Abstract
Interleukin-1β (IL-1β) is a pivotal proinflammatory cytokine. To investigate the mechanism of IL-1β-induced cell death in human malignant melanoma A375-S2 cells, MTT assay, photomicroscopical observation, DNA agarose gel electrophoresis, radioimmunoassay and Western blot analysis were carried out. IL-1β did not only induce nuclear condensation and DNA fragmentation, but also increased degradation of two substrates of caspase-3, poly ADP-ribose polymerase (PARP) and inhibitor of caspase-activated DNase (ICAD). Simultaneously, release of precursor of IL-1β (pro-IL-1β) and endogenous IL-1β production were involved in the apoptotic process. IL-1β enhanced the ratio of Bax/Bcl-2 and Bax/Bcl-xL expression and up-regulated apoptosis inducing factor (AIF) expression, which required the activation of downstream caspases. These results suggest that IL-1β induces endogenous IL-1β production, enhances cleavage of caspase downstream substrates and promotes mitochondria mediated apoptosis in A375-S2 cells.
Interleukin-1β (IL-1β) is one of the multifunctional cytokines produced predominantly by activated monocytes and macrophages (1). It exerts not only pleiotropic effects in host reactions such as immune defence, inflammation and homeostasis (2), but also regulates a variety of cell functions of many cell types in both positive and negative manners. For instance, in human thymocytes, B cells and fibroblasts, IL-1β acts as a growth stimulator for the cell proliferation (3-5), while growth of some other cells, such as human endothelial cells, breast carcinoma cells, mouse myeloid leukemia cells and human melanoma cells, is inhibited by IL-1β (6-9). Previous studies reported that IL-1β induced growth inhibition in human melanoma cells through down-regulation of ornithine decarboxylase (ODC) (10, 11), up-regulation of IL-6 (12) and cell cycle arrest (13). Research carried out by Itoh and his coworkers also indicated that this antiproliferative effect was mediated by p38 mitogen-activated protein kinase (p38 MAPK) (14). Since human melanoma A375-S2 cell exhibits the highest sensitivity to IL-1β in cell death and was used to determine the activity of IL-1β (15), we selected this cell line to conduct the present study.
In general, cells die through two distinct ways: apoptosis or necrosis. In this study, IL-1β-induced death of A375-S2 cells represented some characteristics of apoptosis by morphologic observation under phase contrast microscopy. To verify that IL-1β promotes cell death through apoptotic pathways in A375-S2 cells, caspases, Bcl-2 family proteins and apoptosis inclucing factor (AIF), which have been proved to play important roles in the process of cellular apoptosis, were detected by the caspase activity assay and Western blot analysis. Cysteine proteases of the caspase family play a role in the apoptotic signaling and the execution of apoptosis by cleaving critical cellular proteins solely after aspartate residues. The main function of initiator caspases such as caspase-8 and -9 is to activate downstream executor caspases such as caspase-3, which ultimate in apoptosis by cleavage of death substrates (16, 17). It was considered that caspase-1 is also an important upstream caspase (16), which not only mediate apoptosis, but also cleave the precursor of IL-1β into bioactive cytokine (18). Bcl-2 family proteins are vital for regulation of apoptosis by controlling the mitochondrial membrane potential to release cytochrome c and activating caspase-9, -3 (19, 20). The balance between proapoptotic proteins and antiapoptotic proteins determine the fate of cells (21, 22). In mitochondria-dependent cell death, translocation of AIF from mitochondria and its activation are induced by the activation of caspase-9 pathway (23). In addition, the production of endogenous cytokine has also aroused our interest.
In this study we demonstrated that activation of caspases, AIF and induction of endogenous IL-1β contributed to IL-1β-induced A375-S2 cell apoptosis, and up-regulation of Bax, and down-regulation of Bcl-2, Bcl-xL amplified the activation of caspase cascade in this process.
Human recombinant IL-1α, IL-1β, TNF-α and IL-6 proteins expressed by transfected Escherichia coli were purified by chromatography on DEAE-Sepharose Fast Flow (Amersham Pharmacia Biotech, Uppsala, Sweden) and concentrated by dialysis membrane. The purified recombinant proteins with theoretical molecular mass were analyzed by 15% polyacrylamide gel electrophoresis with sodium dodecyl sulfate (SDS-PAGE). 3-(4,5-dimethylthiazol-2-yl)-2,5-diphenyltetra zolium (MTT), RNase and proteinase K were obtained from Sigma Chemical (St. Louis, MO, U.S.A.). Rabbit polyclonal antiserum against pro-IL-1β was prepared in our laboratory. Rabbit polyclonal antibodies against PARP, ICAD, Bax, AIF and mouse polyclonal antibody against Bcl-2 were purchased from Santa Cruz Biotechnology (Santa Cruz, CA, U.S.A.). Mouse polyclonal antibody against Bcl-xL was from Oncogene Research Products (Boston, MA, U.S.A.).
