Abstract
Neurofibromatosis type 1 (NF1) is one of the most common autosomal dominant disorders in humans. NF1 is caused by mutations in the NF1 gene which consists of 57 exons and encodes a GTPase activating protein (GAP), neurofibromin. To date, more than 640 different NF1 mutations have been identified and registered in the Human Gene Mutation Database (HGMD). In order to assess the NF1 mutational spectrum in Korean NF1 patients, we screened 23 unrelated Korean NF1 patients for mutations in the coding region and splice sites of the NF1 gene. We have identified 21 distinct NF1 mutations in 22 patients. The mutations included 10 single base substitutions (3 missense and 7 nonsense), 10 splice site mutations, and 1 single base deletion. Eight mutations have been previously identified and thirteen mutations were novel. The mutations are evenly distributed across exon 3 through intron 47 of the NF1 gene and no mutational hot spots were found. This analysis revealed a wide spectrum of NF1 mutations in Korean patients. A genotype-phenotype correlation analysis suggests that there is no clear relationship between specific NF1 mutations and clinical features of the disease.
Neurofibromatosis type 1 (NF1) is one of the most common inherited disorders, with an estimated incidence of 1 per 3,500 births. NF1 is caused by mutations in the NF1 gene. The NF1 gene maps to 17q11.2, contains 57 exons spanning approximately 280 kb of genomic DNA, and encodes neurofibromin (1, 2). Neurofibromin, which consists of 2,818 amino acids, is widely expressed in many tissues and acts as a Ras GTPase activating protein (GAP) (3). Two functional regions of neurofibromin have been identified and well-defined, a central GAP related domain (GRD) encoded by exons 20-27 (4, 5) and several cAMP-dependent protein kinase recognition sites encoded by exons 11-17 (6). The NF1 gene exhibits one of the highest mutation rates of any human disorder. To date, more than 640 different NF1 mutations have been found and listed in the Human Gene Mutation Database (HGMD). The majority of them lead to a truncated protein product.
NF1 is clinically characterized by café-au-lait (CAL) spots, neurofibromas, freckling of the axillary or inguinal region, Lisch nodules, optic nerve glioma, and bone dysplasias (7). These features are present in more than 90% of patients at puberty. Further manifestations such as deeply situated and arbitrarily located neurofibromas, plexiform neurofibromas, macrocephaly, short stature, learning difficulties, scoliosis, and pseudarthrosis, as well as certain malignancies, occur less frequently among patients with NF1 (8). NF1 is notable for its extreme phenotypic variability both within and between families. In addition to multiple allelism of the NF1 locus, the influence of modifying gene(s) and the impact of stochastic events have been suggested as causes of this variability (9, 10). However, evidence supporting these hypotheses has not been reported.
A comprehensive analysis of the mutational spectrum might help to elucidate the molecular basis of different NF1 mutations. In Korea, only three cases of NF1 have been previously reported (11, 12). In order to assess the NF1 mutational spectrum in Korean NF1 patients, we performed phenotypic characterization and mutation analysis in 23 unrelated cases. Here, we report the spectrum of NF1 mutations in Korean NF1 patients, and discuss a genotype-phenotype correlation analysis.
Twenty-three NF1 patients of 9 familial and 14 sporadic cases were screened for mutations of the NF1 gene. All subjects were diagnosed to have NF1 based on standard diagnostic criteria (7) at the Genetics Clinic in Ajou University Medical Center between December 2003 and March 2005.
Phenotypic characterization of all subjects was done at the Genetics Clinic in Ajou University Medical Center and is summarized in Table 1. Mutation screening was performed in the Medical Genetics Laboratory, Department of Genetics, University of Alabama at Birmingham, U.S.A. Based on the screening data of the NF1 patients, we performed a detailed mutation analysis of the NF1 gene and further analysis of genotype-phenotype correlations.
