Abstract
This study was done to determine the neuroprotective effect of cycloheximide on neonatal hypoxic-ischemic brain injury. Seven day-old newborn rat pups were subjected to 90 min of 8% oxygen following a unilateral carotid artery ligation. The extent of cerebral infarction was evaluated at 1 and 4 week of recovery. Apoptosis was identified by performing terminal deoxynucleotidyl transferase-mediated dUTP nick end-labeling (TUNEL) staining and flow cytometry with a combination of fluoresceinated annexin V and propidium iodide. Brain infarction area was significantly increased at 4 week compared to 1 week after hypoxia-ischemia in the control group. With cycloheximide treatment, the number of TUNEL positive cells in the ipsilateral cerebral cortex at 48 hr and peri-infarct area at 1 and 4 week of recovery was significantly reduced, both apoptotic and necrotic cells by flow cytometry 48 hr after the injury were significantly reduced, and the extent of cerebral infarction at 1 and 4 week of recovery was also significantly attenuated compared to the hypoxia-ischemia control group. In summary, our data suggest that apoptosis plays an important role in the development of delayed infarction, and inhibition of apoptosis with cycloheximide significantly reduces the ensuing cerebral infarction in a newborn rat pup model of cerebral hypoxia-ischemia.
Perinatal hypoxic-ischemic brain injury is an important cause of neonatal mortality and permanent neurologic sequelae such as cerebral palsy, mental retardation, learning disability, and epilepsy in survivors (1, 2). In spite of increased understanding of the mechanisms of cell death underlying neonatal hypoxic-ischemic brain injury, there is no clinically efficacious treatment available for this common disorder. Therefore, the development of new therapeutic modality to improve the prognosis of this disease is an urgent big subject.
Two major forms of cell death, apoptosis and necrosis, can be distinguished during hypoxic ischemic brain injury (3-6). Necrosis is characterized by prominent acute cell body swelling with subsequent cell lysis. Apoptosis is characterized by compaction of the cell body and internucleosomal DNA cleavage, and new protein synthesis appears to be required in many cases (7). The balance between modes of death may be influenced by maturational stage and severity of insult (5). In the adult brain, although classically hypoxic-ischemic cell death has been considered to occur by necrosis, recently clues have emerged suggesting that apoptosis may also contribute to neuronal loss (3, 8). In the developing newborn brain, apoptosis has been known to be the primary mode of cell death following hypoxia-ischemia (9). Various biochemical and morphologic evidence of apoptotic cell death has been reported in the hypoxic-ischemic immature brain under experimental conditions (5, 10-12) and in the human infant brain after birth asphyxia (13). It thus appears that therapies aimed at inhibiting neuronal apoptosis could ameliorate the hypoxicischemic brain injury. In accordance with this assumption, as apoptosis can require new protein synthesis (7), cycloheximide, a protein synthesis inhibitor, has been shown to prevent apoptosis in vitro (14), and to reduce neuronal cell loss and infarction volume in the adult animal model after cerebral ischemia (3, 8). Surprisingly, the neuroprotective effect of this agent has never been tested in the newborn animal model of cerebral hypoxia-ischemia, in which many neuronal cells die by apoptosis (5, 9-12).
This study was done to determine whether cycloheximide could reduce cerebral injury subsequent to hypoxia-ischemia in the developing brain. We tested the hypothesis that apoptosis plays an important role in the development of delayed brain injury, and inhibition of apoptosis with cycloheximide reduces the ensuing cerebral infarction in a newborn rat pup model of cerebral hypoxia-ischemia. Apoptosis was identified by performing terminal deoxynucleotidyl transferase-mediated dUTP nick end-labeling (TUNEL) staining and flow cytometry with a combination of fluoresceinated annexin V and propidium iodide (PI). The extent of cerebral infarction was evaluated at 1 and 4 week after hypoxia-ischemia to determine whether the infarction in the newborn brain occurs in a delayed fashion, and whether the neuroprotective effect, if any, of cycloheximide was transient or permanent.
The experimental protocols described herein were reviewed and approved by the animal care and use committee of the Samsung Biomedical Research Institute, Seoul Korea. This study also followed the institutional and National Institute of Health guidelines for laboratory animal care.
