Abstract
1α,25-dihydroxyvitamin D3 (1,25(OH)2D3), which is the biologically active form of vitamin D, has anti-inflammatory effects and can prevent experimental Parkinson's disease (PD). 1,25(OH)2D3 exerts most of its actions only after it binds to its specific nuclear receptors. Eighty-five Korean patients with PD and 231 unrelated healthy individuals were evaluated to determine if vitamin D receptor gene (VDRG) BsmI polymorphisms were markers for the susceptibility to PD in Korean patients. Each polymorphism was detected using polymerase chain reaction (PCR)-based restriction analysis. In addition, the relationship between the BsmI polymorphisms and the clinical manifestations of PD was evaluated. Overexpression of the b allele (91.2 vs. 85.7%; p=0.069) and homozygote bb (84.7 vs. 72.7%; p=0.043) was found in the PD patients compared with the controls. These results show for the first time an association between PD and a VDRG polymorphism, which might be involved in the pathogenesis of PD, or in the linkage disequilibrium of the VDRG to another pathogenic gene locus.
The glial cell line-derived neurotrophic factor (GDNF) has been suggested to be a candidate treatment for Parkinson's disease (PD) (1, 2). Recent in vivo data suggests that 1α,25-dihydroxyvitamin D3 (1,25(OH)2D3) treatment might enhance GDNF mRNA and protein expression in the striatum of adult rats (3). 1,25(OH)2D3 has also been shown to attenuate the hypokinesia and neurotoxicity induced by 6-hydroxydopamine in rats (4).
The genomic mechanism of vitamin D is mediated through the vitamin D receptor (VDR) (5). Since the presence of the vitamin D specific nuclear binding in the brain and spinal cord was reported, there has been accumulated evidence suggesting that both the mRNA encoding the VDR and the protein itself are present in the nervous system (6-8). The human VDR gene contains eight coding exons and three alternative 5'non-coding exons spanning more than 75 kb of the DNA on chromosome 12 (9). The VDR belongs to the nuclear hormone receptor superfamily, and modulates the transcription of the target genes in response to 1,25(OH)2D3 (10).
Although several animal studies indicate the potential use of 1,25(OH)2D3 in neurodegenerative diseases, the role of VDR in neurodegeneration has not been clarified and the association between VDR polymorphisms and these diseases has received little attention.
The effect of the VDR genotypes on PD along with their possible importance in predisposing certain individuals to PD prompted us to evaluate the association between the VDR genotype and PD. This study examined the polymorphisms of the VDR gene intron 8 (BsmI) in order to understand the role of the VDR gene in the susceptibility to PD in Koreans. The possible interactions of the VDR gene in terms of gender, the age at onset, clinical expression, and dopa-responsiveness were also investigated.
Eighty-five PD patients (30 men and 55 women, mean age: 64.55±8.86 yr) were evaluated by a neurologist and diagnosed with idiopathic PD based on the presence of two or more of the cardinal features of PD (tremor, rigidity, and bradykinesia), as well as by the lack of signs not related to PD. The secondary forms of parkinsonism were excluded. However, no neuropathological investigations were carried out to define the Lewy body pathology because the patients are still alive. All the PD patients included in this study were sporadic based on pedigree analysis. The local ethics committee approved this study, and all the subjects provided informed consent.
The age at onset was categorized into early-onset (<50 yr) or late-onset (>70 yr), respectively (11). In order to determine the levodopa-responsiveness, the levodopa test was performed in the morning during a fasting state. All the patients were first rated using the unified Parkinson's disease rating scale (UPDRS) motor subscale, then challenged with a single dose of 250/25 levodopa/carbidopa and re-rated 1 hr later. The dopa-responsiveness was defined as a minimum 30% improvement in the scores (12). The UPDRS was also used to assess the severity of the motor symptoms and signs. An average global tremor score as well as the mean score for the complex of postural instability and gait difficulty (PIGD) was calculated as previously described (13). The tremor-predominant group was defined as those patients with a mean tremor score/mean PIGD score ≥1.5. The PIGD group included all patients with a ratio ≤1.0.
The control group consisted of 231 unrelated individuals, who were mainly recruited from the health examination clinic of the Kangnam St. Mary Hospital. The control subjects were screened to ensure that there was no history of neurological and psychiatric disorders. The control individuals had an age and gender distribution similar to the patients.
The genomic DNA was extracted from the peripheral blood using a Genomic DNA isolation reagent kit (QIAamp® kit, Pel-Freez® Clinical Systems). The polymerase chain reaction (PCR) for the intron 8 polymorphisms was performed using a PCR thermocycler (ThermoHybaid PCR Sprint, Thermo Electron Corporation, U.S.A.) and BsmI restriction enzyme (Roche). The BsmI VDR polymorphism was tested as described previously (9). The primers flanking the BsmI restriction site are as follows: forward primer, 5'-CAA CCA AGA CTA CAA GTA CCG CGT CAG TGA-3' and backward primer, 5'-AAC CAG CGG GAA GAG GTC AAG GG-3'. The BsmI restriction polymorphism revealed two possible alleles, allele B (absence of a restriction site) and b (presence of a restriction site). The genotypes were defined as BB (absence of restriction site on both alleles, one band at 825 bp), bb (presence of a restriction site on both alleles, two bands at 650 and 175 bp), or Bb (heterozygous, three bands at 825, 650, and 175 bp).
