Abstract
Recently, the rearrangement of RET proto-oncogene has been reported to be the most common genetic change in papillary thyroid carcinoma (PTC). However, its prevalence has been reported variably and its relation to clinical outcome has been controversial. The characteristic nuclear features of PTC usually render the diagnosis, but problem arises with equivocal cytologic features that are present focally. Although there remains some controversy, CK19 has been reported to be a useful ancillary tool for diagnosis of PTC. To evaluate the expression rate of RET/PTC rearrangement and CK19 in PTCs in a Korean population, we studied 115 papillary thyroid carcinomas in 3 mm-core tissue microarray based immunohistochemical analysis. The prevalence of Ret protein expression was 62.6% and the CK19 immunoreactivity was 80.9%. There was no statistically significant association between the Ret positivity and CK19 immunoreactivity, although the percent agreement of the two was relatively high. The clinicopathological variables did not correlate with the expression of Ret. In conclusion, the prevalence of Ret protein expression and its clinicopathological implications in a Korean population are not much different from those reported in previous studies. However, its detection via immunohistochemistry can be a useful diagnostic tool for diagnosing papillary thyroid carcinoma in conjunction with CK19.
Papillary thyroid carcinoma (PTC) is the most common type of thyroid malignancy (1, 2) and several genes have been implicated in the carcinogenesis of PTC, including TP53, p21, met, trk and ras (3). Recently, however, the rearrangement of RET proto-oncogene, which is normally expressed in neural crest-derived tissues but not in thyroid follicular cells, has been reported to be the most common genetic change in the development of PTC (4). The RET proto-oncogene maps to the long arm of chromosome 10 and encodes a cell membrane-bound receptor tyrosine kinase (5). When the tyrosine kinase encoding domain of the RET proto-oncogene undergoes fusion with the 5'-terminal region of another gene that is constitutively expressed, it results in activation of an oncogene designated RET/PTC. As a consequence, the products of the chimeric RET/PTC oncogenes lead to the relocation of the ret tyrosine kinase domain from the membrane to the cytoplasm and display constitutive tyrosine-kinase activity by autophosphorylation (6-8).
Such rearrangements of the RET proto-oncogene have only been found in thyroid gland tumors of the papillary histotype. However, its prevalence has been reported variably in different geographical regions (9-12), and its correlation with clinical outcome has been controversial (2, 6, 13-15).
The purpose of this current study is first to evaluate the expression rate of Ret protein in a large series of classic PTCs in a Korean population using immunohistochemistry in tissue microarray and explore its possibility as a reliable prognostic factor by analyzing its correlation with known prognostic factors. Secondly, this study is intended to clarify a more specific role of Ret antibody as an ancillary tool for diagnosing PTC in conjunction with cytokeratin (CK) 19.
Recent studies of CK19 expression in thyroid neoplasms have demonstrated that various low and high molecular-weight CKs in general are expressed differentially in PTCs (16, 17). In particular, CK19 is reported to be limited to PTCs, thus favoring the diagnosis of PTC in all its variant patterns (18). Sahoo et al. has reported that immunoreactivity for CK19 is not specific for PTC, although the extent and intensity of staining are significantly greater in PTC than in follicular adenoma (19). However, most of the previous studies have proposed CK19 to be a useful immunohistochemical marker to distinguish PTC from other benign and malignant follicular lesions (17, 20, 21).
The surgical pathology files of Department of Pathology in Yonsei University College of Medicine in Seoul, Korea, in the year 2001 were searched for 'papillary carcinoma' in thyroid. After review of the search results, a consecutive series of 115 classic PTCs were selected for the study. All variants including follicular variant were excluded to maintain the reproducibility and the representativeness of the results. Clinical data were obtained from the medical records.
The slides of 115 cases were reviewed with special attention to the diagnostic nuclear features of PTC, i.e. nuclear grooves, intranuclear inclusions and nuclear clearing (1), and papillary structures to identify representative areas of the specimen for tissue microarray. From these defined areas core biopsies were taken with a precision instrument. Tissue cores with a diameter of 3 mm from each specimen were punched and arrayed on a recipient paraffin block. 4-µm sections of these tissue array blocks were cut and used for immunohistochemical analysis (Fig. 1, 2). Normal thyroid tissue distant from the i) tumor area, ii) adenomatous nodule, and iii) inflammatory cell infiltrates were obtained from each specimen and arrayed. These arrayed normal tissues served as baseline controls.
