Abstract
Pulmonary vascular resistance (PVR) is generally believed to be elevated after cardiopulmonary bypass (CPB) due to whole body inflammation. Aprotinin has an anti-inflammatory action, and it was hypothesized that aprotinin would attenuate the PVR increase induced by CPB. Ten mongrel dogs were placed under moderately hypothermic CPB for 2 hr. The experimental animals were divided into a control group (n=5, group I) and an aprotinin group (n=5, group II). In group II, aprotinin was administered during pre-bypass (50,000 KIU/kg) and post-bypass (10,000 KIU/kg) periods. Additional aprotinin (50,000 KIU/kg) was mixed in CPB priming solution. PVRs at pre-bypass and post-bypass 0, 1, 2, 3 hr were calculated, and lung tissue was obtained after the experiment. Post-bypass PVRs were significantly higher than prebypass levels in all animals (n=10, p<0.001). PVR elevation in group II was less than in group I at 3 hr post-bypass (p=0.0047). Water content of the lung was lower in group II (74±9.4%) compared to that of group I (83±9.5%), but the difference did not reach significance (p=0.076). Pathological examination showed a near normal lung structure in group II, whereas various inflammatory reactions were observed in group I. We concluded that aprotinin may attenuate CPB-induced PVR elevation through its anti-inflammatory effect.
Aprotinin, a non-specific serine protease inhibitor, has a molecular weight of 6,000 dalton and is extracted from a bovine lung. This drug has both anti-inflammatory and hemostatic effects. After being introduced for the treatment of acute pancreatitis in the 1950s, aprotinin had mostly been used for controlling clinical conditions with whole body inflammation such as hemorrhagic shock, multiple traumas, and acute respiratory distress syndrome. It was not until the early 1980s that aprotinin became widely used in the field of cardiovascular surgery as a hemostatic agent (1). Recently, there have been several reports regarding the anti-inflammatory effect of aprotinin, and one of the most outstanding observations is the beneficial effect of aprotinin for the patients with a functional single ventricle (2).
Cardiopulmonary bypass (CPB) is generally believed to cause an increase in pulmonary vascular resistance (PVR), which is sometimes fatal after one ventricle repair. The use of aprotinin may be helpful by attenuating post-bypass elevation of PVR in this setting. Aprotinin prevents CPB-induced lung injury through several mechanisms: inhibition of thromboxane A2 release by protecting the glycoprotein Ib receptor in platelets (3, 4), inhibition of endothelin-1 secretion from pulmonary vascular endothelium (5), and suppression of complement and neutrophil activation. Platelets are easily activated in patients with cyanotic congenital heart disease because the concentration of the glycoprotein Ib receptor is lower than normal (6). Therefore, aprotinin can be particularly useful in cyanotic patients with a functional single ventricle necessitating one ventricle repair.
There are several studies pertaining to the prevention of CPB-induced lung injury: elimination of leukocytes in bypass circuit by anti-leukocyte agents (mustine hydrochloride) or a leukocyte filter (7), prevention of leukocyte adhesion to pulmonary endothelium (8), addition of oxygen free radical scavengers (vitamin E, coenzyme Q, vitamin C, glutathione) (9), utilization of cyclooxygenase antagonists (indomethacin), thromboxane A1 synthetase antagonist (dazmegral) (10), platelet activation factor inhibitors (11), and steroid administration (12, 13). Although these methods may help to protect the pulmonary vascular bed to some extent, the clinical application of these agents is limited due to unproven safety and cost-effectiveness. Ultrafiltration after cardiopulmonary bypass, either conventional or modified, is believed to be an effective measure to ameliorate inflammatory reactions by eliminating inflammatory mediators (14, 15). This newly developed technique, however, focuses on the treatment, rather than the prevention, of systemic inflammatory response. Aprotinin is safe and cost-effective because it has been widely used as a hemostatic agent in open heart surgery, and aprotinin use can be further extended if it proves to prevent post-bypass lung injuries. I postulated that post-bypass elevation of PVR can be reduced by the administration of aprotinin. Therefore, the degree of post-bypass PVR elevation in the control group and the aprotinin treatment group was compared using a canine cardiopulmonary bypass model.
