Abstract
This study was undertaken to compare renal damage, as determined by serum creatinine and degree of apoptosis, caused by iodinated contrast or gadolinium in an acute renal failure (ARF) rat model. Rats were divided into three groups; controls (n=3), a CT contrast medium group (n=9), and an MR contrast medium group (n=9). The CT and MR groups were further subdivided into three groups, namely, low, standard, and high dose subgroups. Renal function was evaluated by determining serum creatinine levels; before ARF, and 48 hr after ARF and contrast administration. Apoptosis was assayed by terminal deoxynucleotidyl transferasemediated dUTP nick-end labeling (TUNEL). No significant creatinine level differences were observed between the CT and MR groups (p=0.116). Degrees of apoptosis in the renal cortex and medulla were more severe in the CT contrast medium group than in the control or MR contrast medium group (p<0.05). The study shows that CT contrast medium did not aggravate renal function more so than MR contrast medium in this ARF rat model. However, apoptosis examination in the renal cortex and medulla indicated that CT contrast medium induced more severe apoptosis than MR contrast medium (p<0.05). We conclude that CT contrast medium can be used for renal imaging studies when subjects are well hydrated and preventive medication is administered.
Acute renal failure (ARF) caused by ischemia or nephrotoxins involves both vascular and tubular events, and vascular ischemia is considered a major contributor to radiocontrast-induced nephropathy (RCIN) (1). Direct tubular toxicity caused by contrast medium has also been postulated to have a role in the induction of RCIN (1). Moreover, ARF following contrast administration for enhanced imaging is an infrequent but serious imaging-associated complication.
Two forms of cell death occur in ischemic renal injury, i.e., apoptosis and necrosis. Renal tubular epithelial cell injury resulting from renal ischemia is generally regarded to be the result of necrotic cell death. And, recently it has become clear that apoptosis has an important role in renal tubular injury (2, 3). Thus, apoptosis is being increasingly recognized a major mode of cell damage after ischemic injury to the kidney (4). Moreover, therapeutic interventions that inhibit or promote the apoptosis of renal tubular cells have the potential to minimize renal dysfunction and accelerate recovery after ARF (5, 6). The degree of renal apoptosis is a reflection of renal damage caused by renal injury and is dependent on both the nature and the severity of an insult (5).
Computed tomographic (CT) contrast medium administration is avoided in cases of poor renal function, especially in patients with an ARF status, whereas magnetic resonance (MR) imaging is used for renal imaging in both patients and experimental animals, especially in those with an ARF status (7-9). Thus, CT scanning is usually performed without contrast medium in patients with renal disease or ARF, because the CT contrast medium aggravates renal function and occasionally causes RCIN. On the other hand, MR imaging is safe in renal disease patients and can also be used to evaluate dynamic physiologic processes (9, 10). We hypothesized that a CT contrast medium injection in an ARF model would not aggravate renal damage more than MR contrast medium, and that might offer a means of obtaining good renal images.
Thus, we undertook this study is to compare the renal damage induced by iodinated contrast and gadolinium in an ARF rat model by monitoring serum creatinine levels and degrees of apoptosis.
Animal experiments were conducted according to the National Institute of Health's Guide for the Care and use of Laboratory Animals (NIH publication No. 80-23, revised in 1996). The acute renal failure rat model was constructed as described previously (11). Briefly, male and non-pregnant female Sprague-Dawley rats, weighing 250-300 g, were obtained from our breeding colony. Abdominal cavities were opened via a midline incision after anesthetization with a 0.1 mL/100 mg mixture of ketamine and xylazine [9 ketamine (30 mg/kg of body weight, I.M.): 1 xylazine (5 mg/kg of body weight, I.M.)]. Both renal pedicles were then exposed and cleaned by blunt dissection. Microvascular clamps were placed on both the renal artery and vein to completely block renal blood flow. Core body temperature was maintained at 37℃ by placing animals on a homeothermic table and was monitored with a temperature-sensing rectal probe. A preliminary experiment indicated that renal injury induced by occlusion with reperfusion after 45 min was optimal. Thus, after 45 min, clamps were removed and blood flow reestablished (12). If reperfusion was found by visual inspection to be incomplete, the experiment was terminated and particular animal sacrificed. Control animals were administered the same anesthetics and underwent the same midline an abdominal incisions, and had both renal arteries and veins cleaned and only placed in contact with microvascular clamps for 45 min before the incision was closed (11). After completing surgery and anesthetic recovery, animals were returned to their metabolic cages and fed a normal diet. All rats were sacrificed 96 hr after ARF using a 0.4 mL/100 mg mixture of ketamine and xylazine [9 ketamine (30 mg/kg of body weight, I.M.): 1 xylazine (5 mg/kg of body weight, I.M.)].
ARF was defined as a two-fold increase in creatinine level from pre-ARF to 48 hr after ARF. Renal function changes were assessed by measuring serum creatinine concentrations at each experimental time point (pre ARF, post 48 hr ARF, and 48 hr after contrast injection in ARF). Creatinine concentrations were measured using an Autoanalyzer (Hitachi 747, Tokyo, Japan) and blood sampled from a rat tail vein using a 24-gauge intravenous catheter (Green-Cross Co., Yong-In, Korea) under same anesthesia. Contrast was injected via a tail vein using a 24-guage intravenous catheter. Blood sampling was performed before ARF, 48 hr after ARF, and then 48 hr after contrast injection in ARF (96 hr after ARF).
