Abstract
In order to investigate the expression patterns of the transforming growth factor (TGF)β isoforms in the internal ear, an immunohistochemical study of rat embryos was performed. Rat embryos were taken on the 13th, 15th, 17th, and 19th day after conception and their internal ears were immunohistochemically stained against TGFβ1, β2, and β3. As a result, the 13-day-old embryo showed a very weak positivity to TGFβ1. After the 15th day of pregnancy, no reactivity to TGFβ1 was defected. Immunoreactivity to TGFβ2 was observed from the 15th day of pregnancy throughout the rest of the period. The ampulla of the semicircular canal and the cochlear duct showed a notably strong immunohistochemical reaction. A strong reaction to TGFβ3 was observed on the 15th day of pregnancy. However, no positive reactions were observed thereafter. A strong immunoreactivity was observed especially on the apical cytoplasms, the surfaces of the epithelial cells, and basement membranes of the cochlear duct, as well as the semicircular canals of the developing internal ear of rat embryo.
The ear, a member of the special sensory organs, consists of three parts: the external, middle and internal ear. At the embryonic stage, the internal ear develops first. The first evidence of development of the human internal ear is the formation of the otic placode after thickening of the surface ectoderm early in the 4th week. Next, the otic placode invaginates and sinks into the underlying mesenchyme and forms an otic pit. Towards the end of the 4th week, around the 26th day of development, the superficial epithelial opening of the otic pit is closed and forms the otic vesicle. By the 5th week, the endolymphatic appendage is formed within the posterolateral part of the otic vesicle and begins to grow. At the same time, other parts of the otic vesicle are differentiated as primordia of the utricle and saccule. Around the 6th week of development, the cochlear duct begins to be formed from the saccule, and flat disk-like diverticula grow out of the utricular portion. The terminal portions of each semicircular canal are enlarged and form the ampulla (1-4).
The process by which the internal ear is formed in mouse is known to be almost identical to that in human. The time when the otic vesicle is shaped in mouse is the 9.5th day, which corresponds to the stage 15 in human, namely about the 33rd postovulatory day according to the Carnegie stage (5). From the otic vesicle, the endolymphatic appendage is formed around the 10th day.
On the 11.5th day, it is possible to distinguish the portions where the utricle and saccule are formed. The flat diverticula, which will form the semicircular canals and primordium of the cochlear duct, are formed from the utricle and saccule, respectively, at around day 12 (6, 7). In rat, compared with in mouse, the formation of the internal ear progresses more slowly, but the order of the developmental stages is similar.
The transforming growth factor β (TGFβ) is a polypeptide growth factors family composed of many isomers. This protein group is known as the TGFβ family (8). Based on the structural differences of the specific region, TGFβ is generally classified as four isomers: TGFβ1, β2, β3 and β5.
TGFβ stimulates or inhibits the growth and differentiation of cells, and also controls the production and secretion of the extracellular matrix (9). The time when these functions of TGFβ are well displayed in individuals is the developmental process of embryos (10-12).
Recently, there have been many studies focused on the functions of these growth factors during the development of experimental animals. Therefore, TGFβ is thought to play important roles in the parts where various interactions occur between ectodermal cells and mesodermal cells during the development, especially in the internal ear. It is also known that the hair cells of the cochlea of developing mouse express TGFβ2 mRNA (12-14). By using the immunohistochemical technique, it was shown that TGFβ2 is also expressed in the internal ear, but unlike the TGFβ2 mRNA, it is limited on the basement membrane of the cochlear epithelia. The expression of TGFβ2 continues from the 12th day to the 17th day of development. TGFβ1 is not expressed in the cochlear duct of mouse embryo. TGFβ3 is expressed on all epithelial cells of the embryonic cochlear duct on the 13th day after fertilization and this positive signal continues until the 17th day. This protein is detected mostly on cell surface. Therefore, during the development of the internal ear of mouse embryo, the expression of TGFβ isoforms undergoes the most dramatic change (15).