Ten male mongrel dogs, each weighing about 20 kg (18-23 kg) were divided into a control group (n=5, Group I) and an aprotinin administration group (n=5, Group II). After the administraion of sodium thiopental (25 mg/kg) and endotracheal intubation, the experimental animals were laid in supine positions and anesthesia was maintained with enflurane, N2O and O2. The initial ventilator setting was a tidal volume of 15 mL/kg, a frequency of 16/min and FiO2 of 0.3, and it was modulated according to the arterial blood gas analysis to maintain pH, PaO2 and PaCO2 close to 7.4, 100 mmHg and 40 mmHg, respectively. Pre-bypass hematocrit ranged from 45% to 55%, and there was no difference in the hematocrit levels between the two groups. A femoral arterial catheter was introduced to monitor arterial pressure, and a Foley catheter was placed. Through median sternotomy, the superior vena cava was dissected and a Swan-Ganz catheter (Edward true size pediatric thermodilution catheter, 5F, model 132F5, Baxter, Irvin, CA, U.S.A.) was inserted via a purse-string suture made in the superior vena cava with 6-0 prolene. The distal end of the catheter was positioned in the main pulmonary artery to assess cardiac output (C.O.) and pulmonary artery pressure (PAP). For the calculation of cardiac output, a cardiac output computer (American Edwards Laboratory, model COM-1 RS, Irvin, CA, U.S.A.) was used. Left atrial pressure was monitored via a left atrial pressure (LAP) line introduced into the left atrial auricle. After the administraion of heparin sulfate (3 mg/kg), arterial and single venous cannulae were placed in the ascending aorta and the right atrial auricle, respectively, and cardiopulmonary bypass was started. The bypass circuit consisted of two roller pumps (Sarns 7000, one-head, 6 inch, Cheshire, CT/Shiley-Stockert double-head, 3 inch, Cheshire, CT, U.S.A.), a bubble oxygenator (Bentley 10 plus, Irvin, CA, U.S.A.), a heat exchanger, and 3/8 inch tubing (350 cm for arterial and venous line, 100 cm for vent and sucker line). The priming solution consisted of Ringer's lactate (1,000 mL) with heparin sulfate 1,100 I.U., sodium bicarbonate 20 mL, mannitol 80 mL, and resultant hematocrit after the initiation of bypass was 25-30%. The animals were treated during the experiment and sacrificed at the end in accordance with the 'Guide for the Care and Use of Laboratory Animals' (National Research Council, U.S.A.).
The total experimental time was 300 min: 120 min of CPB and 180 min of post-bypass hemodynamic monitoring. Before the initiation of CPB, pulmonary artery pressure, left atrial pressure, and cardiac output were measured for the calculation of pre-bypass PVR. Perfusion flow rate was kept at 50 mL/kg/min with arterial blood pressure of 40-50 mmHg, while crystalloid addition to a venous reservoir was limited to less than 500 mL during bypass time in all cases. Rectal temperature was lowered to 25℃ for 1 hr and was re-elevated to 37℃ before the termination of CPB, and normal sinus rhythm was maintained during hypothermia in all cases. PO2 and PCO2 of perfusate were fixed to 100 mmHg and 40 mm Hg, respectively, and acid-base status was regulated according to the 'alpha stat' strategy. Heparin sulfate (1 mg/kg) was administered to the bypass circuit 1 hr after the initiation of cardiopulmonary bypass, and the ACT (activated coagulation time) was kept greater than 480 sec in group I and greater than 750 sec in group II by a celite activator during bypass time. After the termination of CPB, protamine (4.5 mg/kg) was administered, and ventilatory support was resumed. It was attepted to maintain normal blood gas profile by fine adjustments of ventilator settings throughout the post-bypass period for 3 hr. Perfusate in the circuit and oxygenator was recollected for post-bypass transfusion. The PVR prior to CPB and during post-bypass period for 3 hr at 1 hr intervals were calculated according to the following formula, which was corrected by body weight.
PVR=(mean PAP-mean LAP)/C.O.
Corrected PVR=PVR×body weight
The experimental animals were sacrificed three hours after the termination of cardiopulmonary bypass, and right lung tissue was obtained for both pathological examination and water content measurement.
Experimental conditions for group II were the same as group I, except for the use of aprotinin (Aprotimbin, BIOCHMIE Ges.m.b.H,. Austria). The aprotinin administration regimen was as follows:
Before bypass: 50,000 KIU/kg continuous infusion for 20 min
After bypass: 10,000 KIU/kg/hr for 3 hr
Mixed in priming solution: 50,000 KIU/kg
Post-bypass PVRs were compared with pre-bypass PVR in all of the animals (n=10) using the Wilcoxon signed rank test. For a comparison between the two groups, the ratio of post-bypass PVR to pre-bypass PVR was calculated. The ratios were compared between the two groups using the Wilcoxon rank sum test. The water content of lung tissue was also compared between the two groups non-parametrically. p-value under 0.05 was considered significant. Analyses were conducted using a PC-SAS 6.04 package.
The ratios of post-bypass PVRs (at post-bypass 0, 1, 2 and 3 hr) to pre-bypass PVRs in all of the animals (n=10) were 1.44±0.42, 1.88±0.44, 1.95±0.34, 1.86±0.26, respectively. The post-bypass PVRs were significantly higher than pre-bypass PVRs in all of the dogs (n=10) throughout the post-bypass period (p<0.01), but there was no difference in the post-bypass PVR ratios (p>0.1). Post-bypass PVR in group I gradually increased after CPB while post-bypass PVRs were similar in group II (Fig. 1). The ratio of the post-bypass 3 hr PVR to pre-bypass PVR was significantly greater in group I compared to group II (Table 1, p=0.0047).