Human malignant melanoma cell, A375-S2, was obtained from the American Type Culture Collection (#CRL-1872, Manassas, VA, U.S.A.). The cells were cultured in RPMI 1640 (Gibco, Grand Island, NY, U.S.A.) medium supplemented with 10% fetal bovine serum (FBS, Dalian Biological Reagent Factory, Dalian, China), 2 mM L-glutamine (Gibco), 100 U/mL penicillin, and 100 µg/mL streptomycin, and maintained at 37℃ with 5% CO2 in a humidified atmosphere. The cells at exponential phase of growth were used in these experiments.
Apoptotic nuclear morphology was assessed using Hoechst 33258 (Sigma) as described previously (24). Cells were fixed with 3.7% paraformaldelyde for 30 min at room temperature, then washed and stained with Hoechst 33258 167 µM at 37℃ for 30 min. The cells were then washed and resuspended in PBS for morphological observation using a fluorescence microscope (Olympus, Tokyo, Japan).
A375-S2 cells were incubated with 1 nM IL-1β for 0, 24, 48 and 72 hr. Both adherent and non-adherent cells (1×106 cells) were collected by centrifugation at 1,000×g for 5 min. The cell pellets was suspended in cell lysis buffer [Tris-HCl 10 mM (pH 7.4), edetic acid 10 mM (pH 8.0), Triton-X100 (0.5%)] and kept at 4℃ for 10 min. The lysate was centrifuged at 25,000×g for 20 min. The supernatant was incubated with RNase 40 µg/L at 37℃ for 1 hr, and then incubated with proteinase K 40 µg/L at 37℃ for 1 hr. The supernatant was mixed with NaCl 0.5 M and 50 % 2-propanol at -20℃ overnight, and then centrifuged at 25,000×g for 15 min. After drying, DNA was dissolved in TE buffer [Tris-HCl 10 mM (pH 7.4), edetic acid 1 mM (pH 8.0)] and separated by 2% agarose gel electrophoresis at 100 V for 1 hr.
A375-S2 cells were treated with 1 nM IL-1β for 0, 12, 24, 48 and 72 hr. After washing in phosphate buffered saline, cells were collected and lysed in 50 µL of the cell lysis buffer included in caspase-1, -3 and -8 apoptosis detection kit (Santa Cruz Biotechnology). The enzymatic activities of caspase-1, -3 and -8 activity were measured with caspase-1 substrate (YVAD-AFC), capase-3 substrate (DEVD-AFC) and caspase-8 substrate (IETD-AFC), respectively, according to the manufacturer's instruction. The activity of capase-9 was measured by caspase-9 colorimetric activity assay kit (Santa Cruz Biotechnology).
After cell lysis and centrifugation at 12,000×g in 1.5 mL Eppendorf tube, secreted IL-1β and pro-IL-1β were quantitated by human cytokine specific radioimmunoassay (RIA). Methods for RIA for human cytokines were followed as previously reported (25, 26). Exogenous IL-1β was substracted from quantity of the measured IL-1β (pro-IL-1β plus IL-1β) amount.
A375-S2 cells were treated with 1 nM IL-1β for 0, 12, 24, 48 and 72 hr. Both adherent and floating cells were collected. Then Western blot analysis was carried out with some modification. Briefly, the cell pellets were resuspended in lysis buffer, including HEPES (50 mM pH 7.4), Triton-X100 1%, sodium orthovanadate (2 mM), sodium fluoride (100 mM), edetic acid (1 mM), PMSF 1 mM (Sigma), aprotinin 10 µg/mL (Sigma), leupeptin 10 µg/mL (Sigma), and lysed on ice for 60 min. After centrifugation of the cell suspension at 13,000×g for 15 min, the protein content of supernatant was determined by Bio-Rad protein assay reagent (Bio-Rad, Hercules, CA, U.S.A.). Equivalent amounts of protein lysates were mixed in 2×loading buffer [50 mM Tris-HCl (pH 6.8), 2% SDS, 10% 2-mercaptoethanol, 10% glycerol, and 0.002% bromophenol blue], heated at 100℃ for 5 min, then analyzed by electrophoresis in 12% SDS polyacrylamide gel electrophoresis and blotted onto nitrocellulose membrane (Amersham Biosciences, Piscataway, NJ, U.S.A.). Pro-IL-1β was detected using polyclonal antiserum pro-IL-1β PARP, ICAD, Bcl-2, Bcl-xL, Bax and AIF were detected using polyclonal antibodies against them, followed by addition of secondary antibody conjugated with horseradish peroxidase (HRP) and 3,3-diaminobenzidine tetrahydrochloride (DAB) as HRP substrate.