Rats were divided into a control group (n=3), a CT group (n=9), and an MR group (n=9). The CT and MR groups were further subdivided into three subgroups based on clinical dosage, i.e., low-dose (CT: 0.5 mL/kg=0.15 g iodine/kg, MR: 0.2 mL/kg=0.1 mM/kg), standard (CT: 2 mL/kg=0.6 g iodine/kg, MR: 0.8 mL/kg=0.4 mM/kg), and high-dose (CT: 8 mL/kg=2.4 g iodine/kg, MR: 3.2 mL/kg=1.6 mM/kg) (7). The contrast medium used was Ultravist® (Korea Schering, Seoul, Korea) in the CT group and Magnevist® (Gd-DTPA, Schering AG, Allemagne, Germany) in the MR group.
At the end of the study, a midline incision was made in the abdomen of each rat to expose of the aorta and inferior vena cava. For both kidneys in each animal supra- and infra-renal abdominal aortas were clamped using microvascular clamps, inferior vena cava were dissected, and kidneys were perfused with phosphate buffered saline through the aorta. Both kidneys were removed, fixed with formalin for two hours, and embedded in paraffin. Samples were washed for 3×5 min in xylene, 2×5 min in absolute ethanol, once in 95% ethanol for 3 min, once in 70% ethanol for 3 min, and once in phosphate buffered saline for 5 min. Tissues were then pretreated with freshly diluted proteinase K (Proteinase K, Dako) for 15 min at room temperature on the slide, and endogenous peroxidase were quenched by treating with 3% hydrogen peroxide in phosphate buffered saline 5 min. Equilibration buffer was then applied to slides, which were then incubated with terminal deoxynucleotidyl transferase for 1 hr at 37℃. Slide were then placed in the anti-digoxigenin peroxidase conjugate for 30 min at room temperature. To develop color, sufficient peroxidase substrate was applied to specimens, which were then counterstained with 0.5% methyl green for 10 min and mounted. Apoptosis was detected using the Apoptag Plus Peroxidase In Situ Apoptosis Detection Kit (Intergen, NY, U.S.A.), as previously described (13). This method detects the DNA fragmentation associated with apoptosis by labeling 3'-OH DNA termini with digoxigenin-nucleotides, a process facilitated by terminal deoxynucleotidyl transferase (TdT). The labeled fragments produced are then allowed to bind to anti-digoxigenin antibody conjugated with peroxidase; AEC was used as a peroxidase substrate. Specimens were counter-stained with hematoxylin. Two independent investigators unaware of the experimental conditions counted apoptotic cells in 15 microscopic fields in the renal cortex, outer medulla or inner medulla under a light microscope at ×100; the determined inter-investigator variation was <5%. Numbers of apoptotic cells are expressed as cell numbers in each field; value represent mean value determined for 15 fields from each of the six experimental conditions and the control.
Data are presented as means±SD. Degrees of apoptosis were compared 48 hr after contrast injection (96 hr after ARF) using one-way ANOVA which was used SPSS ver. 10.0 for Windows. Change in creatinine levels at 48 hr and 96 hr after ARF were analyzed statistically using the Wilcoxon signed-rank test.
Creatinine ratios versus the control at 48 and 96 hr post-ARF in the CT subgroups were 2.45 and 3.16 in the low dose subgroup; 2.03 and 2.01 in the standard dose subgroup; and 1.76 and 1.79 in high dose subgroup, respectively. Similarly, creatinine ratios in the MR subgroups were 1.52 and 1.34 (low dose); 2.13 and 1.58 (standard dose); and 1.81 and 1.39 (high dose) (Table 1). No significant difference was observed between the creatinine levels of the CT and MR groups (p=0.116) 48 and 96 hr post-ARF. In addition, contrast dose levels were found to have similar effects on creatinine levels in the CT and MR groups (Table 2) (Fig. 1).
The CT group showed a slight increase in the number of scattered apoptotic cells in the cortex and medulla. The control group showed 18±0.8 apoptotic cells/×100 filed in the cortex and 12±2.8 in the medulla (Table 2). The low dose CT subgroup showed significantly more apoptosis in the renal cortex and medulla than the control group (p<0.05), whereas the high dose CT subgroup showed severe apoptosis in the cortex and medulla versus the low and standard CT subgroups (Fig. 2). The MR group showed slightly more scattered apoptotic cells in the cortex and medulla this increase was closely related to contrast dose (Fig. 3). Moreover, the CT group showed significantly more severe apoptosis in the cortex and medulla than the MR group (n=18; p<0.05) (Fig. 4). The numbers of apoptotic cells observed under the various condition used are presented in Fig. 4.
The aim of this study was to compare renal damage, as determined by serum creatinine and degree of apoptosis, caused by iodinated contrast or gadolinium in an acute renal failure animal model. This study shows that CT contrast medium dose not aggravate renal function in ARF rats than MR contrast medium. However, the observation of apoptosis in the renal cortex and medulla, demonstrates that CT contrast medium administration is associated with higher levels of cellular damage than MR contrast medium. Thus, the present study indicates that CT contrast medium should only be used for renal imaging studies when patients are well hydrated and after they have been administered preventive medications.
ARF has been reported after contrast medium injection and numerous theories have been proposed concerning the mechanism involved, which vary from vascular changes and tubular obstruction to direct contrast agent toxicity (1, 7-10, 14-16).