Sections from tissue arrays were deparaffinized in xylene, rehydrated in graded alcohols, and processed using the labeled streptavidin-biotin-peroxidase method. Briefly, sections were submitted to antigen retrieval for 15 min in 44% formic acid for Ret and in 0.4% pepsin for CK19, both at room temperature. Slides were subsequently incubated in 10% normal blocking serum for 30 min. They were then incubated overnight at 4℃ in appropriately diluted primary antibody. Rabbit polyclonal anti-Ret (1:200; Santa Cruz Biotechnology, Santa Cruz, CA, U.S.A.) antibody and mouse monoclonal anti-CK19 (1:75; Biomeda, Hayward, CA, U.S.A.) antibody were used. After washing with Tris buffer, sections were incubated with biotin-labelled secondary antibodies and then with streptavidin-horseradish peroxidase using the DAKO LSAB kit (DAKO, Carpinteria, CA, U.S.A.) at room temperature for 30 min for each step. Nova red (Vector Laboratory, Burlingame, CA, U.S.A.) was used as the chromogen and hematoxylin as the nuclear counterstain. This procedure was performed for all antibodies under study. The sections were also stained with antibodies to thyroglobulin (1:500, DAKO, Glostrup, Denmark), thyroid transcription factor-1 (1:100, DAKO, Glostrup, Denmark), and calcitonin (1:200, DAKO, Glostrup, Denmark) to validate the follicular cell origin of each case. Cases with thyroglobulin and thyroid transcription factor-1 positivity and calcitonin negativity were selected only.
Diffuse cytoplasmic staining of the tumor cells was defined as positive immunoreactivity for Ret and CK19. Informations on regional lymph node metastasis, perithyroidal extension of the tumor, multiplicity at the time of diagnosis, and size of the tumor were assessed from the surgical pathology reports.
Summary statistics were obtained using established methods. Associations between Ret immunoreactivity and each variable, i.e. lymph node metastasis, perithyroidal extension and multiplicity, were evaluated using the chi-square test. Kappa statistics was performed for the evaluation of percent agreement, the extent to which Ret immunoreactivity and CK19 immunoreactivity concur.
In all statistical analyses, a two-tailed p value ≤0.05 was considered statistically significant. All analyses were performed using SPSS for Windows statistical software (Version 10.0).
The male to female ratio of the patient group was 1:10.5, which was similar in both Ret positive and negative groups. The average age at surgery was 44.5 yr, with a range from 19-76 yr (Table 1). Twenty-nine patients had multifocal disease, and 84 patients had single tumors. Information on the multiplicity of the tumor at the time of the surgery was not available in two patients. The average tumor size was 1.8 cm with a range from 0.5 cm to 6.0 cm. The size of the tumor was not mentioned in the surgical pathology reports in 6 patients. Of the remaining 109 tumors, 37 cases were less than 1 cm, 68 were in the range of 1-4 cm, and only 4 cases were more than 4 cm (Table 2). Eighty-five patients underwent regional lymph node dissection, of which 63 had lymph node metastasis. Perithyroidal extension was present in 84 cases and 29 cases had tumors confined to the thyroid parenchyma (Table 2). The presence of perithyroidal extension was not evaluable in two cases, for perithyroidal soft tissue was not included within the specimen.
A total of 72 cases (62.6%) of 115 papillary thyroid carcinomas expressed Ret protein. The immunohistochemical detection of Ret protein was consistently observed in the cytoplasm of the tumor cells in more than 30% of the tissue core for each case. Ret protein expression was absent in the normal thyroid tissues (Fig. 3).
The Ret protein expression rate was not different in those groups with and without regional lymph node metastasis, perithyroidal extension, and multifocal disease (Table 2). Furthermore, age and sex of the patient group (Table 1), and the size of the tumor did not influence the expression of Ret (Table 2).
Ninety-three PTCs (80.9%) showed diffuse cytoplasmic staining of CK19 (Table 3, Fig. 4). As with the Ret protein expression, CK19 immunoreactivity also had no statistically significant correlation with the aforementioned clinicopathological variables. Of the 115 cases, 7 cases were in lack of normal thyroid tissue in the specimen and 12 out of the 108 matched normal thyroid tissue showed CK19 immunoreactivity in the follicular epithelial cells.