To examine the sensitivity of A375-S2 cells to human proinflammatory cytokines, we measured the anti-proliferative effects of four cytokines, IL-1α, IL-1β, TNF-α and IL-6, which have cytocidal effects on A375-S2 cells. After incubation with 10-14M to 10-6 M cytokines for 72 hr, IL-1β showed the strongest cytocidal effect among these cytokines at the same concentration (Fig. 1).
To determine the features of A375-S2 cell death induced by IL-1β, apoptosis assessment assay including morphological analysis and DNA fragmentation analysis were performed. The nuclear morphologic changes were analyzed by Hoechst 33258 staining. In the control group, nuclei of A375-S2 cells were round in shape and stained homogeneously (Fig. 2A). After treatment with 1 nM IL-1β for 72 hr, apoptotic cells had condensed and fragmented nuclei (Fig. 2B). Treatment with 1 nM IL-1β for 72 hr resulted in typical DNA fragmentation in agarose electrophoresis, which is a hallmark of apoptosis (Fig. 2C). These results suggested that IL-1β induced A375-S2 cell death through apoptotic pathways.
To investigate the signal transduction pathways underlying IL-1β-induced apoptosis in A375-S2 cells, assessment of caspase-1, -3, -8 and -9 activities was performed. After treatment with 1 nM IL-1β for 24 hr, activity of caspase-1 and upstream caspase-8 reached a peak, 1.5 times (p<0.01) and 3 times (p<0.01) higher than those of control group, respectively. Simultaneously, activity of caspase-9 was also increased to 3.7 times (p<0.01) of control. Then downstream caspase-3 activation was augmented to nearly four-fold increase (p<0.01) compared to the control group at 48 hr (Fig. 3).
The two classical caspase substrate, PARP (poly ADP-ribose polymerase) (27) and ICAD (inhibitor of caspase-activated DNase) (28) were applied to investigate the role of downstream capases. As shown in Fig. 4, after incubation with 1 nM IL-1β for 12 hr, PARP protein was cleaved to 85 kDa fragment and ICAD protein expression also decreased during the apoptotic process. These results indicated that caspase-3's activation and cleavage of its substrates, PARP and ICAD, were involved in IL-1β-induced apoptosis.
The precursor of IL-1β (pro-IL-1β) has been identified as substrate for caspase-1 (IL-1β converting enzyme, ICE), and pro-IL-1β (33 kDa) is inactive until its cleavage by caspase-1 into bioactive IL-1β (17.5 kDa) (29). To investigate whether treatment of exogenous IL-1β can increase the expression of endogenous IL-1β, production of IL-1β was quantitated by radioimmunoassay. In Western blot analysis, pro-IL-1β protein expression was not observed without administration of exogenous IL-1β, and then was increased by treatment with 1 nM IL-1β especially at 24 hr (Fig. 5A). As shown in Fig. 5B, after treatment with various doses of IL-1β for 24 hr, production of IL-1β and pro-IL-1β were increased in a dose dependent manner, although there was minor differences in several cell densities. These results indicated that exogenous IL-1β induced-endogenous pro-IL-1β production and increased caspase-1 activity had strong relationship to pro-IL-1β's cleavage to IL-1β and its secretion.
In mitochondria, Bcl-2 family proteins play an important role in the regulation of apoptosis. Some of them including Bcl-2 and Bcl-xL suppress apoptosis, while others such as Bax and Bid promote apoptosis (21, 30). Furthermore, AIF is required for mitochondrial pathway dependent apoptosis (31). In view of the roles of Bcl-2 family proteins and AIF in the apoptotic pathway, Western blot analysis was carried out to examine the expression ratio of Bcl-2, Bcl-xL, Bax and AIF during the apoptosis. As shown in Fig. 6A, though Bax expression level slightly increased, expression of Bcl-2 and Bcl-xL were significantly reduced after exposure to 1 nM IL-1β. The ratio of Bax/Bcl-2 and Bax/Bcl-xL expression increased in a time dependent manner. As expected, AIF expression level increased during IL-1β-induced apoptosis in A375-S2 cells (Fig. 6B). In view of these data, mitochondrial pathway was supposed to participate in IL-1β-induced A375-S2 cell apoptosis.