Unilateral carotid artery ligation was induced in 7-day-old Sprague Dawley rat pups (Daihan Biolink Co., Seoul, Korea) under methoxyflurane anesthesia. The neck was incised in the midline, and right common carotid artery was permanently ligated with 4-0 silk. Total time of surgery in each animal never exceeded 5 min. Following surgery, rats were returned to their mother for recovery and feeding for 2 hr. The pups were then exposed to a 90 min period of hypoxia (8% O2, 92% N2) by placing them in an airtight chamber partially submerged in a temperature controlled water bath to maintain the ambient temperature inside the chamber at a constant 36℃. In the hypoxia-ischemia with cycloheximide treatment group, the rat pups received an intraperitoneal injection of cycloheximide at a dose of 0.6 mg/kg immediately after the hypoxic insult (15) or equal volume of normal saline as a hypoxia-ischemia control group. Then, the rat pups were returned to their dam, and sacrificed at 48 hr, 1 and 4 week after hypoxia-ischemia under deep pentobarbital anesthesia (60 mg/kg, intraperitoneal), and the whole brain tissue was obtained for analysis. Ten animals, the minimum number required to achieve statistically meaningful results, were used in all subgroups of analyses.
After intracardiac perfusion with 4% paraformaldehyde, brains were removed, 5 µm thick serial sections were made, and stained with fluorescein in situ apoptosis detection kit S7110 (Chemicon International, Temecula, CA, U.S.A.) and counterstained with the 4,6-diamidino2-phenylindole (DAPI) to determine the degree of DNA fragmentation in cell nuclei at 48 hr after hypoxia-ischemia.
Brain infarction was determined by calculating mean brain areas of surviving tissue of three eosin stained serial sections from each brain at the level of the anterior commissure at 1 and 4 weeks after hypoxia-ischemia. Total brain areas of both contralateral and ipsilateral sides were measured using the Optimas 6.51 Image Analysis System (Media Cybernetics Inc., Silver Spring, MD, U.S.A.), and data are expressed as percentage of ipsilateral hypoxic-ischemic side compared to the contralateral undamaged side.
To delineate the extent of DNA fragmentation bordering the infarction area, the sections were attained with peroxidase in situ apoptosis detection kit S7100 (Chemicon International, Temecula, CA, U.S.A.) at 1 and 4 week after hypoxia-ischemia.
To evaluate the extent of apoptotic and necrotic cells, the midportion of ipsilateral cerebral cortex was dissociated into a single cell, and flow cytometry was done with a combination of PI (Sigma, St. Louis, MO, U.S.A.) and annexin V-FITC (fluorescein isothiocyanate) (Pharmingen, San Diego, CA, U.S.A.). The flow analysis was performed by a PAS (Particle analyzing System, Partec, Munster, Germany) equipped with an argon ion laser tuned at 488 nm wavelength. The green FITC-annexin V fluorescence was measured at 530±15 nm, and the red PI fluorescence was measured at 600 nm (16).
The percentage of normal brain area at the anterior commissure was significantly decreased at 4 week compared to 1 week after hypoxia-ischemia in the hypoxia-ischemia control group (73±27% vs. 51±31%) due to progression of infarction, and this decrease was significantly attenuated in the cycloheximide-treated group compared to the control group both 1 and 4 week after hypoxia-ischemia (93±7% vs. 82±17%) (Fig. 1A, 2).
The number of TUNEL positive cells in the ipsilateral cerebral cortex at 48 hr (112±11 vs. 24±3/high power field) (Fig. 3), and peri-infarct area 1 and 4 week after hypoxia-ischemia were also significantly reduced in the cycloheximide-treated group compared to the hypoxia-ischemia control group (Fig. 1B).
Representative analyses and regional percentage of an annexin V versus PI dot plot of ipsilateral cerebral cortex in hypoxia-ischemia control and cycloheximide-treated group at 48 hr after hypoxia-ischemia are presented in Fig. 4. In the cycloheximide-treated group, the percentage of necrotic and apoptotic cells in the respective area of Q2 (annexin V+/PI+) and Q4 (annexin V+/PI-) decreased, the live cells in Q3 (annexin V-/PI-) increased, and the damaged cells in Q1 (annexin V-/PI+) were not significantly different compared to the control group (Table 1).
In the present study, the area of cerebral infarction at 4 week was much larger than that at 1 week after the hypoxic-ischemic insult, indicating that newborn brain infarction can develop and progress in a very delayed fashion (17). These findings implicate that additional caution will be necessary for selecting a temporal endpoint for measurement of brain injury in a newborn rat pup model of cerebral hypoxia-ischemia. Conventional determination of the magnitude of infarction 1-2 week after injury would have grossly underestimated the damage. Moreover, these findings suggest the intriguing possibility that surprisingly delayed therapeutic interventions might prove to be valuable in reducing the brain tissue loss ultimately resulting from neonatal hypoxic-ischemic insults. Further studies will be necessary to clarify this.
In the present study, TUNEL staining was performed to look for evidence of nuclear DNA breakdown, a common feature of apoptosis (4, 18). Prominent TUNEL positive cells were observed in the ipsilateral cortex at 48 hr and in the periinfarct area at 1 and 4 week after hypoxia-ischemia. Furthermore, treatment with cycloheximide markedly reduced the number of TUNEL positive cells and the ensuing cerebral infarction, and this protective effect of cycloheximide was not transient. Thus our data point to the likelihood that apoptosis plays an important role in the development of delayed infarction (11, 12, 17), and protein synthesis inhibitors, as well as other anti-apoptotic strategies, may have therapeutic utility in a newborn rat pup model of hypoxia-ischemia even when given after the insult (3, 8). Although we did not verify here that cycloheximide indeed inhibited brain protein synthesis, our cycloheximide regimen has been known to inhibit forebrain protein synthesis in 7-day old rat pups (15). The reason why inhibition of protein synthesis should block apoptosis has not been completely understood, but presumably some critical protein must be synthesized de novo before apoptosis can proceed (7).