Of the 115 papillary carcinomas, 62 cases (53.9%) showed positivity for both CK19 and Ret, and twelve cases (10.4%) showed negative results for both CK19 and Ret. The percent agreement of the two antibodies in classic PTC was relatively high, however, it had no statistical significance (κ=0.16, p=0.06) (Table 3).
The RET proto-oncogene has been identified to be responsible for the inherited cancer syndrome MEN 2, and also involved in the molecular pathogenesis of sporadic medullary thyroid carcinomas, PTCs, and Hirschsprung's disease (22).
In the pathogenesis of PTC, RET activation has been hypothesized to constitute a specific genetic event and to account for the characteristic nuclear changes of PTC (23). Sugg et al. (14). identified RET/PTC rearrangements in the majority of occult papillary microcarcinomas and lower percentage of rearrangements in clinically manifest tumors, suggesting a significant role of RET/PTC rearrangements in the initiation of PTC. However, the exact role of RET/PTC in the development of PTC remains unclear and the prevalence of RET/PTC rearrangement is ill-defined, varying widely from 2.5-34.5% among patient series from different geographical regions (9-12). The existence of geographic variability is shown by comparison of the prevalence of RET/PTC activation in different studies of sporadic PTCs, all of which have used reverse transcriptase polymerase chain reaction (24). Santoro et al. found 11% in the French samples, 33% in the Italian samples, and 17% in the American samples (6). These discordant results may be due in part to the different methods and sensitivity of the techniques used, as well as the influence of environmental factors such as ionizing radiation exposure. There have been a few published data in Korea prior to the present study. Park et al. found no RET/PTC-1, -2, or -3 rearrangement by RT-PCR in 24 cases of PTC in a Korean population (25), whereas Chung et al. reported a prevalence of 12.9% in Korean PTCs (26). The latter authors found four out of thirty-one PTCs to be positive for RET/PTC rearrangement by RT-PCR, two of which were positive for RET/PTC-2 and the remaining two were positive for RET/PTC-3, and concluded that the prevalence of RET/PTC rearrangement in a Korean population is not different from that in other Western countries. Similarly, Chung KW et al. found RET/PTC rearrangement by RT-PCR in only 2 (9.1%) of the 22 patients (27). Although the method of detection was different, the frequency of RET/PTC expression in our study was higher than that in those prior studies. By immunohistochemistry, we found 72 cases out of 115 classic PTCs (62.6%) to be positive for Ret protein expression, which is also in accord with previous results in Western countries employing immunohistochemistry as the method of detection (28).
The immunohistochemical analysis on 3 mm-core tissue microarray in our study can be reliably used to overcome the problem of tumor heterogeneity in protein expression throughout the entire tumor specimen. According to the recently published data on validation of tissue microarrays for immunohistochemical profiling of cancer specimens by Hoos et al. (29), the concordance of data between full tissue sections and tissue microarray was the highest with triplicate 0.6 mm cores (96-98%) when compared with one and two 0.6 mm tissue cores. However, even with the one core analysis, the nonconcordance rate was only 9.4-11.4% (29). When the larger size of our tissue core and the relatively smaller size of PTC in general are taken into consideration, the validity of our immunohistochemical analysis on tissue microarray need not be in doubt.
Immunohistochemical staining using antibodies against the Ret tyrosine kinase domain for the detection of RET/PTC in PTC is based on the assumption that since Ret is not expressed in thyroid follicular cells lacking RET/PTC activation, negative Ret-tyrosine kinase immunoreactivity is consistent with lack of RET/PTC rearrangement (30). Furthermore, a good correlation has been shown between immunohistochemical reactivity for Ret-tyrosine kinase and RT-PCR (13, 14). However, the discrepancy in results between groups employing RT-PCR/in situ hybridization and those using immunohistochemistry may be explained by the existence of wild-type RET oncogene. In addition to RET/PTC 1, 2, and 3 and their variants, it is probable that wild-type RET oncogene activation plays a role in the development of PTC. The fact that positive immunoreactivity for RET oncogene not only detects the product of RET/PTC oncogenes, but also the products of wild-type RET may account for the high rate of Ret protein immunoreactivity.