Antiproliferative effect of IL-1β in human melanoma cell A375 has been reported to be involved in some signaling pathways (10-14), which had not been proved to be related with apoptosis. In this study, we have obtained evidences supporting that IL-1β could induce A375-S2 cell apoptosis through caspase and mitochondrial pathways. Based on the morphologic changes, DNA fragmentation, we concluded that IL-1β induced A375-S2 cell death involved in a machinery of apoptosis. Up-regulation of caspase-1, upstream caspase-8, -9 and downstream caspase-3 activities confirmed the participation of caspases in A375-S2 cell apoptosis. Moreover, caspase-8 might locate at downstream of IL-1β receptor and play their roles in the apoptosis.
PARP and ICAD are downstream caspase-3 substrates that control nuclear apoptosis. PARP is a 116 kDa nuclear enzyme which detects and binds to DNA strand breaks and function in transcription and DNA repair (27). CAD activation is attributed to the cleavage of ICAD, which is cleaved by caspase-3 or caspase-7 (32), resulting in DNA fragmentation. In our study, caspase activity assay and Western blot analysis showed that downstream caspase-3 was evidently activated during A375-S2 cell apoptosis, and the endogenously existing PARP and ICAD in A375-S2 cells were down-regulated, leading to typical apoptotic chromatin condensation as shown in Hoechst 33258 staining, and the consequent appearance of DNA ladder in electrophoresis. Therefore, IL-1β triggered the activation of caspase-3 which was responsible for degradation of PARP and ICAD.
Caspase-1, which is also known as IL-1β-converting enzyme (ICE), is a specific intracellular cysteine protease that cleaves the precursor of IL-1β (pro-IL-1β) into bioactive cytokine (29). Our study demonstrated that both pro-IL-1β expression and endogenous IL-1β production in A375-S2 cells increased after treatment with IL-1β. This augmentation exhibited minor differences in various cell densities, that is, when cell numbers increased, production of cytokine might not necessarily increase. It was speculated that maybe when the cell numbers increased excessively, some signaling transduction pathways of cytokine production would be inhibited or altered by cell excessive coalescence, the detailed machinery in this process remains to be confirmed. After treatment with IL-1β for 24 hr, pro-IL-1β expression reached a peak, and simultaneously, caspase-1 was activated as described above, indicating that induced pro-IL-1β was cleaved by caspase-1 (ICE) into IL-1β. Thus, IL-1β-induced apoptosis in A375-S2 cells is not only a direct effect of caspase-1 as a member of caspase cascade, but also is mediated indirectly through pro-IL-1β synthesis and its cleavage by caspase-1.
A375-S2 cells express both the proapoptotic Bax and the antiapoptotic Bcl-2 and Bcl-xL. Bcl-2 and Bcl-xL proteins are known to act as antiapoptotic components that prevent Bax from exerting its proapoptotic effect (21, 22). In our study, A375-S2 cell apoptosis was associated with both decreased Bcl-2 and Bcl-xL expression and slightly increased Bax expression, resulting in a sustained increase in the Bax/Bcl-2 and Bax/Bcl-xL ratios and release of cytochrome c, which might play a critical role in regulation of IL-1β-induced apoptosis. Postmitochondrial caspase-9 activation is dependent on the release of cytochrome c and formation of cytochrome c/Apaf-1 (apoptotic protease-activating factor-1)/caspase-9 'apoptosome', and then downstream caspase-3 and AIF are activated (23). Therefore, AIF was thought to be essential for the mitochondrial pathway dependent apoptosis. In the present study, AIF activation was supposed to contribute to apoptosis in response to IL-1β treatment.
In conclusion, IL-1β induced A375-S2 cell apoptosis through decreased expression of PARP and ICAD in response to the activation of caspase cascade at earlier stage and induction of endogenous IL-1β. Simultaneously, IL-1β-induced apoptosis was mediated by mitochondria, through up-regulation of Bax and down-regulation of Bcl-2, Bcl-xL to initiate caspase-9 activity, leading to the activation of caspase-3 and release of AIF in this apoptotic process.