The effects of TGFβs on cells are confirmed by numerous studies that demonstrated the expression and disappearance of these isoforms in developing tissues or organs. Since most studies on the development of the internal ear has so far been focused on the expression of TGFβ isoform, without consideration of the developmental stages, we performed this study to confirm the process of the development of the internal ear and to observe the expression patterns and various influences upon the neighboring cells of TGFβs in rat embryos during the period from the 13th day to the 19th day of gestation at intervals of two days.
In order to observe the expressions of TGFβs during the development of the internal ear, we used female Sprague-Dawley (SD) rats. We raised these animals in isolated conditions with free feeding until their weight reached 230-250 g. Then, we mated them with males in the evening and confirmed the fertilization by observing the vaginal plug or sperm on the vaginal smear next morning. We designated this day as day 0 of gestation. On the 13th, 15th, 17th, and 19th day of gestation, the embryos were extracted. On each selected day of pregnancy, five female rats were sacrificed and two embryos from each were selected and used for this study.
The embryos were fixed overnight in 10% neutrally buffered formalin immediately after extraction from the uterus. The fixed embryos were washed, dehydrated, and embedded in paraffin. Serial sections were made by 5-µm thickness, attached onto the aminopropyl-triethoxysilane coated slide glass, and air-dried. The tissue sections were deparaffinized, hydrated, stained with hematoxylin-eosin (HE), dehydrated, cleared and sealed with cover glass. Histologic characteristics were examined under a light microscope (BX50, Olympus, Japan).
The sections were deparaffinized, hydrated, and then treated with 3% H2O2 in methanol to block the activity of the endogenous peroxidase. After washing with phosphate buffered saline (PBS 0.1M, pH 7.4) for 5 min, the sections were treated with a 10% protein blocking agent (Shandon, Waltham, MA, U.S.A.) for 1 hr at room temperature to block nonspecific antigen-antibody reactions. These sections were incubated with 1:400 anti-TGFβ1, β2 and β3 antibody (Santa Cruz Biotechnology, Santa Cruz, CA, U.S.A.) diluents for 12 hr at 4℃ and washed with PBS. Thereafter, they were incubated with biotinylated secondary antibodies (Shandon) for 60 min at room temperature. After being washed with PBS, these were treated with streptavidin peroxidase reagent (Shandon) for 30 min at room temperature. After administering the peroxidase treatment, the tissue sections were washed with PBS and colorized with a 0.05% diaminobenzidine (DAB, Vector Laboratories Inc., Burlingame, CA, U.S.A.) solution for less than 1 min.
We took pictures of the HE stained and immunohisochemically stained sections using a light microscope and compared the degrees of staining intensities from each slide. On the 17th day of gestation, the embryos were prepared and sectioned serially at 25-µm thickness. These sections were stained with HE. Then, we took photographs and reconstructed the structure of the developing labyrinth using a desktop personal computer.
From the 13th day of gestation, the embryos showed the otic vesicle clearly and the endolymphatic appendage was sunken posteromedially. The endolymphatic appendage was observed as a large elliptical structure with a small projection in the anterior portion (Fig. 1). At this stage, all TGF beta isoforms showed very weak signals on the epithelial cells and also on the subepithelial tissues of the otic vesicle and endolymphatic appendage, while TGFβ3 showed rather intense signals. Positive immunoreactivity was observed on the posterior part of the otic vesicle, which was composed of columnar epithelial cells. Indeed, it appeared clearly on the surface of those columnar cells. However, there were no significant differences in the expression patterns of TGFβ family on the epithelia of the otic vesicle and endolymphatic appendage at this stage (Fig. 1).
On the 15th day, the internal ear was observed to develop as a form of labyrinth composed of tubes. It was also possible to observe parts of the saccule, utricle, cochlear duct, and lateral semicircular canal (Fig. 2). The immunoreactivity against TGFβ1 was not detected in the above developing internal ear structures, but TGFβ2 and β3 showed apparently positive immunoreactivity.
TGFβ2 showed strong immunoreactivity in the thick epithelial layer within the developing cochlear duct, especially on the surfaces of these sensory epithelial cells. The epithelial cells of the saccule from which the cochlear duct grows showed positive immunoreactivity with anti-TGFβ2 antibody. Furthermore, we found positive reactions in the basement membrane of the saccular epithelia. The epithelia of the lateral semicircular canal showed DAB coloring which was more distinct on the apical domain of these cells (Fig. 2).
