Abstract
The purpose of this study is to investigate the mechanism of alternative responses to low dose irradiation for neuronal cell proliferation in the dentate gyrus of rats. To determine the effect of a single exposure to radiation, rats were irradiated with a single dose of 0.1, 1, 10 or 20 Gy. To determine the effect of the cumulative dose, the animals were irradiated daily with 0.01 Gy or 0.1 Gy from 1 to 4 days. The neuronal cell proliferation was evaluated using immunohistochemistry for 5-bromo-2'-deoxyuridine (BrdU), Ki-67 and terminal deoxynucleotidyl transferase-mediated dUTP nick end-labeling (TUNEL) staining. Four consecutive daily irradiations with a 0.01 Gy/fraction increased the number of BrdU-positive and Ki-67-positive cells in a dose dependent manner, but this did not affect the number of TUNEL-positive cells. However, there was not a dose dependent relationship for the 0.1 Gy/fraction irradiation with the number of BrdU, Ki-67 and TUNEL positive cells. Our data support the explanation that the adaptive response, induced by low-dose radiation, in the hippocampus of rats is more likely a reflection of the perturbations of cell cycle progression.
Radiation is known to cause neuronal cell death in the developing brain (1), and it reduces neurogenesis (2-4). However, low dose radiation (LDR) is known to show a protection effect in most mammalian cells (5). Olivieri et al. showed that lymphocytes exposed to LDR developed a high resistance to the subsequent radiation-induced chromosome aberrations, and this was termed the "adaptive response" (6). Wolff reported that if cells that had been exposed to a very low dose (1 cGy) of radiography were subsequently exposed to a relatively high dose (1 Gy), approximately half as many chromosome breaks were induced (7). Feinendegen et al. reported that the protective responses to single exposures tended to be maximally expressed after about 0.1 Gy and very little protective response was noted after more than about 0.5 Gy radiography radiation or gamma-radiation (8). Also, adaptive responses for the neurogenesis of the dentate gyrus of the hippocampus have been reported with transient (9) or focal ischemia (10). Kang et al. have recently reported that a single exposure of 0.1 Gy radiation induced protective effects in the dentate gyrus of rats against diabetes-induced neuronal suppression (11). However, there has been no such investigation for the neuronal cell responses related with low dose radiation. Thus, we investigated the relationship of low dose radiation and the adaptive response of neuronal cells in the hippocampal dentate gyrus of the rat with using the effective proliferative markers 5-bromo-2'-deoxyuridine (Brd-U) and Ki-67 (12) and the apoptotic marker terminal deoxynucleotidyl transferase mediated dUTP nick end-labeling (TUNEL).
Male Sprague-Dawley rats weighing 200±10 g each (6 weeks of age) were used for the experiment. The experimental procedures were performed in accordance with the animal care guidelines of the National Institutes of Health (NIH) and the Korean Academy of Medical Sciences. The animals were housed under laboratory conditions at a controlled temperature (20±2℃) and maintained under light-dark cycles, each consisting of 12 hr of light and 12 hr of darkness (lighting from 07:00 to 19:00), with food and water being made available ad libitum.
To determine the effect of the radiation dose on cell proliferation and cell death in the hippocampal dentate gyrus, the rats were divided into five groups: the control group, the 0.1 Gy radiation group, the 1 Gy radiation group, the 10 Gy radiation group and the 20 Gy radiation group (n=5 in each group). Each animal was intraperitoneally injected with 50 mg/kg BrdU (Sigma Chemical Co., St. Louis, MO, U.S.A.) 10 min before the radiation and the animals were then sacrificed 2 hr after the BrdU injection.
To determine the effect of the cumulative dose, the rats were divided into nine groups: the control group, the 1-day-0.01 Gy radiation group, the 2-days-0.01 Gy radiation group, the 3-days-0.01 Gy radiation group, the 4-days-0.01 Gy radiation group, the 1-day-0.1 Gy radiation group, the 2-days-0.1 Gy radiation group, the 3-days-0.1 Gy radiation group and the 4-days-0.1 Gy radiation group (n=5 in each group). Each animal was intraperitoneally injected with 50 mg/kg BrdU 10 min before starting the radiation treatment once a day for 4 consecutive days, and the animals were then sacrificed 2 hr after completion of the last BrdU injection.