Figures and Tables
Fig. 1
Inhibitory effects of four cytokines on A375-S2 cells. A375-S2 cells were incubated with IL-1α, IL-1β, TNF and IL-6 at various doses for 72 hr. The data are presented as mean shown only a part here of the results for three independent experiments.
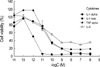
Fig. 2
IL-1β-induced apoptosis in A375-S2 cells. Morphologic changes of A375-S2 cell nuclei were observed by fluorescence microscopy. (A) medium control. (B) After treatment with 1 nM IL-1β for 72 hr, the cells were stained with Hoechst 33258 using ×400 manification. Arrows indicate condensed and fragmented nuclei. (C) Internucleosomal DNA fragmentation induced in A375-S2 cells by IL-1β. The cells were treated with 1 nM IL-1β for 0, 24, 48 and 72 hr (lane 2, 3, 4 and 5; M: molecular weight marker).
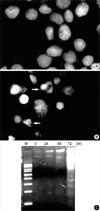
Fig. 3
Effects of IL-1β on the activation of caspase-1, -3, -8 and -9 in A375-S2 cells. The cells were treated with 1 nM IL-1β for 0, 12, 24, 48 and 72 hr. The data are presented as mean±S.D. of the results for three independent experiments.
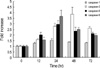
Fig. 4
Expression of PARP and ICAD in IL-1β-treated A375-S2 cells. The cells were treated with 1 nM IL-1β for 0, 12, 24, 48 and 72 hr. Cell lysates were separated by 12% SDS-PAGE electrophoresis, and PARP and ICAD proteins were detected by Western blot analysis.
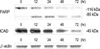
Fig. 5
IL-1β-induced production of IL-1β by A375-S2 cells. (A) The cells were treated with 1 nM IL-1β for 0, 12, 24, 48 and 72 hr. The cell lysates were separated by 12% SDS-PAGE electrophoresis, and pro-IL-1β protein bands was detected by Western blot analysis. (B) A375-S2 cells (1.25, 0.63, 0.32, 0.16, 0.08×106 cells/mL) were treated with 0.005, 0.05, 0.5, 5 nM IL-1β for 24 hr.
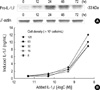
References
1. Pascale RL. The Interleukin-1 family. Eur Cytokine Netw. 1998. 9:565–576.
2. Dinarello CA. Biologiical basis for interleukin-1 in disease. Blood. 1996. 87:2095–2147.
3. Burger D, Molnarfi N, Gruaz L, Dayer JM. Differential induction of IL-1beta and TNF by CD40 ligand or cellular contact with stimulated T cells depends on the maturation stage of human monocytes. J Immunol. 2004. 173:1292–1297.
4. Freedman AS, Freeman G, Whitman J, Segil J, Daley J, Nadler LM. Pre-exposure of human B cells to recombinant IL-1 enhances subsequent proliferation. J Immunol. 1988. 141:3398–3404.
5. Vesey DA, Cheung C, Cuttle L, Endre Z, Gobe G, Johnson DW. Interleukin-1beta stimulates human renal fibroblast proliferation and matrix protein production by means of a transforming growth factor-beta-dependent mechanism. J Lab Clin Med. 2002. 140:342–350.
6. Maier JA, Voulalas P, Roeder D, Maciag T. Extension of the life-span of human endothelial cells by an interleukin-1α antisense oligomer. Science. 1990. 249:1570–1574.
7. Komeva EA, Shanin SN, Rybakina EG. The role of interleukin-1 in stress-induced changes in immune system function. Neurosci Behav Physiol. 2001. 31:431–437.
8. Onozaki K, Tamatani T, Hashimoto T, Matsushima K. Growth inhibition and augmentation of mouse myeloid leukemic cell line differentiation by Interleukin-1. Cancer Res. 1987. 47:2397–2402.
9. Lachman LB, Dinarello CA, Llansa ND, Fidler IJ. Natural and recombinant human interleukin 1-beta is cytotoxic for human melanoma cells. J Immunol. 1986. 136:3098–3102.
10. Endo Y, Matsushima K, Onozaki K, Oppenheim JJ. Role of ornithine decarboxylase in the regulation of cell growth by IL-1 and tumor necrosis factor. J Immunol. 1988. 141:2342–2348.