TGFβ3 showed a similar immunoreactivity to that of TGFβ2 except for some different results on the epithelial surface of the developing saccule and lateral semicircular canal. In the basement membrane of the saccule, TGFβ3 showed less intense DAB coloring than TGFβ2. On the other hand, it is evident that some columnar cells of the developing saccule adjacent to the cochlear duct and the plate of lining cells of the developing cochlear duct expressed both TGFβ2 and TGFβ3 (Fig. 2).
The ampullar portions, where the posterior and anterior semicircular canals grow, were observed clearly in the embryo. From the results of three dimensional reconstruction of serial sections, the structures of the saccule, utricle, cochlea, lateral and posterior semicircular canal were partially observed. The cochlear duct further developed and it made 1.5 turns (Fig. 3, 4).
In the internal ear, immunoreactivity to TGFβ3 was greatly reduced, and positive signals were not observed as in the case of TGFβ1. Very weak immunoreactivity of TGFβ1 and β3 was detected on the surface of epithelia of the ampulla of the semicircular canal and the cochlear duct. In contrast to TGFβ1 and β3, TGFβ2 showed strong immunoreactivity in all parts of the internal ear, especially in the cellular mass lining along the portion toward the site of future spiral ganglion of the cochlea, the surface of epithelial cells, and the basement membrane of the ampullae of the semicircular canals (Fig. 3, 4).
On the 19th day of pregnancy, TGFβ1 and β3 showed no DAB coloring in the internal ear, similar to what we observed on the 17th day. However, the positive immunoreactivity to TGFβ2 was observed in all of the semicircular canals, ampullae and cochlear ducts, but was relatively weaker than those of the 17th-day embryos.
The epithelia of the semicircular canal showed DAB coloring on the apical surfaces. In the ampullae of the semicircular canal, the development of the ampullary crest was observed and some of the ciliated epithelia of the ampullary crest, especially on the apical surfaces, showed more intense signal with the TGFβ2 antibody. In the cochlear duct, a thick epithelial layer settled on the lateral wall of the duct, and these cells showed strong immunoreactivity on the apical cytoplasms and on their surfaces (Fig. 5).
In the developing internal ear of mouse, especially in the hair cells of the cochlear duct, it is known that the expression of TGFβ2 continues from the 12th day to the 17th day of pregnancy (11-14). In contrast to TGFβ2, TGFβ1 is not expressed in the cochlear duct of mouse embryo, and TGFβ3 is expressed somewhat later than β2 in the cochlear duct from the 13th day to the 17th day after fertilization (15, 16).
In this study, SD rats were used as experimental animals. Compared with previous studies, we found that the development of the internal ear in SD rats followed the same course as that in mouse. Thus, we speculate that these growth factors have similar functions in different species such as mouse and rat. In addition, cells from a specific location did not show the same immunoreactivity concordantly, and positive reactivity was restricted within the specific group of cells, while other cells show expression of two or more phenotypes simultaneously. This suggests the development and differentiation of cells are not uniform and their role in the developmental process may differ greatly.
According to the results from the above experimental data, it might be suggested that TGFβ2 plays important roles during the whole developmental process of the internal ear. Indeed, the cochlear duct, spiral limbus, and interdental cells showed a morphological abnormality when the TGFβ2 gene had intentionally been removed (17).
Recently, bone morphogenetic protein 4 (BMP-4), a member of the TGFβ family, has also been reported to play important roles in the development of the internal ear of frog and chicken (18-21).