The animals were first fully anesthetized with Zoletil 50® (10 mg/kg, i.p.; Vibac Laboratories, Carros, France), and next they were transcardially perfused with 50 mM phosphate-buffered saline (PBS); they were then fixed with a freshly prepared solution consisting of 4% paraformaldehyde (PFA) in 100 mM phosphate buffer (PB, pH 7.4). The brains were removed, postfixed in the same fixative overnight and transferred into a 30% sucrose solution for cryoprotection. Coronal sections of 40 µm thickness were made by using a freezing microtome (Leica, Nussloch, Germany).
BrdU immunohistochemistry was used for detecting the newly generated cells in the dentate. An average of 10 sections within the hippocampal region spanning from Bregma -3.30 mm to Bregma -4.16 mm were selected from each brain. The sections were first permeabilized by incubating them in 0.5% Triton X-100 in PBS for 20 min. They were then incubated in 50% formamide-2×standard saline citrate at 65℃ for 2 hr; they were denaturated in 2 N HCl at 37℃ for 30 min and next rinsed twice in 100 mM sodium borate (pH 8.5). Afterwards, the sections were incubated overnight at 4℃ with a BrdU-specific mouse monoclonal antibody (1:600; Boehringer Mannheim, Mannheim, Germany). The sections were then washed three times with PBS and incubated for 1 hr with a biotinylated mouse secondary antibody (1:200; Vector Laboratories, Burlingame, CA, U.S.A.). The sections were then incubated for another 1 hr with a VECTASTAIN® Elite ABC Kit (1:100; Vector Laboratories). For staining, the sections were incubated for 5 min in a reaction mixture that consisted of 0.02% 3,3'-diaminobenzidine with 40 mg/mL nickel chloride (nickel-DAB) and 0.03% H2O2 in 50 mM Tris-HCl (pH 7.6). The sections were then washed three times with PBS and finally mounted onto gelatin-coated slides. The slides were air-dried overnight at room temperature, and the coverslips were mounted using Permount® (Fisher Scientific, Fair Lawn, NJ, U.S.A.).
Ki-67 immunohistochemistry was performed for visualizing the Ki-67 expression. The sections were taken from each brain and incubated overnight with mouse anti-Ki-67 antibody (1:200; Novocastra Laboratories Ltd, U.K.), and then they were incubated for another 1 hr with biotinylated mouse secondary antibody. The bound secondary antibody was then amplified with a VECTASTAIN® Elite ABC Kit (Vector Laboratories). The antibody-biotin-avidin-peroxidase complexes were visualized using nickel-DAB and 0.03% H2O2 in 50 mM Tris-HCl (pH 7.6) for 5 min. The sections were then washed three times with PBS and finally mounted onto gelatin-coated slides.
For the visualization of the apoptotic cell death, TUNEL assay was performed using the In Situ Cell Death Detection Kit (Roche, Mannheim, Germany). Briefly, the sections were post-fixed in ethanol-acetic acid (2:1) and then rinsed. Next, the sections were incubated with proteinase K (100 µg/mL), rinsed and then incubated in 3% H2O2. They were permeabilized with 0.5% Triton X-100, rinsed again and incubated in the TUNEL reaction mixture. The sections were rinsed and finally visualized using converter-POD with nickel-DAB.
The area of the dentate granular layer in the selected region of the hippocampus was measured using Image-Pro Plus software (Media Cybernetics, Silver Spring, MD, U.S.A.). The numbers of BrdU-positive, Ki-67-positive and TUNEL-positive cells in the dentate gyrus were counted hemilaterally and expressed as the number of cells per mm2 of the granular layer. The statistical analysis was performed using one-way ANOVA followed by Duncan's post-hoc test. The results are presented as the mean±standard error of the mean (S.E.M.). Differences were considered significant at p values <0.05.
The numbers of BrdU-positive cells in the dentate gyrus were 44.37±2.25/mm2 in the control group, 55.44±3.68/mm2 in the 0.1 Gy group, 10.02±1.69/mm2 in the 1 Gy group, 1.76±0.37/mm2 in the 10 Gy group and 0.59±0.23/mm2 in the 20 Gy group, respectively. The numbers of Ki-67-positive cells in the dentate gyrus were 119.14±12.54/mm2 in the control group, 164.59±13.58/mm2 in the 0.1 Gy group, 50.20±4.68/mm2 in the 1 Gy group, 13.66±2.49/mm2 in the 10 Gy group and 7.95±1.31/mm2 in the 20 Gy group. The numbers of TUNEL-positive cells in the hippocampal dentate gyrus were 1.31±0.91/mm2 in the control group, 1.16±0.54/mm2 in the 0.1 Gy group, 143.42±13.67/mm2 in the 1 Gy group, 515.18±42.46/mm2 in the 10 Gy group and 624.61±26.01/mm2 in the 20 Gy group (Fig. 1).