The water contents of the lung in group l and group II were 83±9.5% and 74±9.4%, respectively, but the difference did not reach statistical significance (p=0.076) (Fig. 2).
Pathological examination showed inflammatory cell infiltration in the pulmonary interstitium, destruction of the alveolar structure, and vasoconstriction of small to medium sized pulmonary arteries in group I. Near normal histology was observed in group II (Fig. 3).
The surgical correction of many congenital and acquired cardiac diseases has been made possible by theoretical and technical developments in cardiopulmonary bypass (CPB) ever since this innovative technique was introduced in the early 1950s. Whole body inflammatory response due to contact activation of blood components exposed to foreign materials in bypass circuit, however, is still an unsolved problem, which causes a variety of post-bypass complications in many organs (16, 17). The lung is deemed the most important organ in this regard because it is susceptible to CPB-induced injury due to its weak supporting tissue and abundant vascularity and because abnormal gas exchange and instability of PVR have a devastating impact on postoperative course after cardiac surgery. Elevation of PVR after extra-corporeal circulation is thought to originate from endothelial dysfunction. Postoperative pulmonary vascular instability has special implications in pediatric patients because it is associated with pulmonary hypertensive crisis in patients with preoperative pulmonary hypertension, hemodynamic instability after palliative operations in which postoperative systemic circulation is determined by the balance between PVR and SVR (systemic vascular resistance), and inadequate oxygenation after one ventricle repair (bi-directional cavo-pulmonary shunt or Fontan operation). Several clinical studies regarding the beneficial effect of aprotinin on postoperative course after one ventricle repair have been reported (2), and these observations imply that aprotinin can be used for both hemostatic (17-22) and anti-inflammatory purposes. The mechanisms of anti-inflammatory effects of aprotinin is not fully understood, but various indirect evidences have been accumulated through in vivo and in vitro studies including the attenuation of post-bypass elevation of interleukin-8 in BALF (bronchial alveolar lavage fluid) (23), suppression of complement activation upon hemodialysis (24), reduction of inflammatory mediators in an experimental bypass circuit (25), the beneficial effect of aprotinin on post-bypass cardiac function in a swine experimental model (26), beneficial effect of aprotinin on renal function recovery after cardiopulmonary bypass (27), reduction of post-bypass intra-bronchial i-NOS (inducible NO synthase) during cardiopulmonary bypass (28), reduction of the concentration of cell adhesion molecules (ICAM, VCAM, ELAM) (29), the beneficial effect of aprotinin on neurologic recovery after circulatory arrest (30), and so forth. With respect to its effect on the pulmonary vascular bed, several hypothetical mechanisms have been postulated including inactivation of the kallikrein-bradykinin system, suppression of complement activation, suppression of the secretion of vaso-active substances (thromboxane A2, endothelin) (31), protection of pulmonary vascular endothelial functions, and maintenance of the production of nitric oxide.
One of the limitations of this study is that the dose of aprotinin is arbitrary and relatively 'high' compared to other experiments. The aprotinin regimen of this experiment is based on a clinical report of 'high dose' aprotinin treatment for pediatric cardiac patients (32). Aprotinin doses used in other experiments, however, vary widely from one another (33), and there is no recommended dose of aprotinin with which the anti-inflammaotry effect is anticipated. Furthermore, hemodilution in small children (34, 35) during cardiopulmonary bypass can make it more difficult to predict adequate serum aprotinin levels in a clinical setting (20, 25). Therefore, direct measurement of serum aprotinin levels may be helpful for the precise evaluation of the effect of aprotinin.
Aprotinin has already been widely used as a hemostatic agent in various types of open heart surgery, and the indication of aprotinin use can be extended if its ability to attenuate post-bypass lung injury is verified, especially in the pediatric patient population. Additional experimental and clinical studies are needed to find out the exact mechanisms of the effects of aprotinin on the pulmonary vascular bed, and also to determine the ideal regimen of aprotinin in various clinical settings.
Figures and Tables
Fig. 1
The ratio of post-bypass over pre-bypass PVR in the control and the aprotinin groups.
PVR, prebypass PVR; PVR 0-3, postbypass 0-3 hr PVR.
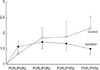
References
2. Tweddell JS, Berger S, Frommelt PC, Pelech AN, Lewis DA, Fedderly RT, Frommelt MA, McManus TS, Mussatto KA, Kessel MW, Litwin SB. Aprotinin improves outcome of single-ventricle palliation. Ann Thorac Surg. 1996. 62:1329–1336.