During the internal ear development, mesodermal tissues are differentiated and form the otic capsule. In addition, it was asserted that many interactions occur between the epithelial cells and the mesodermal tissues and that growth factors participate in these interactions during the otic capsule formation. In particular, it is known that TGFβ1 is expressed in both epithelial cells and mesodermal tissues. Thus, TGFβ1 may play certain roles in the process of formation of the otic capsule (22, 23). Another line of evidence that supports the above statement is that the retinoic acid, a potent teratogen, produces abnormalities also in the internal ear and it was suggested that TGFβ1 might play an important role in this phenomenon (24). In this case, abnormalities were mainly due to the hypoplasia of the cartilaginous tissue. Moreover, they were associated with an apparent decrease of immunoreactivity in adjacent mesodermal tissues with the treatment of anti-TGFβ1 antibody. It was also reported that the TGFβ1 expression of the periotic tissue disappears right after finishing its role around the embryonic day 14 (23), and the observation of very weak immunoreactivity to TGFβ1 in a 13-day embryo logically suggests that the chondrogenesis occurring around the otic capsule had already been completed. Another recent study showed that the expression of TGFβ receptor II and Smad2, a downstream component of the TGFβ signal transduction pathway, were downregulated after the treatment with a high dose of retinoic acid (25).
In this study, we could not observe immunoreactivity with the anti-TGFβ1 antibody except at early stages of development. To explain these differences, further studies involving a wider time-window during the development, with shorter intervals. When we consider the differences between species, it is necessary to search other growth factors that participate in the differentiation of cartilaginous tissues.
The internal ear is composed of many tubular tissues, and the epithelial cells of these ducts are not a single cellular group. Thus, in order to confirm how these different cells under differentiation, we need elaborate reorganizations of the serial sections and special staining tools, such as the immunohistochemical or in situ hybridization techniques.
Figures and Tables
Fig. 1
Photomicrographs of hematoxylin-eosin stains (A) and immunohistochemical stains using antibodies against TGFβ1 (B), β2 (C), and β3 (D) in the developing internal ear of the 13th-day rat embryo. Horizontal section. The otic vesicle (a) is observed and endolymphatic appendage (b) forms another cavity medial to the otic vesicle. At this stage, the immunoreactivities to all the TGFβ isoforms are very weak (×200).
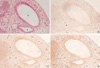
Fig. 2
Photomicrographs of hematoxylin-eosin stains (A) and immunohistochemical stains using antibodies against TGFβ2 (B) and β3 (C) in the developing internal ear of the 15th-day rat embryo. Sagittal section. Magnification ×100. At this stage, the cochlear duct (a), saccule (b) and lateral semicircular canal (c) could be identified. No immunoreactivity to TGFβ1 was observed (result not shown). Some epithelial regions show immunoreactivities to TGFβ2 (B) and TGFβ3 (C) in each sections of the cochlear duct and saccule (arrows).
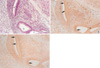
Fig. 3
Photomicrographs of hematoxylin-eosin stains (A, ×100) and immunohistochemical stains using antibodies against TGFβ2 (B, ×100; C, D, ×400) in the developing internal ear of the 17th-day rat embryo. Sagittal sections. At this stage, cochlear duct (a), ampulla (b), and semicircular canals (c, d) can be identified. In the epithelium of cochlear duct and semicircular canals, strong immunohistochemical reactions of TGFβ2 antibodies are observed, especially at the apices of lining columnar cells (arrows).
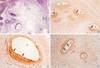
Fig. 4
A 3-dimensional reconstruction of the sagittal sections of the developing internal ear of 17th-day rat embryo. In the middle, the utricle (Utr) and the saccule (Sac) are interconnected and the ampulla (Amp) made a growth upward from them. Two semicircular canals, namely anterior (ASC) and posterior (PSC), formed loops lateralward, and the cochlear duct (Coc) roughly had a spiral form located medially.
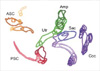
Fig. 5
Photomicrographs of hematoxylin eosin stains (A, B, ×100) and immunohistochemical stains using antibodies against TGFβ2 (C, D, ×100; E, F, ×400) in the developing internal ear of the 19th-day rat embryo. Sagittal sectons. At this stage, the cochlear duct (a), ampulla (b), saccule (c) and semicircular canals (d) are clearly identified. The immunoreactivity to TGFβ2 was relatively weaker than those of 17th-day embryo is observed at the apices of columnar cells of cochlear duct and crista ampullaris (arrows).
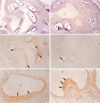
References
1. Larsen WJ. Human Embryology. 2001. 3rd Edition. Oxford: Churchill Livingstone;392–412.
2. Moore KL, Persaud TV. The Developing Human: Clinically oriented embryology. 2003. 7th Edition. Philadelphia: Saunders;476–479.