The animals were irradiated with 0.01 Gy or 0.1 Gy from 1 to 4 consecutive days. The number of BrdU-positive cells in the hippocampal dentate gyrus was 110.93±11.15/mm2 in the control group, 233.20±13.36/mm2 in the 0.01 Gy group, 349.80±26.35/mm2 in the 0.02 Gy group, 409.79±28.70/mm2 in the 0.03 Gy group, 446.28±40.26/mm2 in the 0.04 Gy group, 287.39±22.22/mm2 in the 0.1 Gy group, 285.92±21.63/mm2 in the 0.2 Gy group, 262.78±23.96/mm2 in the 0.3 Gy group and 254.19±15.27/mm2 in the 0.4 Gy group. The number of Ki-67-positive cells in the hippocampal dentate gyrus was 121.26±7.37/mm2 in the control group, 217.96±27.69/mm2 in the 0.01 Gy group, 266.75±47.28/mm2 in the 0.02 Gy group, 378.29±69.27/mm2 in the 0.03 Gy group, 489.15±63.71/mm2 in the 0.04 Gy group, 301.68±44.84/mm2 in the 0.1 Gy group, 196.68±34.72/mm2 in the 0.2 Gy group, 188.13±31.87/mm2 in the 0.3 Gy group, and 163.10±30.97/mm2 in the 0.4 Gy group. The number of TUNEL-positive cells in the hippocampal dentate gyrus was 3.20±2.17/mm2 in the control group, 2.32±1.51/mm2 in the 0.01 Gy group, 4.09±2.50/mm2 in the 0.02 Gy group, 1.61±0.67/mm2 in the 0.03 Gy group, 1.08±0.76/mm2 in the 0.04 Gy group, 2.26±1.00/mm2 in the 0.1 Gy group, 1.38±0.95/mm2 in the 0.2 Gy group, 3.30±1.13/mm2 in the 0.3 Gy group and 2.10±1.42/mm2 in the 0.4 Gy (Fig. 2).
Neural stem cells reside in the hippocampus and they produce new neurons in all vertebrates that have been studied, including humans (13). Previous studies with animal models have demonstrated that exposure to therapeutic doses of radiation results in an increase in apoptosis (3, 14) and a decrease in cell proliferation within the neurogenic region of the hippocampus (3). Also, a single dose of 20 to 25 Gy cranial irradiation cause delayed deficits in performance in hippocampal-dependent behavioral tasks (15). However, the effect of radiation on neurogenesis seems to be different at low dose levels of irradiation. Sienkiewicz et al. reported that compared with the sham exposed control mice, 0.35 Gy irradiation caused a significant impairment in performance, and exposure at 0.5 Gy resulted in a still larger impairment, but the performance was not significantly affected with irradiation at 0.1 Gy and it was slightly but non-significantly reduced at 0.25 Gy (16). Although the authors suggested there was an association between the dose and behavioral impairment via a linear relationship without a threshold, they were not able to detect any impairment at doses lower than about 0.25 Gy.
In our study, the numbers of Brd-U and Ki-67 positive cells in the dentate gyrus were decreased by a single dose of 1, 10 and 20 Gy irradiation. The numbers of Brd-U and Ki-67 positive cells of the rats sacrificed two hours after 10 Gy irradiation were about 4% and 11% of the control, respectively. Although any direct comparison to other researches was not possible due to the lack of similar experiments, in the research on adult neurogenesis with irradiation, Mizumatsu et al. reported that the number of proliferating cells (Ki-67 positive) was reduced about 90% at 12 hr after doses of 2-10 Gy (2); this showed similar results to our results. Also, in the research of neural precursor-cell dysfunction induced by irradiation, Monje et al. reported that for rats sacrificed two months after a single dose of 10 Gy cranial irradiation, the number of new born cells in the irradiated granular layer and hilus was decreased (17). These results suggest that the acute ablation of cell proliferation induced by radiation occurs from the very beginning and it lasts for at least two months.
In contrast, the numbers of Brd-U and Ki-67 positive cells were increased in the 0.1 Gy irradiation group and it decreased with 1 Gy or more irradiation. The results were consistent with other studies concerning the protective response to a single exposure of radiation of around 0.1 Gy. It has been reported that the protective responses to single exposures tended to be maximally expressed after about 0.1 Gy and there were very little protective response after more than about 0.5 Gy radiation (8, 18, 19). Feinendegen et al. reported that depending on the type of adaptive protection in a given cell system, in most mammalian cells that have been examined so far, the expression of adaptive protection had a maximum above 0.05 Gy and below about 0.2 Gy (5, 8). With these results, low-dose irradiation below 0.1 Gy was thought to enhance cell proliferation in the dentate gyrus of the hippocampus of rats.
The numbers of TUNEL positive cells were dramatically increased with 1 Gy or more irradiation in a dose dependent manner. But the number of TUNEL positive cell was slightly decreased with 0.1 Gy irradiation, which did not reach statistical significance. The present results showed 1 Gy or more irradiation increased neuronal cell death, while 0.1 Gy of irradiation did not exert a significant effect on neuronal cell death. These results are concordant with the reports that the rate of apoptosis in thymocytes with 0.2 Gy or less radiation was lower than that in controls in vitro or in vivo (20, 21). In summary, a single exposure to 0.1 Gy radiation increased neuronal cell proliferation in the dentate gyrus without increasing cell apoptosis.