Several ARF animal model designs have been described; bilateral renal artery clamping for 45 min and subsequent release, which is similar to the model used in the present study (11, 12); the 2-mg/kg intravenous injection of HgCl2 (1 mg/dL) (7, 8); and the intramuscular injection of a 50% glycerol solution (10 mL/kg) (13). ARF model with drug injection do not provide a good animal model because they did not evaluate the renal function with the contrast medium reaction.
The incidence of acute contrast-induced reductions in renal function varies from 0 to 90% depending on the presence of various risk factors, which include chronic renal insufficiency, diabetes mellitus, and the amount of contrast agent administered (14-17). If a patient is suspicious for ARF or is in a high-risk group for renal failure, unenhanced CT is normally used to examine the genitourinary system, because an acute deterioration of renal function may occur after exposure to radiocontrast media. However, although a renal stone or cyst can be detected by CT without radiocontrast enhancement, renal contours and vascular-enriched masses cannot. The greatest impact of radiocontrast nephropathy is that in severe cases renal replacement therapy may be required, and to avoid this risk radiologic evaluation without radiocontrast, despite its limitations, is the current practice (7-9). On the other hand, clinicians have been able to perform MR studies in such patients since the modality was first introduced (18). Although gadolinium DTPA is ionic and has high-osmolarity, the required dose is small compared to the quantities of iodinated contrast medium required for CT (8). Currently, clinicians assumed that MR contrast media do not have adverse effects in poor renal function patients and that they can be freely used to evaluate renal function. Moreover, experience indicates that the technique is both safe and effective in cases of possible ARF and in patients at high-risk of ARF development.
Earlier studies encouraged hydration in patients with chronic renal insufficiency in order to prevent acute contrast-induced reductions in renal function (17, 19). It has been reported that vacuoles are formed in the proximal tubular cells in animals and humans after contrast injection (17, 19). Others have reported that nonionic and hypo-osmolar contrast media induced more vacuolization than the ionic, hyper-osmolar contrast medium diatrizoate (17, 19). Moreover, MR contrast media were found not to produce lysosomal alterations, and gadolinium DTPA administration in an ARF model showed no significant difference from controls (19). In the present study, apoptosis was more severe in the CT contrast group than in the MR contrast group (p<0.05). However, creatinine levels were not statistically different between these two groups, and did not respond to dose.
A reduction in the glomerular filtration rate (GFR) is a primary characteristic of ischemic ARF in humans and animals (20, 21), and serum creatinine levels are a good indicator of renal insufficiency (1). Therapeutic interventions that inhibit or promote renal tubular cell apoptosis potentially minimize renal dysfunction and accelerate recovery after ARF (5, 6). However, ARF and acute tubular necrosis continue to be clinically frustrating entities from both the diagnostic and therapeutic standpoints (22). Moreover, many preventive medications with contrast have been administered in animal and clinical studies. These include atrial natriuretic peptide (23), endothelin receptor antagonists (24), adenosine receptor antagonists (25), calcium antagonists (26), furosemide, mannitol, and dopamine-1 receptor agonist as well as inhibitors of oxygen/hydroxyl radical scavengers (27-29). In the present study, no preventive medication was administered prior to contrast medium.
Creatinine level is a good indicator of renal function (1), and apoptosis degree is a good indicator of renal damage (2-6). Lieberthal et al. (30) reported that high levels of creatinine were observed during the first phase of apoptosis in ARF (12-48 hr), and these were found to be well correlated with renal function and the degree of renal damage. During the second phase (the recovery phase; 5-7 days) creatinine levels were lower, which possibly contributed to injured tubule remodeling and facilitated their return to normal structural and functional states. However, the degree of renal apoptosis increased when contrast medium administration, as the contrast medium was probably toxic.
The present study has some limitations; 1) the number of animals used was small, 2) the rat ARF model may not well reflect human ARF, 3) creatinine levels were not constant after ARF and may depend on individual animal condition, 4) GFR was not calculated because urine creatinine levels were not measured, 5) the discrepancy between the creatinine levels and the degree of apoptosis observed in the two groups cannot be explained by proposed renal damage mechanism.
We conclude that CT contrast medium did not aggravate renal function in ARF rats more than MR contrast medium. However, based on the apoptosis observed in the renal cortex and medulla, it is apparent that CT contrast medium administration is associated with higher levels of apoptosis than MR contrast medium. Thus, we conclude that CT contrast medium should only be used for renal imaging studies when patients are well hydrated and after they have been administered preventive medications.
Figures and Tables
Fig. 1
Graph showing no significant difference in the creatinine levels of the CT and MR groups (p=0.116). In addition, no significant differences were found on changing CT or MR contrast medium dosages. CM, Contrast medium; ARF, Acute renal failure.