Many attempts have been made to correlate the presence of RET/PTC activation with the clinical parameters of human PTCs. Our results demonstrate that the Ret protein expression is not influenced by any of the clinicopathologic parameters, i.e. age, sex, size of the tumor, multiplicity, lymph node metastasis, and perithyroidal extension. Jhiang et al. proposed that RET/PTC might be associated with distant metastatic disease, as 2 of 4 (50%) tumors that had distant metastases expressed RET/PTC rearrangements compared to 4 of 32 (12%) tumors that did not metastasize (11). Miki et al. found a higher incidence of RET/PTC expression in cases with extrathyroidal invasion than in those without invasion by immunohistochemistry (68% vs. 20%, p<0.01), suggesting that RET/PTC may be related to the local invasion of PTCs (15). However, other investigators have shown that RET/PTC is more frequently detected in smaller, slow growing, and less aggressive PTCs, proposing that RET/PTC may serve as a marker for favorable prognosis (31, 32). It has also been suggested that RET/PTC positivity correlates with early lymph node spread but lower metastatic potential (33). This discrepancy may be explained by the differences in screening methods, sample preparation, genetic background of patients, and environmental factors. Thus, it is difficult to compare reports that have studied different patient populations and used different approaches to detect RET/PTC rearrangements. Also, it is yet to be determined whether the clinical behavior of human PTC is affected by differences in RET/PTC expression levels, and/or the different forms of RET/PTC. It is conceivable that signaling pathways perturbed by RET/PTC activation in thyroid cells could be overcome by other factors, if RET/PTC expression occurs at a low level. Larger patient numbers, longer follow-up times and employment of molecular pathologic methods may provide additional information in future studies.
Although we found no prognostic implications of Ret protein expression in PTCs in a Korean population, considering its relatively high expression rate detected by immunohistochemistry we explored its potential role as an ancillary tool in the diagnosis of PTC. We analyzed the concordance rate with CK19, which is reported to be reactive in most PTCs and used as a useful ancillary diagnostic marker (16, 17, 20). The findings of our study showed 80.9% positivity to CK19 in the 115 classic PTCs, which are in corroboration of previous reports that CK19 immunohistochemistry is a useful ancillary tool for diagnosing PTC (19). Cheung et al. have reported that CK19 and Ret antibody comprise an excellent diagnostic panel for PTC along with HBME-1 (28). However, they did not show the percent agreement of the two antibodies in immunoreactivity. We have found 62 (53.9%) out of 115 PTCs to be positive for both Ret and CK19, implicating a relatively high percent agreement of the two antibodies in diagnosing PTC. However, it was statistically not significant (p=0.06, κ=0.16), due in part to comparatively high percentage of positive immunoreactivity for CK19.
Although none of the clinicopathological parameters showed any correlation with Ret protein expression in our cohort of 115 classic PTCs, RET/PTC oncogene activation is a marker for PTC with potentially useful applications to the diagnosis of clinically suspicious thyroid lesions with questionable cytologic features of PTC as well as to the follow-up of patients.
Figures and Tables
Fig. 1
(A) 4-µm section of tissue microarray stained with H&E, showing 3 mm cores of papillary thyroid carcinomas and matched normal thyroid tissues. (B) Immunohistochemical stain for Ret antibody. (C) Immunohistochemical stain for CK19.
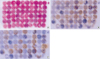
Fig. 2
H&E sections of papillary carcinomas and normal thyroid tissue in the tissue microarray. (A) Papillary carcinoma showing papillary structure (×200) and (B) unequivocal nuclear features (×400). (C) Normal thyroid tissue (×100).
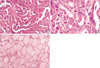
Fig. 3
(A) Diffuse cytoplasmic staining of the Ret antibody in the tumor tissue (×200). (B) No immunoreactivity in the matched normal thyroid tissue (×100).
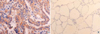
Fig. 4
(A) Diffuse cytoplasmic staining of the tumor cells for CK19 (×200). (B) No immunoreactivity in the matched normal thyroid tissue (×100).
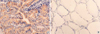
References
1. Rosai J, Carcangiu ML, DeLellis RA. Tumors of the thyroid gland. Atlas of Tumor Pathology, Third Series, Fascicle 5. 1992. Washington, DC: Armed Forces Institute of Pathology;65–121.
2. Kjellman P, Learoyd DL, Messina M, Weber G, Hoog A, Wallin G, Larson C, Robinson BG, Zedenius J. Expression of the RET protooncogene in papillary thyroid carcinoma and its correlation with clinical outcome. Br J Surg. 2001. 88:557–563.