In the second part of this study, irradiation with a 0.01 Gy/fraction or a 0.1 Gy/fraction from 1 to 4 consecutive days was done to evaluate the effect of the cumulative dose. The number of Brd-U and Ki-67 positive cells was increased with a cumulative dose of 0.01, 0.02, 0.03 and 0.04 Gy. These results showed that with a low dose level (0.01 Gy/fraction), neuronal cell proliferation was increased in a dose dependent manner. But with using the 0.1 Gy/fraction, neuronal cell proliferation did not show a relationship with the cumulative dose. In the mean time, the numbers of TUNEL positive cells were not significantly changed throughout the irradiation schedule, suggesting that at least up to a cumulative dose of 0.4 Gy, the irradiation did not cause any alteration in the level of apoptosis. In summary, 0.01 Gy/fraction irradiation increases cell proliferation as the dose is accumulated without altering cellular apoptosis.
A low dose of ionizing radiation has been shown to enhance cellular resistance with the same effect as a subsequent higher dose. This phenomenon has been called the adaptive response. The biological mechanisms underlying the adaptive response are rather unclear. The mechanism of adaptive responses to low dose radiation is explained by a cell-survival adaptive response and/or a genotoxic adaptive response. The characteristics of a genotoxic adaptive response may differ from a cell-survival adaptive response because the increase in cell survival could not be explained by the increased number of non-aberrant cells. The suggested explanation for the radiation-induced adaptive response is that the production of free radicals from radiation stimulated cells to evolve a repair system for chromosome breaks and in turn, an alteration of the DNA molecule triggers this repair system; frequent activation may increase the general repair capacity, irrespective of the cause of the damage (22, 23). However, in a recent report, Cramers et al. have reported that adaptation in non-dividing cells is not mediated by enhanced rejoining of strand breaks and that any protection against the induction of DNA damage is rather small. They concluded by suggesting that the adaptive response is most likely a reflection of perturbations of cell cycle progression (24). Therefore, we observed the Ki-67 staining pattern, which is known to be strictly correlated with the cell cycle phases (25). The G2-phase cells show fine granular Ki-67 staining in the karyoplasm. In early mitotic cells (pro- and metaphase), an intense perichromosomal Ki-67 staining is observed, but during anaphase and telophase, the Ki-67 antigen rapidly disappears. In resting cells there is no Ki-67 staining. In our present study, the exposure to 0.01 Gy/fraction irradiation for 4 consecutive days resulted in an increased granular staining pattern of the Ki-67-positive cells, suggesting that the early mitotic cells were increased.
Cells exposed to low dose radiation in vitro or in vivo can develop high resistance to subsequent high dose radiation induced gene mutation, DNA damage, and cell death. This phenomenon is termed radiation induced adaptive responses. There are several reports that the adaptive responses occur in neuronal tissues (26). But the process of neurogenesis consists of distinct developmental processes and it depends on a complex microenvironment that involves signaling between multiple cell types, and irradiation could affect any or all of these cells or interactions. So far, there is few reports concerning the mechanism of adaptive responses in dentate gyrus of hippocampus, which is known to have a critical function for cognitive and memory function. Our study showed that the radiation response in the hippocampus was related with the increased number of proliferating cells, but not with the decreased number of cells exhibiting apoptosis. The results support the explanation that the adaptive response in the hippocampus of rats is more likely the reflection of perturbations of cell cycle progression. In the present study, low doses of radiation below 0.1 Gy clearly induced neuronal proliferation in the hippocampus. Although a cause-and-effect relationship has yet to be shown, given the apparent relationship between hippocampal neurogenesis and associated cognitive and memory function, introducing low dose of irradiation may be possible to ameliorate or rescue individuals at risk from many kinds of neuronal damages.
Figures and Tables
Fig. 1
The effect of a single dose on the 5-bromo-2'-deoxyuridine (BrdU), Ki-67, and TUNEL positive cells in the dentate gyrus of rats. The Brd-U positive and Ki-67 positive cells are increased after 0.1 Gy radiations. The change of the number of apoptotic cells is minimal with 0.1 Gy radiation but dramatically increases as the dose increased.