Fig. 2
Effect of CT contrast medium on the renal cortex and medulla. The red cells are TUNEL positive. Apoptotic degree in the renal medulla was more severe than that in the renal cortex. CT contrast medium increased the severity of apoptosis in a high dose. CT contrast medium induced more apoptosis in the renal cortex and medulla than MR contrast medium. (A) Cortex-control, (B) Cortex-low dose, (C) Cortex-standard dose, (D) Cortex-high dose, (E) Medulla-control, (F) Medulla-low dose, (G) Medulla-standard dose, (H) Medulla-high dose. Original magnification ×100 (Bar; 50 µm). C, Control; L, Low dose; S, Standard dose; H, High dose.
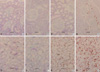
Fig. 3
Effect of MR contrast medium on the renal cortex and medulla. The red cells shown are TUNEL-positive. The degree of apoptosis observed in the renal medulla was more severe than in the renal cortex. MR contrast medium increased the severity of apoptosis in a dose-dependent manner. (A) Cortex-control, (B) Cortex-low dose, (C) Cortex-standard dose, (D) Cortex-high dose, (E) Medulla-control, (F) Medullalow dose, (G) Medulla-standard dose, (H) Medulla-high dose. Original magnification ×100 (Bar, 50 µm). C, Control; L, Low dose; S, Standard dose; H, High dose.