3. Belfiore A, Gangemi P, Costantino A, Russo G, Santonocito G, Ippolito O, DiRenzo MF, Comoglio P, Fiumara A, Vigneri R. Negative/low expression of the Met/hepatocyte growth factor receptor identifies papillary thyroid carcinomas with high risk of distant metastases. J Clin Endocrinol Metab. 1997. 82:2322–2328.


4. Basolo F, Molinaro E, Agate L, Pinchera A, Pollina L, Chiappetta G, Monaco C, Santoro M, Fusco A, Miccoli P, Elisei R, Capezzone M, Pacini F. RET protein expression has no prognostic impact on the long-term outcome of papillary thyroid carcinoma. Eur J Endocrinol. 2001. 145:599–604.


5. Pierotti MA, Bongarzone I, Borrello MG, Greco A, Pilotti S, Sozzi G. Cytogenetics and molecular genetics of carcinomas arising from thyroid epithelial follicular cells. Genes Chromosomes Cancer. 1996. 16:1–14.


6. Santoro M, Carlomagno F, Hay ID, Hermann MA, Grieco M, Melillo R, Pierotti MA, Bongarzone I, Della Porta G, Berger N. Ret oncogene activation in thyroid neoplasms is restricted to the papillary cancer subtype. J Clin Invest. 1992. 89:1517–1522.
7. Grieco M, Santoro M, Berlingieri MT, Melillo RM, Donghi R, Bongarzone I, Pierotti MA, Della Porta G, Fusco A, Vecchio G. PTC is a novel rearranged form of the ret proto-oncogene and is frequently detected in vivo in human thyroid papillary carcinomas. Cell. 1990. 60:557–563.


8. Pierotti MA, Santoro M, Jenkins RB, Sozzi G, Bongarzone I, Grieco M, Monzini N, Miozzo M, Herrmann MA, Fusco A, Hay ID, Della Porta G, Vecchio G. Characterization of an inversion on the long arm of chromosome 10 juxtaposing D10S170 and RET and creating the oncogenic sequence RET/PTC. Proc Natl Acad Sci USA. 1992. 89:1616–1620.


9. Lee CH, Hsu LS, Chi CW, Chen GD, Yang AH, Chen JY. High frequency of rearrangement of the RET proto-oncogene (RET/PTC) in Chinese papillary thyroid carcinomas. J Clin Endocrinol Metab. 1998. 83:1629–1632.


10. Wajjwalku W, Nakamura S, Hasegawa Y, Miyazaki K, Satoh Y, Funahashi H, Matsuyama M, Takahashi M. Low frequency of rearrangements of the ret and trk proto-oncogenes in Japanese thyroid papillary carcinomas. Jpn J Cancer Res. 1992. 83:671–675.
11. Jhiang SM, Caruso DR, Gilmore E, Ishizaka Y, Tahira T, Nagao M, Chiu IM, Mazzaferri EL. Detection of the PTC/retTPC oncogene in human thyroid cancers. Oncogene. 1992. 7:1331–1337.
12. Zou M, Shi Y, Farid NR. Low rate of ret proto-oncogene activation (PTC/retTPC) in papillary thyroid carcinomas from Saudi Arabia. Cancer. 1994. 73:176–180.


13. Viglietto G, Chiappetta G, Martinez-Tello FJ, Fukunaga FH, Tallini G, Rigopoulou D, Visconti R, Mastro A, Santoro M, Fusco A. RET/PTC oncogene activation is an early event in thyroid carcinogenesis. Oncogene. 1995. 11:1207–1210.
14. Sugg SL, Zheng L, Rosen IB, Freeman JL, Ezzat S, Asa SL. ret/PTC-1, -2, and -3 oncogene rearrangements in human thyroid carcinomas: implications for metastatic potential? J Clin Endocrinol Metab. 1996. 81:3360–3365.


15. Miki H, Kitaichi M, Masuda E, Komaki K, Yamamoto Y, Monden Y. ret/PTC expression may be associated with local invasion of thyroid papillary carcinoma. J Surg Oncol. 1999. 71:76–81.