3. Lu H, Soria C, Commin PL, Soria J, Piwnica A, Schumann F, Regnier O, Legrand Y, Caen JP. Hemostasis in patients undergoing extracorporeal circulation: the effect of aprotinin (Trasylol). Thromb Haemost. 1991. 66:633–637.


4. Huang H, Ding W, Su Z, Zhang W. Mechanism of the preserving effect of aprotinin on platelet function and its use in cardiac surgery. J Thorac Cardiovasc Surg. 1993. 106:11–18.


5. Komai H, Adatia IT, Elliott MJ, de Leval MR, Haworth SG. Increased plasma levels of endothelin-1 after cardiopulmonary bypass in patients with pulmonary hypertension and congenital heart disease. J Thorac Cardiovasc Surg. 1993. 106:473–478.


6. Rinder CS, Gaal D, Student LA, Smith BR. Platelet-leukocyte activation and modulation of adhesion receptors in pediatric patients with congenital heart disease undergoing cardiopulmonary bypass. J Thorac Cardiovasc Surg. 1994. 107:280–288.


7. Bando K, Pillai R, Cameron DE, Brawn JD, Winkelsteins JA, Hutchin GM, Reitz BA, Baumgartner WA. Leukocyte depletion ameliorates free radical-mediated lung injury after cardiopulmonary bypass. J Thorac Cardiovasc Surg. 1990. 99:873–877.


8. Gillinov AM, Redmond JM, Zehr KJ, Wilson IC, Curtis WE, Bator JM, Burch RM, Reitz BA, Baumgartner WA, Herskowitz A. Inhibition of neutrophil adhesion during cardiopulmonary bypass. Ann Thorac Surg. 1994. 57:126–133.


9. Utley JR. Pathophysiology of cardiopulmonary bypass: Current Issues. J Card Surg. 1990. 5:177–189.


10. Cave AC, Marche A, Derias NW, Hearse DJ. Thromboxane A2 mediates pulmonary hypertension after cardiopulmonary bypass in the rabbit. J Thorac Cardiovasc Surg. 1993. 106:959–967.


11. Zehr KJ, Poston RS, Lee PC, Uthoff K, Kumar P, Cho PW, Gillinov AM, Redmond JM, Winkelstein JA, Herskowitz A. Platelet activating factor inhibition reduces lung injury after cardiopulmonary bypass. Ann Thorac Surg. 1995. 59:328–335.


12. Bourbon A, Vionnet M, Leprince P, Vaissier E, Copeland J, McDonagh P, Debre P, Gandjbakhch I. The effect of methylprednisolone treatment on the cardiopulmonary bypass-induced systemic inflammatory response. Eur J Cardiothorac Surg. 2004. 26:932–938.


13. Celik JB, Gormus N, Okesli S, Gormus ZI, Solak H. Methylprednisolone prevents inflammatory reaction occurring during cardiopulmonary bypass: effects on TNF-alpha, IL-6, IL-8, IL-10. Perfusion. 2004. 19:185–191.
14. Chew MS, Brix-Christensen V, Ravn HB, Brandslund I, Ditlevsen E, Pedersen J, Hjortholm K, Hansen OK, Tonnesen E, Hjortdal VE. Effect of modified ultrafiltration on the inflammatory response in paediatric open-heart surgery: a prospective, randomized study. Perfusion. 2002. 17:327–333.
15. Shimpo H, Shimamoto A, Sawamura Y, Fujinaga K, Kanemitsu S, Onoda K, Takao M, Mitani Y, Yada I. Ultrafiltration of the priming blood before cardiopulmonary bypass attenuates inflammatory response and improves postoperative clinical course in pediatric patients. Shock. 2001. 16:51–54.