ACKNOWLEDGEMENTS
The author thank Bonnie Hami, MA, Department of Radiology, University Hospitals of Cleveland, for her editorial assistance in the preparation of this manuscript and Dr. John Roberts, Harrisco Language Research Institute, for his editorial assistance in the revision of manuscript.
References
1. Porter GA. Radiocontrast-induced nephropathy. Nephrol Dial Transplant. 1994. 9:146–156.
2. Schumer M, Colombel MC, Sawczuk IS, Gobe G, Connor J, O'Toole KM, Olsson CA, Wise GJ, Buttyan R. Morphologic, biochemical, and molecular evidence of apoptosis during the reperfusion phase after brief periods of renal ischemia. Am J Pathol. 1992. 140:831–838.
3. Kaushal GP, Basnakian AG, Shah SV. Apoptotic pathways in ischemic acute renal failure. Kidney Int. 2004. 66:500–506.


4. Dagher PC. Apoptosis in ischemic renal injury: roles of GTP depletion and p53. Kidney Int. 2004. 66:506–509.


5. Ueda N, Kaushal GP, Shah SV. Apoptotic mechanisms in acute renal failure. Am J Med. 2000. 108:403–415.


6. Park CP, Noh JW, Keum JS, Kim MS, Park MH. Apoptosis in renal hypertrophy after uninephrectomy in the rats. Korean J Pathol. 2001. 35:513–523.
7. Trillaud H, Degreze P, Combe C, Deminiere C, Palussiere J, Benderbous S, Grenier N. USPIO-enhanced MR imaging of glycerol-induced acute renal failure in the rabbit. Magn Reson Imaging. 1995. 13:233–240.


8. Carvlin MJ, Arger PH, Kundel HL, Axel L, Dougherty L, Kassab EA, Moore B. Acute tubular necrosis: use of gadolinium-DTPA and fast MR imaging to evaluate renal function in the rabbit. J Comput Assist Tomogr. 1987. 11:488–495.
9. Laissy JP, Benderbous S, Idée JM, Chillon S, Beaufils H, Schouman-Claeys E. MR assessment of iodinated contrast-medium-induced nephropathy in rats using ultrasmall particles of iron oxide. J Magn Reson Imaging. 1997. 7:164–170.


10. Prasad PV, Priatna A, Spokes K, Epstein FH. Changes in intrarenal oxygenation as evaluated by BOLD MRI in a rat kidney model for radiocontrast nephropathy. J Magn Reson Imaging. 2001. 13:744–747.


11. Park SK, Kang MJ, Kim W, Koh GY. Renal tubule regeneration after ischemic injury is coupled to the up-regulation and activation of cyclins and cyclin dependent kinases. Kidney Int. 1997. 52:706–714.


12. Heyman SN, Lieberthal W, Rogiers P, Bonventre JV. Animal models of acute tubular necrosis. Curr Opin Crit Care. 2002. 8:526–534.


13. Nath KA, Grande JP, Croatt AJ, Likely S, Hebbel RP, Enright H. Intracellular targets in heme protein-induced renal injury. Kidney Int. 1998. 53:100–111.