Fig. 2
The effect of cumulative dose on the 5-bromo-2'-deoxyuridine (BrdU), Ki-67 and TUNEL positive cells in the dentate gyrus of rats. The Brd-U positive and Ki-67 positive cells are increased with 0.01 Gy/fraction radiation as the dose increased. But with 0.1 Gy/fraction radiation, Brd-U positive and Ki-67 positive cells show a peak at 0.1 Gy and decrease afterward as the dose increased. The number of apoptotic cells do not show significant alterations with both 0.01 Gy/fraction and 0.1 Gy/fraction radiation although the dose was accumulated. Open circle: 0.01 Gy radiation group. Closed circle: 0.1 Gy radiation group.
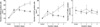
References
1. Ferrer I, Borras D. Effects of X-irradiation on glial cells in the developing rat brain. Int J Radiat Biol. 1994. 66:181–187.


2. Mizumatsu S, Monje ML, Morhardt DR, Rola R, Palmer TD, Fike JR. Extreme sensitivity of adult neurogenesis to low doses of X-irradiation. Cancer Res. 2003. 63:4021–4027.
3. Tada E, Parent JM, Lowenstein DH, Fike JR. X-irradiation causes a prolonged reduction in cell proliferation in the dentate gyrus of adult rats. Neuroscience. 2000. 99:33–41.


