Abstract
The authors developed a biodegradable polymer that releases an antibiotic (nalidixic acid) slowly and continuously, for prevention of catheter-induced infection during drainage of cerebrospinal fluid. We investigated the in vitro antibiotic releasing characteristics and bacterial killing effects of the new polymer against E. coli. The novel fluoroquinolone polymer was prepared using diisopropylcarbodiimide, poly (e-caprolactone) diol, and nalidixic acid. FT-IR, mass spectrometry, and elemental analysis proved that the novel antibacterial polymer was prepared successfully without any side products. Negative MS showed that the released drug has a similar molecular weight (M.W.=232, 350) to pure drug (M.W.=232). In high pressure liquid chromatography, the released drug and drug-oligomer showed similar retention times (about 4.5-5 min) in comparison to pure drug (4.5 min). The released nalidixic acid and nalidixic acid derivatives have antibacterial characteristics against E. coli, Staphylococcus aureus, and Salmonella typhi, of more than 3 months duration. This study suggests the possibility of applying this new polymer to manufacture drainage catheters that resist catheter-induced infection, by delivering antibiotics for a longer period of more than 1 month.
Catheter infection in the management of acute hydrocephalus could result in serious consequences such as intellectual deterioration and neurological deficits, including death in severe cases. Infection rates related with external drainage of cerebrospinal fluid (CSF) is reported as high as 4-17.2%, and efforts to reduce catheter-induced infection rates during CSF drainage have included intravenous antibiotics, local antiseptics, or lengthening of the subcutaneous catheter (1-3). All of these efforts during last two decades are still unsuccessful in significantly reducing catheter-induced infection (4-8).
It is very difficult to develop a polymer that releases antibiotics slowly to reduce catheter-induced infection rates, because the antibiotic ratio to catheter is limited to less than a few percent, resulting in the low concentration of releasing antibiotic (9, 10). This is because the released quantity of the antibiotic is variable with time, such as an initial burst, or terminal dumping effect of the antibiotic and also because the polymer usually does not endure heat up to 300℃ during the catheter molding process of the silicon (11, 12). Complexity of development of these antibiotic-releasing polymers was resolved with introduction of a simple coating and impregnation method of the catheter with antibiotics or antiseptics (5, 11-15). Coating of the silicon catheter is relatively simple, but still complex than impregnation of the catheter in methodology and safety (5, 16). The impregnation method is so simple and effective that some clinical trials have already been held and entered the commercial market, for the example, Bactiseal™ of Codman® (14, 16).
The authors of this study developed a basic polymeric structure applicable for a drug-delivery-system that had 1) a high drug composition of more than 10% in drug-polymer weight ratio, 2) easy control of the releasing rate by a minor change of the polymer structure, and 3) release duration of up to more than 6 months. Here we confirm the in vitro releasing characteristics and anti-bacterial activity, and suggest this to be new drug-delivery-system applied to CSF drainage catheter and others.
Authors synthesized a new nalidixic acid polymer using diisopropylcarbodiimide, poly (e-caprolactone) diol, and nalidixic acid. Boiling temperature of the polymer was 350℃, and the white powder in room temperature. Depending on the amount of nalidixic acid, PCL-1250 and PCL-2000 were developed (Fig. 1). The synthetic process is summarized as follows: 1.16 g of nalidixic acid and 0.756 g of diisopropylcarbodiimide was dissolved in 40 mL of methylene chloride and stirred at room temperature for 1 hr. After 1 hr, 10 g of poly (caprolactone) diol (M.W.:1250) was added and stirred at room temperature for more than 24 hr. The solvent was evaporated, and the residue was dissolved in tetrahydrofuran and washed with 1 M NaOH. After drying under reduced pressure, the product was obtained (13). The authors evaluated the released monomeric structure, including nalidixic acid from nalidixic polymer, releasing rate, and anti-bacterial activity.
Mass spectrometry (Agilent 1100 LC/MSD: LC solvent; Acetonitle: Ion polarity; positive: Ion source; APCI) was used to validate the structure of the released drug from the film associated with the cleavage sites in the drug polymer. Mass spectrometry was performed at 1 week, 3 weeks, and 6 weeks after the antibiotic polymer was immersed in 25 mL phosphate buffer saline (PBS; 0.1 M, NaCl; 0.138 M, KCl; 0.0027 M, Fig. 2). High pressure liquid chromatography (HPLC) estimated that releasing components were the polymer only, nalidixic acid, nalidixic polymer 1 week after in pH 7.4 phosphate buffer saline (NaCl 0.138 M, KCl 0.0027 M) and nalidixic polymer in chloroform (Fig. 3).
