:532–543.
19. Oh JH, Ha HJ, Yu MR, Lee HB. Sequential effects of high glucose on mesangial cell transforming growth factor-β1 and fibronectin synthesis. Kidney Int. 1998. 54:1872–1878.


20. Park IS, Kiyomoto H, Abboud SL, Abboud HE. Expression of transforming growth factor-β and type IV collagen in early streptozotocin-induced diabetes. Diabetes. 1997. 46:473–480.


21. Hill C, Flyvbjerg A, Gronbaek H, Petrik J, Hill DJ, Thomas CR, Sheppard MC, Logan A. The renal expression of transforming growth factor-β isoforms and their receptors in acute and chronic experimental diabetes in rats. Endocrinology. 2000. 141:1196–1208.


22. Border WA, Nobel NA. Transforming growth factor β in tissue in tissue fibrosis. N Engl J Med. 1994. 331:1286–1292.
24. Border WA, Noble NA. TGF-β in kidney fibrosis: a target for gene therapy. Kidney Int. 1997. 51:1388–1396.


25. Fire A, Xu S, Montgomery MK, Kostas SA, Driver SE, Mello CC. Potent and specific genetic interference by double-stranded RNA in Caenorhabditis elegans. Nature. 1998. 391:806–811.


26. Roberts AB, Sporn MB, Assoian RK, Smith JM, Roche NS, Wakefield LM, Heine UI, Liotta LA, Falanga V, Kehrsl JH, Fauci AS. Transforming growth factor type β: rapid induction of fibrosis and angiogenesis in vivo and stimulation of collagen formation in vitro. Proc Natl Acad Sci USA. 1986. 83:4167–4171.