The absolute criteria for distinction between apoptosis and necrosis have not been delineated. As several recent studies have demonstrated that necrotic neurons can be TUNEL positive (4, 19), the detection of endonucleolytic DNA degradation cannot be a specific marker for apoptosis. Recently, flow cytometry has been established as a sensitive, rapid and objective method to distinguish the mode of cell death compared to the alternative methods such as morphological analysis (16, 20-24). Exposure of phosphatidylserine on the outside of the plasma membrane, the early and most characteristic change of apoptosis, can be detected by annexin V (20-24). The disruption of the plasma membrane and alterations in permeability, observed in necrotic cells, could be detected by staining with PI. Therefore, by combined staining of neuronal cells with both annexin V and PI as done in the present study, it was be possible to distinguish live cells, apoptotic cells, damaged cells, and necrotic cells by flow cytometry (16, 20-25).
As we hypothesized that cycloheximide would reduce only the apoptotic cell death by inhibiting active protein synthesis (7), our data of simultaneous inhibition of both apoptosis (annexin V+/PI-) and necrosis (annexin V+/PI+) with cycloheximide treatment observed by flow cytometry in the present study were rather unexpected. One possible explanation might be that the neuroprotective effect of cycloheximide might be mediated, at least in part, by a reduction in glutamate release and inhibition of the ensuing excitotoxic necrosis (26). Another possibility is that as some necrotic cells represent the secondary degradation of apoptotic cells, inhibition of apoptosis with cycloheximide might also attenuate the secondary necrosis (5, 21). Otherwise, the simple dichotomy of apoptosis or necrosis could not account for the complex mechanism of neuronal cell death (5, 6, 21), and the two mechanisms of neuronal death may be interrelated in a way yet to be defined (27). Further studies will be necessary to clarify this.
In conclusion, our data support the hypothesis that apoptosis plays an important role in the development of delayed brain injury, and inhibition of apoptosis with cycloheximide reduces the ensuing cerebral infarction in a newborn rat pup model of cerebral hypoxia-ischemia. These results suggest that anti-apoptotic treatments may have a useful role as neural rescue therapies for newborn infants, and such treatments deserve further exploration.
Figures and Tables
Fig. 1
Representative photomicrographs of Eosin staining (A) and TUNEL staining (B) at the anterior commisure from the hypoxia-ischemia control group (HI) and hypoxia-ischemia with cycloheximide treatment group (HI-CHX) 1 and 4 week after the injury. Note progression of infarction in HI, and significantly reduced infarct area and less TUNEL-positive staining cells bordering the infarct of HI-CHX, compared to HI.
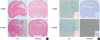
Fig. 2
Percentage of normal brain area at the anterior commissure from the hypoxia-ischemia control group (HI) and hypoxia-ischemia with cycloheximide treatment group (HI-CHX) 1 and 4 week after the injury. Significantly increased infarct area at 4 week compared to 1 week after the insult in HI, and significantly reduced infarct area of HI-CHX, compared to HI, was observed. *, p<0.05 compared to HI. †, p<0.05 compared to 1 week. All values are mean±standard deviation.
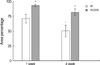
Fig. 3
Representative photomicrographs of fluorescent staining for TUNEL (green) and DAPI (blue) in the ipsilateral cortex (A) and the number of TUNEL positive cells (B) from the hypoxia-ischemia control group (HI)and hypoxia-ischemia with cycloheximide treatment group (HI-CHX) 48 hr after the injury. Significantly less TUNEL-positive staining cells of HI-CHX, compared to HI, are observed. All values are mean±standard deviation. *, p<0.05 compared to HI. Original magnification×400.
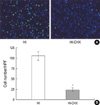
Fig. 4
Representative flow cytogram of an annexin V binding (abscissa, FL1) versus propidium iodide uptake (ordinate, FL2) in the ipsilateral hemisphere of the newborn rat brain cells at 48 hr after hypoxia-ischemia. The numbers in the left upper quadrant, right upper quadrant, left lower quadrant and right lower quadrant represent the percentage of damaged (annexin V-/PI+), necrotic (annexin V+/PI+), live (annexin V-/PI-), and apoptotic cells, respectively. HI, hypoxia-ischemia control group; HI-CHX, hypoxia-ischemia with cycloheximide treatment group.
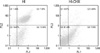
Table 1
Regional cell percentage of ipsilateral hemisphere at 48 hr after hypoxia-ischemia