16. Raphael SJ, McKeown-Eyssen G, Asa SL. High-molecular weight cytokeratin and cytokeratin-19 in the diagnosis of thyroid tumors. Mod Pathol. 1994. 7:295–300.
17. Schelfhout LJ, Van Muijen GN, Fleuren GJ. Expression of keratin 19 distinguishes papillary thyroid carcinoma from follicular carcinomas and follicular thyroid adenoma. Am J Clin Pathol. 1989. 92:654–658.
18. Beesley MF, McLaren KM. Cytokeratin 19 and galectin-3 immunohistochemistry in the differential diagnosis of solitary thyroid nodules. Histopathology. 2002. 41:236–243.


19. Sahoo S, Hoda SA, Rosai J, DeLellis RA. Cytokeratin 19 immunoreactivity in the diagnosis of papillary thyroid carcinoma: a note of caution. Am J Clin Pathol. 2001. 116:696–702.
20. Miettinen M, Kovatich AJ, Karkkainene P. Keratin subsets in papillary and follicular thyroid lesion: a paraffin section analysis with diagnostic implications. Virchows Arch. 1997. 431:407–413.
21. Baloch ZW, Abraham S, Roberts S, LiVolsi VA. Differential expression of cytokeratins in follicular variant of papillary carcinoma: an immunohistochemical study and its diagnostic utility. Hum Pathol. 1999. 30:1166–1171.


22. Komminoth P. The RET proto-oncogene in medullary and papillary thyroid carcinoma. Molecular features, pathophysiology and clinical implications. Virchows Arch. 1997. 431:1–9.
23. Fischer AH, Bond JA, Taysavang P, Battles OE, Wynford-Thomas D. Papillary thyroid carcinoma oncogene (RET/PTC) alters the nuclear envelope and chromatin structure. Am J Pathol. 1998. 153:1443–1450.


24. Motomura T, Nikiforov YE, Namba H, Ashizawa K, Nagataki S, Yamashita S, Fagin JA. RET rearrangements in Japanese pediatric and adult papillary thyroid cancers. Thyroid. 1998. 8:485–489.


25. Park KY, Koh JM, Kim YI, Park HJ, Gong G, Hong SJ, Ahn IM. Prevalences of Gs alpha, ras, p53 mutations and ret/PTC rearrangement in differentiated thyroid tumors in a Korean population. Clin Endocrinol. 1998. 49:317–323.
26. Chung JH, Hahm JR, Min YK, Lee MS, Lee MK, Kim KW, Nam SJ, Yang JH, Ree HJ. Detection of RET/PTC oncogene rearrangements in Korean papillary thyroid carcinomas. Thyroid. 1999. 9:1237–1243.


27. Chung KW, Chang MC, Noh DY, Oh SK, Choe KJ, Youn YK. RET oncogene expression of papillary thyroid carcinoma in Korea. Surg Today. 2004. 34:485–492.


28. Cheung CC, Ezzat S, Freeman JL, Rosen IB, Asa SL. Immunohistochemical diagnosis of papillary thyroid carcinoma. Mod Pathol. 2001. 14:338–342.


29. Hoos A, Urist MJ, Stojadinovic A, Mastorides S, Dudas M, Kuo D, Brennan MF, Lewis JJ, Cordon-Cardo C. Validation of tissue microarrays for immunohistochemical profiling of cancer specimens using the example of human fibroblastic tumors. Am J Pathol. 2001. 158:1245–1251.


30. Chiappetta G, Hui P, Santoro M. RET/PTC oncogene activation and assessment of clonality in thyroid nodules with minimal cytologic evidence of papillary carcinoma. Lab Invest. 2001. 81:75A.
31. Soares P, Fonseca E, Wynford-Thomas D, Sobrinho-Simoes M. Sporadic ret-rearranged papillary carcinoma of the thyroid: a subset of slow growing, less aggressive thyroid neoplasms? J Pathol. 1998. 185:71–78.
32. Tallini G, Santoro M, Helie M, Carlomagno F, Salvatore G, Chiappetta G, Carcangiu ML, Fusco A. RET/PTC oncogene activation defines a subset of papillary thyroid carcinomas lacking evidence of progression to poorly differentiated or undifferentiated tumor phenotypes. Clin Cancer Res. 1998. 4:287–294.
33. Mayr B, Goretzki P, Ruschoff J, Dralle H. Ret/PTC-1, -2, and -3 oncogene rearrangements in human thyroid carcinomas: implications for metastatic potential [Letter]? J Clin Endocrinol Metab. 1997. 82:1306–1307.