Mutation screening was performed as described previously (13). Briefly, genomic DNA was prepared from blood samples of the patients and total RNA was extracted from lymphocytes isolated from the blood samples and amplified in culture. Microsatellite polymorphism markers were analyzed as a first approach to determine whether 2 copies of the NF1 gene were present. If no heterozygous signal was observed for any of these markers, FISH analysis was performed using PAC clones 22 (926B9; 5' NF1) and 13 (1002G3; 3' NF1) to confirm or rule out a total gene deletion. Thereafter, genomic DNA-PCR and RT-PCR were performed to screen splice site mutations and coding region mutations of the NF1 gene, respectively. Sequencing was performed using dye-primer chemistry on an ABI3100 capillary sequencer (Applied Biosystems, Foster City, CA, U.S.A.).
The patients were analyzed using genomic PCR-directed sequencing, microsatellite polymorphism analysis, FISH analysis, and RT-PCR. Several other methods, including heteroduplex analysis (HA), protein truncation test (PTT), and denaturing high performance liquid chromatography (DHPLC), have been used for analysis of NF1 mutations (13, 14) and are useful for high-throughput detection of mutations. However, we used PCR-directed genomic sequencing as our primary technique to detect mutations because it is very accurate and directly identifies changes in gene sequence.
Twenty-three unrelated patients who were clinically diagnosed with NF1 were screened for mutations in the coding region (57 exons) and splice sites of the NF1 gene. Mutations in NF1 were detected in 22 of 23 patients (96%). Mutation analysis revealed a wide spectrum of NF1 mutations in these patients (Table 1). Twenty-one different mutations were identified, which included 10 single base substitutions (3 missense and 7 nonsense), 10 splice site mutations, and 1 single base deletion. Eight mutations (38%) have been previously reported and thirteen mutations (62%) were novel. Only 2 patients shared the same mutation, c.4537C>T, and 20 patients had unique mutations, indicating that no mutational "hot spots" exist in Korean NF1 patients.
The mutations were evenly distributed across exon 3 to intron 47 of the NF1 gene (Fig. 1). The previously characterized mutations detected in this study were: IVS8+1G>A in intron 8, c.1381C>T (R461X) in exon 10, c.2446C>T (R816X) in exon 16, c.3827G>A (R1276Q) in exon 22, c.4537C>T (R1513X) in exon 27, c.6709C>T (R2237X) in exon 36, c.6792C>G (Y2264X) in exon 37, and c.6792C>A (Y2264X) in exon 37. The newly identified mutations were: c.236delT in exon 3, c.532G>T (E178X) in exon 4, c.1642-449A>G in intron 10, c.1733T>C (L578P) in exon 12, c.2033_2034insC in intron 13, c.2252-33_-8del26 in intron 14, c.2851-2A>C in intron 16, IVS21+2-3insT in intron 21, c.4269+1_4269+12del12bp in intron 24, 4861G>A and 4862T>G (V1621R) in exon 28, c.5749+4_+7delAGTA in intron 30, c.5750-?_5943+?del in intron 31, and c.61-?_8315-?del in intron 47.
The majority of these mutations resulted in a truncated or shortened protein product. Only three missense mutations, L578P, R1276Q, and V1621R, do not affect the size of neurofibromin. Two mutations, c.4537 in exon 27 (patients 14 and 15 in Table 1) and c.6792 in exon 37 (patients 20 and 21 in Table 1) were each found in two patients. Two mutations, R1276Q and R1513X, were detected in the GAP related domain (GRD) which has been suggested to be a "hot spot" for NF1 mutations, given its important functional role in modulating Ras activity (14). Another two mutations, L578P and R816X, were observed in the cAMP-dependent protein kinase recognition sites (6).
Phenotypic characterization revealed that the only clinical feature common to all patients was the CAL spots (Table 1). To identify genotype-phenotype associations, we first analyzed six NF1 patients (5 sporadic cases and 1 familial case) who share an identical phenotype, plexiform neurofibromas. Among them, five patients (5, 7, 14, 15, and 20 in Table 1) possessed mutations which caused a splicing error (c.1642-449A>G and c.2033_2034insC) or mutations which produced a truncated neurofibromin (R1513X and Y2264X), but one patient (16 in Table 1) had a mutation which resulted in an amino acid substitution (V1621R). This result indicates that the effects of different mutations are not always distinguishable at the phenotypic level. Despite distinct genotypes, the patients shared the same phenotype, plexiform neurofibroma, suggesting that there is no obvious correlation between the type of mutation and the severity of the disease.