5. Feinendegen LE. Evidence for beneficial low level radiation effects and radiation hormesis. Br J Radiol. 2005. 78:3–7.


6. Olivieri G, Bodycote J, Wolff S. Adaptive response of human lymphocytes to low concentrations of radioactive thymidine. Science. 1984. 223:594–597.


7. Wolff S. The adaptive response in radiobiology: evolving insights and implications. Environ Health Perspect. 1998. 106:Suppl 1. 277–283.


8. Feinendegen LE, Loken MK, Booz J, Muhlensiepen H, Sondhaus CA, Bond VP. Cellular mechanisms of protection and repair induced by radiation exposure and their consequences for cell system responses. Stem Cells. 1995. 13:Suppl 1. 7–20.
9. Liu J, Solway K, Messing RO, Sharp FR. Increased neurogenesis in the dentate gyrus after transient global ischemia in gerbils. J Neurosci. 1998. 18:7768–7778.


10. Jin K, Minami M, Lan JQ, Mao XO, Batteur S, Simon RP, Greenberg DA. Neurogenesis in dentate subgranular zone and rostral subventricular zone after focal cerebral ischemia in the rat. Proc Natl Acad Sci USA. 2001. 98:4710–4715.


11. Kang JO, Kim SK, Hong SE, Lee TH, Kim CJ. Low dose radiation overcomes diabetes-induced suppression of hippocampal neuronal cell proliferation in rats. J Korean Med Sci. 2006. 21:500–505.


12. Kee N, Sivalingam S, Boonstra R, Wojtowicz JM. The utility of Ki-67 and BrdU as proliferative markers of adult neurogenesis. J Neurosci Methods. 2002. 115:97–105.


13. Eriksson PS, Perfilieva E, Bjork-Eriksson T, Alborn AM, Nordborg C, Peterson DA, Gage FH. Neurogenesis in the adult human hippocampus. Nat Med. 1998. 4:1313–1317.


14. Peissner W, Kocher M, Treuer H, Gillardon F. Ionizing radiation-induced apoptosis of proliferating stem cells in the dentate gyrus of the adult rat hippocampus. Brain Res Mol Brain Res. 1999. 71:61–68.
15. Hodges H, Katzung N, Sowinski P, Hopewell JW, Wilkinson JH, Bywaters T, Rezvani M. Late behavioural and neuropathological effects of local brain irradiation in the rat. Behav Brain Res. 1998. 91:99–114.


16. Sienkiewicz ZJ, Haylock RG, Saunders RD. Prenatal irradiation and spatial memory in mice: investigation of dose-response relationship. Int J Radiat Biol. 1994. 65:611–618.


17. Monje ML, Mizumatsu S, Fike JR, Palmer TD. Irradiation induces neural precursor-cell dysfunction. Nat Med. 2002. 8:955–962.


18. Shadley JD, Wiencke JK. Induction of the adaptive response by X-rays is dependent on radiation intensity. Int J Radiat Biol. 1989. 56:107–118.


19. Feinendegen LE, Bond VP, Sondhaus CA, Muehlensiepen H. Radiation effects induced by low doses in complex tissue and their relation to cellular adaptive responses. Mutat Res. 1996. 358:199–205.


20. Liu SZ, Zhang YC, Mu Y, Su X, Liu JX. Thymocyte apoptosis in response to low-dose radiation. Mutat Res. 1996. 358:185–191.


21. Shaposhnikova VV, Korystov YN. Thymocyte proliferation and apoptosis induced by ionizing radiation. Scanning Microsc. 1995. 9:1203–1206.
22. Ikushima T, Aritomi H, Morisita J. Radioadaptive response: efficient repair of radiation-induced DNA damage in adapted cells. Mutat Res. 1996. 358:193–198.


23. Johansson L. Hormesis, an update of the present position. Eur J Nucl Med Mol Imaging. 2003. 30:921–933.


24. Cramers P, Atanasova P, Vrolijk H, Darroudi F, van Zeeland AA, Huiskamp R, Mullenders LH, Kleinjans JC. Pre-exposure to low doses: modulation of X-ray-induced dna damage and repair? Radiat Res. 2005. 164(4 Pt 1):383–390.