For the release study, the drug loaded polymer was immersed in 25 mL phosphate buffer saline (phosphate buffer; 0.1 M, NaCl; 0.138 M, KCl; 0.0027 M) containing 0.01 wt % NaN3. They were allowed to stand in thermostated shaking water bath at 37℃, 60 rpm. At each interval, the liquid phase was sampled for analysis, and the solvent in the tube was replaced with an equal volume of fresh buffer medium for the next release test. The amount of the released drug was measured at 330 nm with a UV visible spectrometer, and the cumulative release percent for each sample was calculated (Fig. 4).
The E. coli PI strain was grown in trypticase soy broth (Becton Dickinson Microbiology Systems, Franklin Lakes, NJ, U.S.A.) at 37℃ for 18 hr, and then adjusted to a concentration of 0.5 McFarland units (108 colony-forming units/mL) by using standardized spectrophotometric analysis. A cotton swab was dipped into the bacterial suspension and then rubbed in a streaking fashion across the whole surface of a Mueller-Hinton agar plate (Becton Dickinson Microbiology Systems, Franklin Lakes, NJ, U.S.A.). The 10 µL was taken from 30 mL of normal saline solution in which the drug loaded polymer (10 g) was immersed for a given period (every 2-4 weeks). After sampling, the whole saline solution was replaced with a fresh one, and the drug loaded polymer was immersed till the next sampling. The 10 µL taken from 30 mL of normal saline solution, in which drug loaded polymer (10 g) was immersed for a given period, was diluted four times with normal saline. Each diluted 10 µL was dropped softly onto the center of the agar plate that had been freshly inoculated with E. coli. After 10 µL drop was dried, the agar plate was incubated at 37℃ for 24 hr. The zone of inhibition was assessed by measuring the radius of the clear zone of inhibition on the given dilution ratio. The comparative anti-bacterial activity was calculated on the basis of first 5 days, from the ratio of dilution and the radius of zone of inhibition. Each cumulative antimicrobial activity was calculated from comparative anti-bacterial activities. This assay was performed every 2-4 weeks up to 16 weeks (Fig. 5).
The mass spectrometry showed two high peaks (233.1, 274.0) demonstrating that the pure drug, nalidixic acid, and nalidixic acid salt were the major products of degradation and release. Early minor peaks of 90, 104, 127, 146, 174 might be the degraded products of the drug or oligomer, in which the cleavage sites are other than the ester linkage directly attached nalidixic acid. Several minor peaks larger than nalidixic acid (233) at about 7 days might be the drug loaded oligomer or degraded one (Fig. 3).
HPLC showed a peak at 5 min for nalidixic acid in PBS, and no peak within 30 min for polymer in PBS. It showed various peaks of less than 5 min and multiple overlapped peaks on 30 min for nalidixic acid loaded polymer in chloroform. It also showed a peak of nalidixic acid on 5 min for nalidixic acid loaded polymer in PBS for 7 days (Fig. 4). PCL-1250 and PCL-2000 showed the same results in the mass spectrometry and HPLC.
The cumulative amount of released nalidixic acid from the two drug-loaded polymer of PCL-1250 and PCL-2000 was calculated from the measurement with UV visible spectrometer, and showed 2.1 and 3.3% at 1 week, 3.6% and 5.7% at 2 weeks, 4.9% and 7.5% at 3 weeks, and 5.5% and 8.7% at 4 weeks, respectively (Fig. 5). The drug nalidixic acid was released from the drug-loaded polymer beyond 20% for about 40 days without initial burst effect.
When each anti-bacterial activity of the first 5 days for E. coli, Staphylococcus aureus, and Salmonella typhi was compared comparatively, each cumulative anti-bacterial activity of PCL-2000 for E. coli, Staphylococcus aureus, and Salmonella typhi was 5, 9, 2.4 during next 5 days, 7, 9.5, 3.5 during next 30 days, 15, 17.5, 26.9 during next 20 days, 31, 49.5, 73.5 during next 20 days, 32, 65.5, 78.5 during next 30 days, and 37.7, 88.4, 85.7 during the last 10 days (Fig. 6).