Similarly, patients 14 and 15 carried the same nonsense mutation (R1513X), but had no clinical features in common, with the exception of the CAL spots. In contrast, patients 20 and 21 who possessed the same truncated neurofibromin by mutation of Y2264X showed distinct clinical features with the occurrence of neurofibromas and scoliosis in one patient, and microcephaly and seizures in the other. Our data suggest that there is no clear relationship between specific NF1 mutations and clinical features.
A survey of previously reported NF1 mutations listed in the HGMD revealed that the most frequent type of mutation is a small deletion and the next most frequent type is nucleotide substitution. However, our data indicate that the most frequent mutation type is nucleotide substitution (11 of 22 cases) and followed by splicing error mutations (10 of 21 cases). Only one deletion mutation was identified in our study, suggesting that the genotypic characteristics of Korean NF1 patients may be distinct from that of other populations.
Only three cases of NF1 mutation in Koreans have been previously described (11, 12). Park et al. reported two frame shift mutations (a TGGA insertion at codon 1270 of exon 22 and a one base pair deletion at codon 1398 of exon 24) (11) and one nonsense mutation (R1947X) (12). Recently, another 30 Korean cases were reported at the 29th Symposium of the Korean Society of Medical Genetics (2004), by Lee et al., Department of Clinical Genetics, College of Medicine, Yonsei University, Seoul, Korea. A review of the 55 Korean NF1 genotypes available, including the above 30 cases, showed that most Korean NF1 patients bear unique mutations, with the exception of two mutations, c.4537C>T in exon 27 and c.6709C>T in exon 36. These data indicate that the mutational spectrum in Korean NF1 patients is highly variable.
Two mutations were detected in 3 patients in exons 20-27 which correspond to the GRD of neurofibromin. The GRD is known to be the most important functional domain of the NF1 gene. The predicted GRD in neurofibromin consists of 367 amino acids (1172-1538) and shares homology with GAP family proteins such as p120GAP in yeast and mammals (3). This region of the gene has also been reported as representing a "hot spot" for mutations (15). The crystal structure of the GRD of neurofibromin revealed that three prominent regions in the GRD, the arginine-finger loop, the phenylalanine-leucine-arginine (FLR) region, and the α7/variable loop contain structural fingerprints governing the GAP function of neurofibromin (4, 5). The finger loop is crucial for stabilization of the transition state of the GTPase reaction whose function is controlled by residues proximal to the catalytic arginine (R1276). In the previous report, missense mutations at R1276 in the GRD showed severe phenotypes (16). The substitution, R1276P, was found as an apparently spontaneous mutation in an NF1 patient with malignant schwannona (16). The R1276P mutation slightly reduced the binding affinity to Ras, but resulted in an 8000-fold reduction of GAP-stimulated GTP hydrolysis (17), suggesting that the loss of GAP function is critical for the development of neurofibromatosis. In this study, however, patient 12 in Table 1, with the missense mutation R1276Q, showed mild neurofibromas. It may be that the phenotypic variations between the two patients are caused by the difference in the change of the amino acid residues P and Q.
A putative second hot spot in a possible functional domain upstream of GRD, comprising exons 11-17, has been described (6, 16). The region is rich in cysteine and serine, with three cysteine pairs suggestive of ATP binding sites, as well as three potential cAMP-dependent protein kinase recognition sites. Two mutations, L578P and R816X, have been observed in two of the cAMP-dependent protein kinase recognition sites (6). Further evidence for cAMP signaling being perturbed in NF1 comes from mutations in Drosophila neurofibromin that have been shown to inhibit the cAMP-dependent protein kinase signaling pathway (6, 18).
Many NF1 patients have been genotyped, but little evidence of genotype-phenotype correlation has been observed (19). This may indicate that the phenotypic differences resulting from NF1 allelic heterogeneity are generally small in comparison to other sources of variability. It is also possible, however, that important NF1 genotype-phenotype correlations exist but have not been recognized because of the complexity of the NF1 phenotype and the heterogeneity of pathogenic NF1 mutations (13, 15, 17).