Q1, Damaged (Annexin V-/Propidium iodide+) cells; Q2, Necrotic (Annexin V+/Propidium iodide+) cells; Q3, Live (Annexin V-/Propidium iodide-) cells; Q4, Apoptotic (Annexin V+/Propidium iodide-) cells; HI, hypoxia-ischemia control group; HI+CHX, hypoxia-ischemia with cycloheximide treatment group. All values given are mean±standard deviation.
*, p<0.05 compared to HI.
References
1. Delivoria-Papadopoulos M, Mishra OP. Mechanisms of cerebral injury in perinatal asphyxia and strategies for prevention. J Pediatr. 1998. 132:S30–S34.
2. Vannucci RC, Perlman JM. Interventions for perinatal hypoxic-ischemic encephalopathy. Pediatrics. 1997. 100:1004–1014.


3. Linnik MD, Zobrist RH, Hatfield MD. Evidence supporting a role for programmed cell death in focal cerebral ischemia in rats. Stroke. 1993. 24:2002–2009.


4. Renvoize C, Biola A, Pallardy M, Breard J. Apoptosis: identification of dying cells. Cell Biol Toxicol. 1998. 14:111–120.
5. Taylor DL, Edwards AD, Mehmet H. Oxidative metabolism, apoptosis and perinatal brain injury. Brain Pathol. 1999. 9:93–117.


6. Martin LJ, Al-Abdulla NA, Brambrink AM, Kirsch JR, Sieber FE, Portera-Cailliau C. Neurodegeneration in excitotoxicity, global cerebral ischemia, and target deprivation: a perspective on the contributions of apoptosis and necrosis. Brain Res Bull. 1998. 46:281–309.


7. Johnson EM Jr, Deckwerth TL. Molecular mechanisms of developmental neuronal death. Annu Rev Neurosci. 1993. 16:31–46.


8. Du C, Hu R, Csernansky CA, Hsu CY, Choi DW. Very delayed infarction after mild focal cerebral ischemia: a role for apoptosis? J Cereb Blood Flow Metab. 1996. 16:195–201.