Bacterial infection is often a major complication to the more widespread application of polymeric materials in medical devices (17, 18). A foreign body such as a synthetic biomaterial causes the adhesion of microorganisms (18). The attachment of bacteria to a biomaterial surface has been known as a main factor in the initiation of biomaterial-related infections. Bacterial adhesion leads to their accumulation and production of extracellular polymeric substances. Bacterial growth in extracellular polymeric substances brings to the formation of the adhesive 'biofilms' and isolates the surface from the surrounding environment (19). The biofilms have been considered to diminish the activity of antibacterials, phagocytic cells, humoral antagonists and others (20, 21). Some general etiologic agents associated with biomaterial-related infections include Escherichia coli, Proteus mirabilis, Staphylococcus epidermidis, Enterococcus faecalis and Candida albicans (7, 13, 19, 22).
The bacterial growths with biofilms are able to resist several times of the lethal dose of the antibacterial agent (20, 23). Many approaches have been developed to make a biomaterial surface that has an antibiotic activity to reduce bacterial colonization and the formation of biofilm. Most of these approaches have been based on surface coating with drug or diffusion-limited systems for the delivery of the pharmacophores. Two most popular approaches are the coating of a device, such as a stent or catheter with antibacterial agents via an ionic bonding technique (25), and the impregnation of the silicon or urethane catheter with antibacterial solution via a simple dipping method of physical incorporation of the antibiotics in the polymer matrix (9, 11, 12). Other methods include the use of silver (25), and physicochemical surface modification (26-28). However, major problems in all these approaches were the fast or irregular release and the initial burst of antibacterial agents, leading to an erroneous infection during the contact (13, 15, 29).
Consequently, there has been a demand for a new method to prevent the adhesion of microorganisms. An alternative can be found in using the antimicrobial polymer that contains the antimicrobial agent linked covalently as a monomer into the backbone structure of the macromolecule. Woo and coworkers (10) studied a polyurethane copolymer synthesized using hexane diisocyante, poly (caprolactone) diol, a biodegradable polyester, and ciprofloxacin, a fluoroquinolone antibiotic. Their study showed that the degradable polyurethane, including the fluoroquinolone by covalent linkage, has an ability to destroy microorganism when released by degradation of polymer chains by the macrophage-derived enzyme cholesterol esterase. However, specification of the cleavage sites and releasing speed of the drug in the drug polymer were not easy to control (10, 15).
The authors of this study developed a new polymeric delivery system of slow releasing of drugs that has been never reported. New features of this delivery system might be that the drug is water soluble, but the matrix polymer and oligomers are not soluble in water. Since the drug-bounded oligomers are not soluble in water, the drug can be released only after the bound oligomers are degraded below a certain length. Also, the mutual compatibility between the matrix and the oligomer prevents the initial burst effectively. Practically, we could increase the drug-polymer ratio up to more than 10% very easily, and change the releasing speed of the drug to 10 months by controlling the water solubility (hydrophobicity) of the drug and polymer only, without any initial burst (data not shown). It is suggested that this delivery system could be applied to the drainage catheter and drug-delivery systems, for slow and continuous drug delivery probably such as anticancer agents or hormones.
Figures and Tables
Fig. 2
Synthesis and 3 dimension structure of nalidixic-polymer from nalidixic acid and poly (caprolactone) diol.
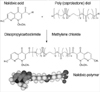
Fig. 3
Mass spectrometric analysis of the released drug. (A) control of nalidixic acid, (B) control of polymer, (C) nalidixic polymer at 0 day in PBS buffer, (D) after 1 weeks in PBS buffer, (E) after 3 weeks in PBS buffer, (F) after 6 weeks in PBS buffer. Two major peaks in (D), (E), and (F) are correspond to the pure drug, nalidixic acid and nalidixic acid salt which are the major products of degradation and release. Minor peaks in (C), (D), (E), and (F) might be the degraded products of the drug bounded oligomer in which the cleavage sites are other than the ester linkage directly attatched
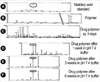
Fig. 4
Analysis with High Pressure Liquid Chromatography for polymer, nalidixic acid, nalidixic acid polymer in chloroform and nalidixic acid polymer after 7 days in PBS buffer pH 7.4.
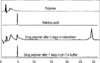
References
1. Holloway KL, Barnes T, Choi S, Bullock R, Marshall LF, Eisenberg HM, Jane JA, Ward JD, Young HF, Marmarou A. Ventriculostomy infections: the effect of monitoring duration and catheter exchange in 584 patients. J Neurosurg. 1996. 85:419–424.