In this study, Korean NF1 patients showed a wide range of phenotypic variations. We found that patients exhibited different disease phenotypes, even though they carried an identical NF1 mutant allele. Two patients (14, 15) shared the same mutation, R1513X, but their clinical features were substantially different. Although they shared the clinical features of CAL spots and plexiform neurofibromas, patient 15 also showed several other NF1 clinical features including subcutaneous plexiform neurofibromas, freckling, Lish nodules, and subcutaneous neurofibromas (Table 1). R1513X is one of the most prevalent NF1 gene mutations (20). One patient with R1513X mutation was characterized by the typical clinical features of CAL spots, axillary freckling, and nodular neurofibromas (14). In contrast, other patients with the R1513X mutation had malignant myeloid disorders (21). These data indicate that the R1513X mutation in the NF1 gene is associated with malignant myeloid disorders as well as NF1. Comparison of phenotypes among the patients with R1513X mutation, in previously reported studies and in this study, reveals that there is no correlation between genotype and phenotype in NF1 patients.
Other common mutations, c.6792C>G and c.6792C>A, caused an alternative splicing event which skipped exon 37 and produced a neurofibromin protein lacking 34 amino acid residues (22). These mutations were found in patients 20 and 21, respectively. Even though these mutations result in the same effect at the protein level, Y2264X, their phenotypes were distinguished by the presence of plexiform neurofibromas, cutaneous neurofibromas, subcutaneous neurofibromas, and scoliosis in the patient 20, versus microcephaly and seizures in the patient 21. One NF1 patient with a Y2264X mutation was reported (23) to have symptomatic optic glioma and multiple clinical features that do not fulfill the NIH criteria (7) for the clinical diagnosis of NF1, while another patient with the same mutation was reported to have the typical clinical features of NF1, CAL spots, axillary freckling, and cutaneous neurofibromas. Analysis of the genotype-phenotype correlation by comparison of clinical features among the above patients and the patients in this study suggests that phenotypic variation in NF1 patients is not correlated with the type of mutations, as reported previously (8-10).
In conclusion, the location and type of mutation within the NF1 gene and its putative effect at the protein level do not indicate a relationship to any specific clinical features of NF1, suggesting that unlinked modifying gene(s) may be involved in the development of particular clinical features of NF1 and that some of the clinical variability that characterizes NF1 may result from association of more than one genetic factor. Recently two candidate modifiers for NF1, GDNF and TLF, have been reported (24, 25). However, there has been no clear evidence to explain the phenotypic variations among patients with an identical mutation.
A larger number of NF1 cases may be required to identify potential genotype-phenotype correlations in Korean patients with NF1. A functional analysis of NF1 gene mutations in specific regions, such as the GRD, may clarify the mechanisms of pathogenesis associated with NF1. This study will contribute to a better understanding the genotypic and phenotypic characteristics of Korean NF1 patients.
Figures and Tables
![]() | Fig. 1Location of the NF1 gene mutations detected in 22 unrelated Korean NF1 patients. The cAMP protein kinase recognition sites (cAMP/PK) spanning exons 11-17 and the GAP related domain (GRD) region spanning exons 20-27 are highlighted. |
Table 1
Summary of clinical features and genotypic characteristics of 23 Korean patients with NF1
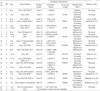
Legend: S/F, Sporadic/Familial; CALs, Café-au-Lait Spots; CNf, Cutaneous Neurofibromas; SCNf, Subcutaneous Neurofibromas; PNf, Plexiform Neurofibromas; LN, Lisch Nodules; F, Freckling; S, Scoliosis; PA, Pseudarthrosis.
Nucleotide numbering is based on the NF1 cDNA sequence (GenBank accession No. M82814) and genomic DNA sequence (GenBank accession No. AY796305).
References
1. Li Y, O'Connell P, Breidenbach HH, Cawthon R, Stevens J, Xu G, Neil S, Robertson M, White R, Viskochil D. Genomic organization of the neurofibromatosis 1 gene (NF1). Genomics. 1995. 25:9–18.