16. Allison PM, Whitten CW. What is the mechanism of action of aprotinin? Anesthesiology. 1991. 75:377–379.


17. Boyle EM Jr, Pohlman TH, Johnson MC, Verrier ED. Endothelial cell injury in cardiovaslcuar surgery: the systemic inflammatory response. Ann Thorac Surg. 1997. 63:277–284.
18. Royston D, Bidstrup BP, Sapsford RN, Taylor KM. Effect of aprotinin on need for blood transfusion after repeat open-heart surgery. Lancet. 1987. 2:1289–1291.


19. Bidstrup BP, Royston D, Sapsford RN, Taylor KM. Reduction in blood loss and blood use after cardiopulmonary bypass with high dose aprotinin (Trasylol). J Thorac Cardiovasc Surg. 1989. 97:364–372.


20. Boldt J, Knothe C, Zickermann B, Wege N, Dapper F, Hempelmann G. Comparison of two aprotinin dosage regimens in pediatric patients having cardiac operations; Influence on platelet function and blood loss. J Thorac Cardiovasc Surg. 1993. 105:705–711.
21. HerynKopf F, Lucchese F, Pereira E, Kalil R, Prates P, Nesralla IA. Aprotinin in children undergoing correction of congenital heart defects. A double-blind pilot study. J Thorac Cardiovasc Surg. 1994. 108:517–521.
22. Penkoske PA, Entwistle LM, Marchak BE, Seal RF, Gibb W. Aprotinin in children undergoing repair of congenital heart defects. Ann Thorac Surg. 1995. 60:S529–S532.


23. Hill GE, Pohorecki R, Alonso A, Rennard SI, Robbins RA. Aprotinin reduces interleukin-8 production and lung neutrophil accumulation after cardiopulmonary bypass. Anesth Analg. 1996. 83:696–700.


24. Himmelfarb J, Holbrook D, McMonagle E. Effects of aprotinin on complement and granulocyte activation during ex vivo hemodialysis. Am J Kidney Dis. 1994. 24:901–906.


25. Wachtfogel YT, Kucich U, Hack CE, Gluszko P, Niewiarowski S, Colman RW, Edmunds LH Jr. Aprotinin inhibits the contact, neutrophil, and platelet activation systems during simulated extracoporeal perfusion. J Thorac Cardiovasc Surg. 1993. 106:1–10.
26. Ali M, Becket J, Brannan J, Fleming J, Taylor KM. The effect of high dose aprotinin therapy on the systemic inflammatory response in a porcine model of cardiopulmonary bypass. Perfusion. 1996. 11:278–280.


27. Blauhut B, Gross C, Necek S, Doran JE, Spath P, Lundsgaard-Hansen P. Effects of high-dose aprotinin on blood loss, platelet function, fibrinolysis, complement, and renal function after cardiopulmonary bypass. J Thorac Cardiovasc Surg. 1991. 101:958–967.


28. Hill GE, Springall DR, Robbins RA. Aprotinin is associated with a decrease in nitric oxide production during cardiopulmonary bypass. Surgery. 1997. 121:449–455.


29. Boldt J, Osmer C, Linke LC, Dapper F, Hempelmann G. Circulating adhesion molecules in pediatric cardiac surgery. Anesth Analg. 1995. 81:1129–1135.


30. Aoki M, Jonas RA, Nomura F, Stromski ME, Tsuji MK, Hickey PR, Holtzman DH. Effects of aprotinin on acute recovery of cerebral metabolism in piglets after hypothermic circulatory arrest. Ann Thorac Surg. 1994. 58:146–153.


31. Lim C, Yun TJ, Kim YS, Kim SH, Lee JD, Rho JR, Song MG. Effect of aprotinin on changes in plasma thromboxane B2 and endothelin-1 concentration after extracorporeal circulation. Korean J Thorac Cardiovasc Surg. 2000. 33:221–230.
32. Carrel TP, Schwanda M, Vogt PR, Turina MI. Aprotinin in pediatric cardiac operations: a benefit in complex malformations and with high-dose regimen only. Ann Thorac Surg. 1998. 66:153–158.


33. Dietrich W, Mossinger H, Spannagl M, Jochum M, Wendt P, Barankay A, Meisner H, Richter JA. Hemostatic activation during cardiopulmonary bypass with different aprotinin dosages in pediatric patients having cardiac operations. J Thorac Cardiovasc Surg. 1993. 105:712–720.


34. Kern FH, Morana NJ, Sears JJ, Hickey PR. Coagulation defects in neonate during cardiopulmonary bypass. Ann Thorac Surg. 1992. 54:541–546.
35. Mcdonough J, Gruenwald C. The use of aprotinin in pediatric patients: a review. J Extra Corpor Technol. 2003. 35:346–349.