2. Mayhall CG, Archer NH, Lamb VA, Spadora AC, Baggett JW, Ward JD, Narayan RK. Ventriculostomy-related infections. A prospective epidemiologic study. N Engl J Med. 1984. 310:553–559.


3. Stenager E, Gerner-Smidt P, Kock-Jensen C. Ventriculostomy-related infections-an epidemiological study. Acta Neurochir (Wien). 1986. 83:20–23.


4. Friedman WA, Vries JK. Percutaneous tunnel ventriculostomy: Summary of 100 procedures. J Neurosurg. 1980. 53:662–665.
5. Hamilton AJ, Orozco J, Narotam P, Bowersock T. Efficacy of vancomycin/tri-iododecyclemethyl ammonium chloride-coated ventriculostomy catheters in reducing infection. Neurosurgery. 1997. 40:1043–1049.


6. Khanna RK, Rosenblum ML, Rock JP, Malik GM. Prolonged external ventricular drainage with percutaneous long-tunnel ventriculostomies. J Neurosurg. 1995. 83:791–794.


7. Mermel LA. Prevention of intravascular catheter-related infections. Ann Intern Med. 2000. 132:391–402.


8. Mermel LA, Stolz SM, Maki DG. Surface antimicrobial activity of heparin bonded and antiseptic impregnated vascular catheters. J Infect Dis. 1993. 167:920–924.
9. Kwok CS, Wan C, Hendricks S, Bryers JD, Horbett TA, Ratner BD. Design of infection-resistant antibiotic-releasing polymers: I. Fabrication and formulation. J Control Release. 1999. 62:289–299.


10. Woo GL, Mittelman MW, Santerre JP. Synthesis and characterization of a novel biodegradable antimicrobial polymer. Biomaterials. 2000. 21:1235–1246.


11. Bayston R, Milner RD. Antimicrobial activity of silicone rubber used in hydrocephalus shunts after impregnation with antimicrobial substances. J Clin Pathol. 1981. 34:1057–1062.


12. Schierholz J, Jansen B, Jaenicke L, Pulverer G. In-vitro efficacy of an antibiotic releasing silicone ventricle catheter to prevent shunt infection. Biomaterials. 1994. 15:996–1000.


13. Bach A, Bohrer H, Motsch J, Martin E, Geiss HK, Sonntag HG. Prevention of bacterial colonization of intravenous catheters by antiseptic impregnation of polyurethane polymers. J Antimicrob Chemother. 1994. 33:969–978.


14. Darouiche RO, Raad II, Heard SO, Thornby JI, Wenker OC, Garbrielli A, Berg J, Khardori N, Hanna H, Hachem R, Harris RL, Mayhall G. A comparison of two antimicrobial-impregnated central venous catheters. N Engl J Med. 1999. 340:1–8.


15. Jansen B, Jansen S, Peters G, Pulverer G. In vitro effcacy of a central venous catheter ('Hydrocath') loaded with teicoplanin to prevent bacterial colonization. J Hosp Infect. 1992. 22:93–107.
16. Zabramski JM, Whiting D, Darouiche RO, Horner TG, Olson J, Robertson C, Hamilton AJ. Efficacy of antimicrobial-impregnated external ventricular drain catheters: a prospective, randomized, controlled trial. J Neurosurg. 2003. 98:725–730.


17. Buret A, Ward KH, Olson ME, Costerton JW. An in vivo model to study the pathobiology of infectious biofilms on biomaterial surfaces. J Biomed Mater Res. 1991. 25:865–874.
19. Costerton JW, Lewandowski Z, Caldwell D, Korber DR, Lapin-Scott HM. Microbial biofilms. Ann Rev Microbiol. 1995. 49:711–745.


20. Anwar H, Strap JL, Costerton JW. Establishment of aging biofilms: possible mechanism of bacterial resistance to antimicrobial therapy. Antimicrob Agents Chemother. 1992. 36:1347–1351.


21. Gray ED, Peters G, Verstegen M, Regelmann WE. Effect of extracellular slime substance from Staphylococcus epidermidis on the human cellular immune response. Lancet. 1984. 1:365–367.


22. Raad I, Darouiche R, Hachem R, Sacilowski M, Bodey GP. Antibiotics and prevention of microbial colonization of catheters. Antimicrob Agents Chemother. 1995. 39:2397–2400.


23. Arizono T, Oga M, Sugioka Y. Increased resistance of bacteria after adherence to polymethyl methacrylate. An in vitro study. Acta Orthop Scand. 1992. 63:661–664.
24. Sherertz RJ, Forman MD, Solomon DD. Efficacy of dicloxacillin coated polyurethane catheters in preventing subcutaneous Staphylococcus aureus infection in mice. Antimicrob Agents Chemother. 1989. 33:1174–1178.
25. Dasgupta MK. Silver-coated catheters in peritoneal dialysis. Perit Dial Int. 1997. 17:S142–S145.


26. Jansen B, Kohnen W. Prevention of biofilm formation by polymer modification. J Ind Microbiol. 1995. 15:391–396.


27. Levy ML, Luu T, Meltzer HS, Bennett R, Bruce DA. Bacterial adhesion to surfactant-modified silicone surfaces. Neurosurgery. 2004. 54:488–490.


29. Pemberton LB, Ross V, Cuddy P, Kremer H, Fessler T, McGurk E. No difference in catheter sepsis between standard and antiseptic central venous catheters. A prospective randomized trial. Arch Surg. 1996. 131:986–989.