2. Marchuk DA, Saulino AM, Tavakkol R, Swaroop M, Wallace MR, Andersen LB, Mitchell AL, Gutmann DH, Boguski M, Collins FS. cDNA cloning of the type 1 neurofibromatosis gene: complete sequence of the NF1 gene product. Genomics. 1991. 11:931–940.


3. Xu GF, O'Connell P, Viskochil D, Cawthon R, Robertson M, Culver M, Dunn D, Stevens J, Gesteland R, White R. The neurofibromatosis type 1 gene encodes a protein related to GAP. Cell. 1990. 62:599–608.


4. Scheffzek K, Ahmadian MR, Wiesmuller L, Kabsch W, Stege P, Schmitz F, Wittinghofer A. Structural analysis of the GAP-related domain from neurofibromin and its implications. EMBO J. 1998. 17:4313–4327.


5. Ahmadian MR, Kiel C, Stege P, Scheffzek K. Structural fingerprints of the Ras-GTPase activating proteins neurofibromin and p120GAP. J Mol Biol. 2003. 329:699–710.


6. Mattocks C, Baralle D, Tarpey P, Ffrench-Constant C, Bobrow M, Whittaker J. Automated comparative sequence analysis identifies mutations in 89% of NF1 patients and confirms a mutation cluster in exons 11-17 distinct from the GAP related domain. J Med Genet. 2004. 41:e48.


7. Gutmann DH, Aylsworth A, Carey JC, Korf B, Marks J, Pyeritz RE, Rubenstein A, Viskochil D. The diagnostic evaluation and multidisciplinary management of neurofibromatosis 1 and neurofibromatosis 2. JAMA. 1997. 278:51–57.


8. Riccardi VM. Genotype, malleotype, phenotype, and randomness: lessons from neurofibromatosis-1 (NF-1). Am J Hum Genet. 1993. 53:301–304.
9. Easton DF, Ponder MA, Huson SM, Ponder BA. An analysis of variation in expression of neurofibromatosis (NF) type 1 (NF1): evidence for modifying genes. Am J Hum Genet. 1993. 53:305–313.
10. Wiest V, Eisenbarth I, Schmegner C, Krone W, Assum G. Somatic NF1 mutation spectra in a family with neurofibromatosis type 1: toward a theory of genetic modifiers. Hum Mutat. 2003. 22:423–427.
11. Park KC, Choi HO, Han WS, Hwang JH, Park KH, Kim KH, Chung JH, Eun HC. Identification of two novel frame shift mutations of the NF1 gene in Korean patients with neurofibromatosis type 1. J Korean Med Sci. 2000. 15:542–544.
12. Park KC, Choi HO, Park KH, Kim KH, Eun HC. A nonsense mutation at Arg-1947 in the NF1 gene in a case of neurofibromatosis type 1 in a Korean patient. J Hum Genet. 2000. 45:84–85.