11. Yang D, Hayashi H, Takii T, Mizutani Y, Inukai Y, Onozaki K. Interleukin-1-induced growth inhibition of human melanoma cells. J Biol Chem. 1997. 272:3376–3383.


12. Morinaga Y, Suzuki H, Takatsuki F, Akiyama Y, Taniyama T, Matsushima K, Onozaki K. Contribution of IL-6 to the antiproliferative effect of IL-1 and tumor necrosis factor on tumor cell lines. J Immunol. 1989. 143:3538–3542.
13. Murai T, Yukari N, Hideko M, Terada K. Altered regulation of cell cycle machinery involved in interleukin-1-induced G1 and G2 phase growth arrest of A375S2 human melanoma cells. J Biol Chem. 2001. 276:6797–6806.


14. Itoh S, Hattori T, Hayashi H, Mizutani Y, Toto M, Takii T, Yang D, Lee JC, Matsufuji S, Murakami Y, Chiba T, Onozaki K. Antiproliferative effect of IL-1 is mediated by p38 mitogen-activated protein kinase in human melanoma cell A375. J Immunol. 1999. 162:7434–7440.
15. Tuo W, Harney JP, Bazer FW. Developmentally regulated expression of interleukin-1 beta by peri-implantation conceptuses in swine. J Reprod Immunol. 1996. 31:185–198.
18. Thornberry NA, Bull HG, Calaycay JR, Chapman KT, Howard AD, Kostura MJ, Miller DK, Molineaux SM, Weidner JR, Aunins J. A novel heterodimeric cysteine protease is required for interleukin-1β processing in monocytes. Nature. 1992. 30:768–774.
19. Akgul C, Moulding DA, Edwards SW. Molecular control of neutrophil apoptosis. FEBS Lett. 2001. 487:318–322.


20. Han Z, Pantazis P, Wyche JH, Kouttob N, Kidd VJ, Hendrickson EA. A Fas-associated death domain protein-dependent mechanism mediates the apoptotic action of non-steroidal anti-inflammatory drugs in the human leukemic Jurkat cell line. J Biol Chem. 2001. 276:38748–38754.


21. Kroemer G. The proto-oncogene Bcl-2 and its role in regulating apoptosis. Nat Med. 1997. 3:614–620.


22. Weinmann P, Gaehtgens P, Walzog B. Bcl-xL-and Bax-α-mediated regulation of apoptosis of human neutrophils via caspase-3. Blood. 1999. 93:3106–3115.
23. Arnoult D, Gaume B, Karbowski M, Sharpe JC, Cecconi F, Youle RJ. Mitochondrial release of AIF and EndoG requires caspase activation downstream of Bax/Bak-mediated permeabilization. EMBO J. 2003. 22:4385–4399.


24. Sarin A, Haddad EK, Henkart PA. Caspase dependence of target cell damage induced by cytotoxic T lymphocytes. J Immunol. 1998. 161:2810–2816.
25. van der Meer JW, Endres S, Lonnemann G, Cannon JG, Ikejima T, Okusawa S, Gelfand JA, Dinarello CA. Concentration of immunoreactive human tumor necrosis factor alpha produced by human mononuclear cells in vitro. J Leukocyte Biol. 1998. 43:216–223.
26. Endres S, Ghorbani R, Lonnemann G, van der Meer JW, Dinarello CA. Measurement of immunoreactive interleukin-1β from human mononuclear cell: optimization of recovery, intransubject consistency, and comparison with interleukin-1α and tumor necrosis factor. Clin Immunol Immunopathol. 1988. 49:424–438.
27. Decker P, Isenberg D, Muller S. Inhibition of caspase-3-mediated poly (ADP-ribose) polymerase (PARP) apoptotic cleavage by human PARP autoantibodies and effect on cells undergoing apoptosis. J Biol Chem. 2000. 275:9043–9046.
28. Chen DX, Stetler RA, Cao GD. Characterization of the rat DNA fragmentation factor 35/inhibitor of caspase-activated DNase (short form). J Biol Chem. 2000. 275:38508–38517.


29. Siegmund B, Lehr HA, Fantuzzi G, Dinarello CA. IL-1β-converting enzyme (caspase-1) in intestinal inflammation. Proc Natl Acad Sci USA. 2001. 98:13249–13254.


30. Kim YM, Talanian RV, Billiar TR. Nitric oxide inhibits apoptosis by preventing increases on caspase-3-like activity via two distinct mechanisms. J Biol Chem. 1997. 272:31138–31148.