The allelic and genotypic distributions of the patients and controls were compared. In addition, the following comparisons were performed: the allelic distribution between the early-onset group and the late-onset group, the tremor group and PIGD group, the dopa-responsive group and dopa-non-responsive group. The results were compared using a chi-square test for statistical significance. Calculations were performed using SPSS for Windows version 11.0 (SPSS Inc., Chicago, IL, U.S.A.). A p value <0.05 was considered significant.
The gender distribution and the mean age of the PD patients and control subjects were similar (Table 1). Table 2 shows that among the PD patients, two patients (2.4%) had the VDR genotype BB, 11 patients (12.9%) had Bb, and 72 (84.7%) had bb. Among the 231 control subjects, the VDR genotype BB was identified in 3 (1.3%), Bb in 60 (26%), and bb in 168 subjects (72.7%). The VDR genotype bb was significantly more common in the PD patients than in the control subjects. The allelic frequency in the PD patients was 15 (8.8%) with the homozygote B and 155 (91.2%) with the homozygote b, whereas the distribution for the control group was 66 (14.3%) with the homozygote B and 396 with the homozygote b (85.7%).
Among the 85 patients, the bb genotype was overexpressed in the PIGD predominant PD patients (94.3%) than in the tremor predominant PD patients (75.6%) (Table 3). The male to female ratio, the age at onset, and the dopa-responsiveness of the patient groups with and without the bb genotype were similar (Table 4).
Most efforts to identify the sequence variations in the VDR gene have focused on the 3' regulatory region because it is close to the anonymous markers used mainly in association studies. Three adjacent restriction fragment length polymorphism (RFLP) for BsmI, ApaI, and TaqI, which are located near the 3' end of the gene, is known to be involved in regulating expression, particularly through the control of mRNA stability (14). Recent studies have suggested a differential luciferase activity for the two 3' UTR-variants, which are linked to the two most frequent haplotypes, "baT" and "BAt" (9), and a tendency for the BAt haplotype to display somewhat higher of mRNA expression levels than baT (15). At another level, the result by analyzing the in vivo responses to treatment demonstrated a trend for the BAt haplotype to display a somewhat better response than baT (16, 17). Although the influences of this linkage disequilibrium on the VDR protein function and signaling is largely unknown, it is tempting to speculate that individuals with the BAt haplotype have higher numbers of VDR in the target cells, which confer these target cells a better response to vitamin D.
In view of what has been discussed above, a BsmI polymorphism in intron 8 of the VDR gene can be an intriguing candidate for PD. This study is the first to show an association between the VDR gene polymorphisms and PD. This study found that the bb genotype was more common in those PIGD predominant PD patients compared with the tremor predominant PD patients and the controls.
It was suggested that PD is not a homogenous entity, but is divisible into two subtypes (a tremor predominant group and a PIGD-predominant group) according to the clinical features as assessed by the components of the UPDRS (18). The PIGD-dominant group had poorer responsiveness to dopaminergic replacement therapy, a rapid progression of motor disabilities and a poor prognosis compared to the tremor-dominant group (13, 18). Therefore, it is possible that different neuronal mechanisms are involved in the development of the major symptoms in PD. In this study, the distribution of BsmI polymorphisms in the tremor predominant group was similar to the control group, whereas in the PIGD-dominant group did not. Although the functional significance of the VDR gene in PIGD predominant PD is still unclear, this study indicates a possible role for the VDR gene in the development of nigrostriatal neuronal loss leading to specific parkinsonian symptoms.
However, care should be taken when interpreting the results of this study because the distribution of the VDR genotype at intron 8 BsmI shows ethnic variations. In this study, the distribution of the VDR genotype in healthy Korean subjects (BB 1.3%, Bb 26%, bb 72.7%) was similar to that in Japanese subjects (BB 3.2%, Bb 25.3%, bb 71.6%) (19). In contrast, Morrison et al. reported the BB genotype in 18.4%, Bb in 52.9%, and bb in 28.7% of healthy controls with Caucasian British-Australian ancestry (20). Suppose that the bb homozygosity for the BsmI genotype is a genetic risk factor for PD, the high frequency of the bb genotype observed in this study may be some contradiction to the lower incidence of PD in Asians (21,22).
It should be noted that no firm pathophysiological conclusions could be drawn from this genetic association study. A possible explanation is that the VDR gene polymorphism is a marker of the linkage disequilibrium with another gene, which is actually responsible for the observed association. Furthermore, generalizing these results might be limited because this study population is small. Since the evidence for an association between PD and the VDR gene polymorphisms is based on a BsmI RFLP alone, the distribution of other polymorphisms in the VDR gene, such as ApaI, TaqI and FokI, will need to be analyzed in order to confirm these results. In addition, the role of a VDR gene polymorphism should be further examined in other populations in order to confirm another susceptibility gene for PD and to determine more adequate strategies for treating PD.
Figures and Tables
Table 2
Distribution of the VDR genotyping in the Parkinson's disease patients and healthy control subjects
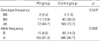
References
1. Gash DM, Zhang Z, Gerhardt G. Neuroprotective and neurorestorative properties of GDNF. Ann Neurol. 1998. 44:S121–S125.