13. Messiaen LM, Callens T, Mortier G, Beysen D, Vandenbroucke I, Van Roy N, Speleman F, Paepe AD. Exhaustive mutation analysis of the NF1 gene allows identification of 95% of mutations and reveals a high frequency of unusual splicing defects. Hum Mutat. 2000. 15:541–555.
14. De Luca A, Schirinzi A, Buccino A, Bottillo I, Sinibaldi L, Torrente I, Ciavarella A, Dottorini T, Porciello R, Giustini S, Calvieri S, Dallapiccola B. Novel and recurrent mutations in the NF1 gene in Italian patients with neurofibromatosis type 1. Hum Mutat. 2004. 23:629.
15. Upadhyaya M, Osborn MJ, Maynard J, Kim MR, Tamanoi F, Cooper DN. Mutational and functional analysis of the neurofibromatosis type 1 (NF1) gene. Hum Genet. 1997. 99:88–92.
16. Klose A, Ahmadian MR, Schuelke M, Scheffzek K, Hoffmeyer S, Gewies A, Schmitz F, Kaufmann D, Peters H, Wittinghofer A, Nürnberg P. Selective disactivation of neurofibromin GAP activity in neurofibromatosis type 1. Hum Mol Genet. 1998. 7:1261–1268.


17. Fahsold R, Hoffmeyer S, Mischung C, Gille C, Ehlers C, Kucukceylan N, Abdel-Nour M, Gewies A, Peters H, Kaufmann D, Buske A, Tinschert S, Nürnberg P. Minor lesion mutational spectrum of the entire NF1 gene does not explain its high mutability but points to a functional domain upstream of the GAP-related domain. Am J Hum Genet. 2000. 66:790–818.


18. Guo HF, Tong J, Hannan F, Luo L, Zhong Y. A neurofibromatosis-1-regulated pathway is required for learning in Drosophila. Nature. 2000. 403:895–898.
19. Szudek J, Joe H, Friedman JM. Analysis of intrafamilial phenotypic variation in neurofibromatosis 1 (NF1). Genet Epidemiol. 2002. 23:150–164.


20. Ars E, Kruyer H, Morell M, Pros E, Serra E, Ravella A, Estivill X, Lazaro C. Recurrent mutations in the NF1 gene are common among neurofibromatosis type 1 patients. J Med Genet. 2003. 40:e82.


21. Side L, Taylor B, Cayouette M, Conner E, Thompson P, Luce M, Shannon K. Homozygous inactivation of the NF1 gene in bone marrow cells from children with neurofibromatosis type 1 and malignant myeloid disorders. N Engl J Med. 1997. 336:1713–1720.
22. Messiaen L, Callens T, De Paepe A, Craen M, Mortier G. Characterisation of two different nonsense mutations, C6792A and C6792G, causing skipping of exon 37 in the NF1 gene. Hum Genet. 1997. 101:75–80.


23. Buske A, Gewies A, Lehmann R, Ruther K, Algermissen B, Nurnberg P, Tinschert S. Recurrent NF1 gene mutation in a patient with oligosymptomatic neurofibromatosis type 1 (NF1). Am J Med Genet. 1999. 86:328–330.
24. Bahuau M, Pelet A, Vidaud D, Lamireau T, LeBail B, Munnich A, Vidaud M, Lyonnet S, Lacombe D. GDNF as a candidate modifier in a type 1 neurofibromatosis (NF1) enteric phenotype. J Med Genet. 2001. 38:638–643.


25. Chong JA, Moran MM, Teichmann M, Kaczmarek JS, Roeder R, Clapham DE. TATA-binding protein (TBP)-like factor (TLF) is a functional regulator of transcription: reciprocal regulation of the neurofibromatosis type 1 and c-fos genes by TLF/TRF2 and TBP. Mol Cell Biol. 2005. 25:2632–2643.


26. Maynard J, Krawczak M, Upadhyaya M. Characterization and Significance of nine novel mutation in exon 16 of the neurofibromatosis type I (NF1) gene. Hum Genet. 1997. 99:674–676.
27. Robinson PN, Boddrich A, Peters H, Tinschert S, Buske A, Kaufmann D, Numberg P. Two recurrent nonsense mutations and a 4bp deletion in a quasi-symmetric element in exon 37 of the NF1 gene. Hum Genet. 1995. 96:95–98.