9. Zhu C, Wang X, Xu F, Bahr BA, Shibata M, Uchiyama Y, Hagberg H, Blomgren K. The influence of age on apoptotic and other mechanisms of cell death after cerebral hypoxia-ischemia. Cell Death Differ. 2005. 12:162–176.


10. Pulera MR, Adams LM, Liu H, Santos DG, Nishimura RN, Yang F, Cole GM, Wasterlain CG. Apoptosis in a neonatal rat model of cerebral hypoxia-ischemia. Stroke. 1998. 29:2622–2630.


11. Northington FJ, Ferriero DM, Flock DL, Martin LJ. Delayed neurodegeneration in neonatal rat thalamus after hypoxia-ischemia is apoptosis. J Neurosci. 2001. 21:1931–1938.


12. Nakajima W, Ishida A, Lange MS, Gabrielson KL, Wilson MA, Martin LJ, Blue ME, Johnston MV. Apoptosis has a prolonged role in the neurodegeneration after hypoxic ischemia in the newborn rat. J Neurosci. 2000. 20:7994–8004.


13. Yue X, Mehmet H, Squier MV, Hope PL, Azzopardi D, Edwards AD. Apoptosis and necrosis in the brains of infants dying after birth asphyxia. Pediatr Res. 1995. 37:387A.
14. Rosenbaum DM, Michaelson M, Batter DK, Doshi P, Kessler JA. Evidence for hypoxia-induced, programmed cell death of cultured neurons. Ann Neurol. 1994. 36:864–870.


15. Pavlik A, Teisinger J. Effect of cycloheximide administered to rats in early postnatal life: prolonged of DNA synthesis in the developing brain. Brain Res. 1980. 192:531–541.
16. Hwang JH, Sung DK, Choi CW, Kang S, Chang YS, Park WS, Lee M. Single cell dissociation methods for flow cytometric analysis of hypoxia-ischemia injured newborn rat pup brain. Korean J Pediatr. 2005. 48:545–550.
17. Geddes R, Vannucci RC, Vannucci SJ. Delayed cerebral atrophy following moderate hypoxia-ischemia in the immature rat. Dev Neurosci. 2001. 23:180–185.


18. Wijsman JH, Jonker RR, Keijzer R, van de Velde CJ, Cornelisse CJ, van Dierendonck JH. A new method to detect apoptosis in paraffin sections: in situ end-labelling of fragmented DNA. J Histochem Cytochem. 1993. 41:7–12.
19. MacManus JP, Hill IE, Preston E, Rasquinha I, Walker T, Buchan AM. Differences in DNA fragmentation following transient cerebral or decapitation ischemia in rats. J Cereb Blood Flow Metab. 1995. 15:728–737.


20. Vermes I, Haanen C, Steffens-Nakken H, Reutelingsperger C. A novel assay for apoptosis: flow cytometric detection of phosphatidylserine expression on early apoptotic cells using fluorescein labeled annexin V. J Immunol Methods. 1995. 184:39–51.
21. Honda O, Kuroda M, Joja I, Asaumi J, Takeda Y, Akaki S, Togami I, Kanazawa S, Kawasaki S, Hiraki Y. Assessment of secondary necrosis of Jurkat cells using a new microscopic system and double staining method with annexin V and propidium iodide. Int J Oncol. 2000. 16:283–288.


22. Darzynkiewicz Z, Bender E. Analysis of apoptotic cells by flow and laser scanning cytometry. Methods Enzymol. 2000. 322:18–39.


23. Walsh GM, Dewdson G, Wardlaw AJ, Levi-Schaffer F, Moqbel R. A comparative study of different methods for the assessment of apoptosis and necrosis in human eosinophils. J Immunol Methods. 1998. 217:153–163.


24. Schutte B, Nuydens R, Geerts H, Ramaekers F. Annexin V binding assay as a tool to measure apoptosis in differentiated neuronal cells. J Neurosci Methods. 1998. 86:63–69.


25. Darzynkiewicz Z, Juan G, Li X, Gorczyca W, Murakami T, Traganos F. Cytometry in cell necrobiology: analysis of apoptosis and accidental cell death (necrosis). Cytometry. 1997. 27:1–20.