2. Bjorklund A, Rosenblad C, Winkler C, Kirik D. Studies on neuroprotective and regenerative effects of GDNF in a partial lesion model of Parkinson's disease. Neurobiol Dis. 1997. 4:186–200.
3. Sanchez B, Lopez-Martin E, Segura C, Labandeira-Garcia JL, Perez-Fernandez R. 1,25-Dihydroxyvitamin D(3) increases striatal GDNF mRNA and protein expression in adult rats. Brain Res Mol Brain Res. 2002. 108:143–146.


4. Wang JY, Wu JN, Cherng TL, Hoffer BJ, Chen HH, Borlongan CV, Wang Y. Vitamin D3 attenuates 6-hydroxydopamine-induced neurotoxicity in rats. Brain Res. 2001. 904:67–75.
5. Segaert S, Bouillon R. Vitamin D and regulation of gene expression. Curr Opin Clin Nutr Metab Care. 1998. 1:347–354.
6. Stumpf WE, Sar M, Reid FA, Tanaka Y, DeLuca HF. Target cells for 1,25-dihydroxyvitamin D3 in intestinal tract, stomach, kidney, skin, pituitary, and parathyroid. Science. 1979. 206:1188–1190.


7. Stumpf WE, Sar M, Clark SA, DeLuca HF. Brain target sites for 1,25-dihydroxyvitamin D3. Science. 1982. 215:1403–1405.


8. Stumpf WE, Clark SA, O'Brien LP, Reid FA. 1,25(OH)2 vitamin D3 sites of action in spinal cord and sensory ganglion. Anat Embryol (Berl). 1988. 177:307–310.


9. Morrison NA, Qi JC, Tokita A, Kelly PJ, Crofts L, Nguyen TV, Sambrook PN, Eisman JA. Prediction of bone density from vitamin D receptor alleles. Nature. 1994. 367:284–287.


11. Yamamura Y, Sobue I, Ando K, Iida M, Yanagi T. Paralysis agitans of early onset with marked diurnal fluctuation of symptoms. Neurology. 1973. 23:239–244.


12. Merello M, Nouzeilles MI, Arce GP, Leiguarda R. Accuracy of acute levodopa challenge for clinical prediction of sustained long-term levodopa response as a major criterion for idiopathic Parkinson's disease diagnosis. Mov Disord. 2002. 17:795–798.


13. Jankovic J, McDermott M, Carter J, Gauthier S, Goetz C, Golbe L, Huber S, Koller W, Olanow C, Shoulson I. The Parkinson Study Group. Variable expression of Parkinson's disease: a base-line analysis of the DATATOP cohort. Neurology. 1990. 40:1529–1534.
14. Decker CJ, Parker R. Diversity of cytoplasmic functions for the 3' untranslated region of eukaryotic transcripts. Curr Opin Cell Biol. 1995. 7:386–392.


15. Uitterlinden AG, Fang Y, Van Meurs JB, Pols HA, Van Leeuwen JP. Genetics and biology of vitamin D receptor polymorphisms. Gene. 2004. 338:143–156.


16. Krall EA, Parry P, Lichter JB, Dawson-Hughes B. Vitamin D receptor alleles and rates of bone loss: influences of years since menopause and calcium intake. J Bone Miner Res. 1995. 10:978–984.


17. Graafmans WC, Lips P, Ooms ME, van Leeuwen JP, Pols HA, Uitterlinden AG. The effect of vitamin D supplementation on the bone mineral density of the femoral neck is associated with vitamin D receptor genotype. J Bone Miner Res. 1997. 12:1241–1245.


18. Zetusky WJ, Jankovic J, Pirozzolo FJ. The heterogeneity of Parkinson's disease: Clinical and prognostic implications. Neurology. 1985. 35:522–526.


19. Fukazawa T, Yabe I, Kikuchi S, Sasaki H, Hamada T, Miyasaka K, Tashiro K. Association of vitamin D receptor gene polymorphism with multiple sclerosis in Japanese. J Neurol Sci. 1999. 166:47–52.


20. Morrison NA, Yeoman R, Kelly PJ, Eisman JA. Contribution of trans-acting factor alleles to normal physiological variability: vitamin D receptor gene polymorphism and circulating osteocalcin. Proc Natl Acad Sci USA. 1992. 89:6665–6669.


21. Kondo K. Nagatsu T, Harabayashi H, Yoshida M, editors. Epidemiology of Parkinson's disease: interplaying gene and environmental factors. Parkinson's disease: From clinical aspects to molecular basis, key topics in brain research. 1991. New-York: Springer-Verlag;129–138.