3. Park HW. Human embryology. 2005. 3rd Edition. Seoul: Koonja Publishing Inc.;503–507.
4. Streit A. Origin of the vertebrate inner ear: evolution and induction of the otic placode. J Anat. 2001. 199:99–103.


5. O'Rahilly R, Muller F. Developmental Stages in Human Embryos. 1987. Washington DC: Carnegie Institution of Washington;186.
6. Theiler K. The House Mouse. 1972. New York: Springer-Verlag;44–108.
7. Rugh R. The mouse. Its reproduction and development. 1991. Oxford: Oxford University Press;249–251.
8. Miller DA, Pelton RW, Derynck R, Moses HL. Transforming growth factor-beta. A family of growth regulatory peptides. Ann N Y Acad Sci. 1990. 593:208–217.
9. McCartney-Francis NL, Frazier-Jessen M, Wahl SM. TGF-beta: a balancing act. Int Rev Immunol. 1998. 16:553–580.
10. Akhurst RJ, Lehnert SA, Gatherer D, Duffie E. The role of TGF beta in mouse development. Ann N Y Acad Sci. 1990. 593:259–271.
11. Nilsen-Hamilton M. Transforming growth factor-beta and its actions on cellular growth and differentiation. Curr Top Dev Biol. 1990. 24:95–136.
12. Millan FA, Denhez F, Konaiah P, Akhurst RJ. Embryonic gene expression patterns of TGFβ1, β2 and β3 suggest different development functions in vivo. Development (Camb.). 1991. 111:131–143.
13. Pelton RW, Dickinson ME, Moses HL, Hogan BL. In situ hybridization analysis of TGFβ3 RNA expression during mouse development: comparative studies with TGF β1 and β2. Development (Camb.). 1990. 110:609–620.
14. Schmid P, Cox D, Bilbe G, Maier R, McMaster GK. Differential expression of TGFβ1, β2 and β3 genes during mouse embryogenesis. Development (Camb.). 1991. 111:117–130.
15. Pelton RW, Saxena B, Jones M, Moses HL, Gold LI. Immunohistochemical localization of TGFβ1, TGFβ2 and TGFβ3 in the mouse embryo: Expression patterns suggest multiple roles during embryonic development. J Cell Biol. 1991. 4:1091–1105.
16. Takemura T, Sakagami M, Takebayashi K, Umemoto M, Nakase T, Takaoka K, Kubo T, Kitamura Y, Nomura S. Localization of bone morphogenetic protein-4 messenger RNA in developing mouse cochlea. Hear Res. 1996. 95:26–32.


17. Paradies NE, Sanford LP, Doetschman T, Friedman RA. Developmental expression of the TGFβ in the mouse cochlea. Mech Dev. 1998. 79:165–168.
19. Morsli H, Choo D, Ryan A, Johnson R, Wu DK. Development of the mouse inner ear and origin of its sensory organs. J Neurosci. 1998. 18:3327–3335.


20. Cole LK, Le Roux I, Nunes F, Laufer E, Lewis J, Wu DK. Sensory organ generation in the chicken inner ear: contributions of bone morphogenetic protein 4, serrate1, and lunatic fringe. J Comp Neurol. 2000. 424:509–520.
21. Kil SH, Collazo A. Origin of inner ear sensory organs revealed by fate map and time lapse analyses. Dev Biol. 2001. 233:365–379.
22. Frenz DA, Van de Water TR, Galinovic-Schwartz V. Transforming growth factor beta: does it direct otic capsule formation? Ann Otol Rhinol Laryngol. 1991. 100:301–307.


23. Frenz DA, Galinovic-Schwartz V, Liu W, Flanders KC, Van de Water TR. Transforming growth factor beta 1 is an epithelial-derived signal peptide that influence otic capsule formation. Dev Biol. 1992. 153:324–336.
24. Frenz DA, Liu W. Treatment with all-trans-retinoic acid decreases levels of endogenous TGF-beta (1) in the mesenchyme of the developing mouse inner ear. Teratology. 2000. 61:297–304.