14. Katholi RE, Taylor GJ, Woods WT, Womack KA, Katholi CR, McCann WP, Moses HW, Dove JT, Mikell FL, Woodruff RC, Miller BD, Schneider JA. Nephrotoxicity of nonionic low-osmolality versus ionic high-osmolality contrast media: a prospective double-blind randomized comparison in human beings. Radiology. 1993. 186:183–187.


15. Tepel M, van der Giet M, Schwarzfeld C, Laufer U, Liermann D, Zidek W. Prevention of radiographic-contrast-agent-induced reductions in renal function by acetylcysteine. N Engl J Med. 2000. 343:180–184.


16. Heyman SN, Reichman J, Brezis M. Pathophysiology of radiocontrast nephropathy. A role for medullary hypoxia. Invest Radiol. 1999. 34:685–691.
17. Deray G, Dubois M, Martinez F, Baumelou B, Beaufils H, Bourbouze R, Baumelou A, Jacobs C. Renal effects of radiocontrast agents in rats: a new model of acute renal failure. Am J Nephrol. 1990. 10:507–513.


18. Brillet G, Dubois M, Beaufils H, Bourbouze R, Deray G. Renal tolerance of AMI 25. Invest Radiol. 1991. 26:879–881.


19. Tervahartiala P, Kivisaari L, Kivisaari R, Virtanen I, Standertskjöld-Nordenstam CG. Contrast media-induced renal tubular vacuolization. A light and electron microscopic study on rat kidneys. Invest Radiol. 1991. 26:882–887.
20. Kim SJ, Lim YT, Kim BS, Cho SI, Woo JS, Jung JS, Kim YK. Mechanism of reduced GFR in rabbits with ischemic acute renal failure. Ren Fail. 2000. 22:129–141.


21. Arendshorst WJ, Finn WF, Gottschalk CW. Pathogenesis of acute renal failure following temporary renal ischemia in the rat. Circ Res. 1975. 37:558–568.


22. Vari RC, Natarajan LA, Whitescarver SA, Jackson BA, Ott CE. Induction, prevention and mechanisms of contrast media-induced acute renal failure. Kidney Int. 1988. 33:699–707.


23. Kurnik BR, Weisberg LS, Cuttler IM, Kurnik PB. Effects of atrial natriuretic peptide versus mannitol on renal blood flow during radiocontrast infusion in chronic renal failure. J Lab Clin Med. 1990. 116:27–36.
24. Bird JE, Giancarli MR, Megill JR, Durham SK. Effects of endothelin in radiocontrast-induced nephropathy in rats are mediated through endothelin-a receptors. J Am Soc Nephrol. 1996. 7:1153–1157.


25. Erley CM, Heyne N, Burgert K, Langanke J, Risler T, Osswald H. Prevention of radiocontrast-induced nephropathy by adenosine antagonists in rats with chronic nitric oxide deficiency. J Am Soc Nephrol. 1997. 8:1125–1132.


26. Alvarez A, Martul E, Velga F, Forteza J. Functional, histologic, and ultrastructural study of the protective effects of verapamil in experimental ischemic acute renal failure in the rabbit. Ren Fail. 1994. 16:193–207.


27. Touati C, Idee J, Deray G, Santus R, Balut C, Beaufils H, Jounanneau C, Bourbouze R, Doucet D, Bonnemain B. Modulation of the renal effects of contrast media by endothelium-derived nitric oxide in the rat. Invest Radiol. 1993. 28:814–820.


28. Liss P. Effects of contrast media on renal microcirculation and oxygen tension. Acta Radiol Suppl. 1997. 409:1–29.
29. Bakris GL, Lass NA, Glock D. Renal hemodynamics in radiocontrast medium-induced renal dysfunction: a role for dopamine-1 receptors. Kidney Int. 1999. 56:206–210.


30. Lieberthal W, Koh J, Levine JS. Necrosis and apoptosis in acute renal failure. Semin Nephrol. 1998. 18:505–518.