Abstract
Methylprednisolone (MP), a glucocorticoid steroid, has an anti-inflammatory action and seems to inhibit the formation of oxygen free radicals produced during lipid peroxidation in a spinal cord injury (SCI). However, the effects of MP on the functional recovery after a SCI is controversial. The present study was conducted to determine the effects of MP on the recovery of neural conduction following a SCI. A SCI was produced using the NYU spinal cord impactor. A behavioral test was conducted to measure neurological disorders, and motor evoked potentials (MEPs) were recorded. According to the behavioral test, using BBB locomotor scaling, MP-treated animals showed improved functional recoveries when compared to salinetreated animals. MEP latencies in the MP-treated group were shortened when compared to those in the control group. Peak amplitudes of MEPs were larger in the MP-treated group than those in the control group. The thresholds of MEPs tended to be lower in the MP-treated group than those in the control group. These results suggest that MP may improve functional recovery after a SCI.
An acute spinal cord injury (SCI) can produce a neurologic injury with many physiological, psychological, and social ramifications (1). It has been shown that the two separate components, the primary and secondary injuries, were previously combined and were collectively thought to produce neurologic damage in an acute spinal cord injury (2). The primary mediator of a spinal cord injury is the actual mechanical tissue disruption, which is a passive process that occurs immediately after the trauma. A secondary injury cascade follows the initial injury and appears to be mediated by cellular and molecular processes that are working through complex mechanisms (3, 4).
The treatment with high doses of methylprednisolone (MP) is a successful intervention of secondary spinal cord damage (5). A significant improvement in neurological recovery was found in humans with a SCI when a high-dose of MP was administered within 8 hr after the trauma. A MP treatment, that started later than 8 hr after the injury, had no beneficial effects. Thus, it has been proposed that the dose and time of MP administration are important factors in the treatment of an acute SCI (6).
However, conflicting results have also been reported. For example, Ross and Tator (7) and Ross et al. (8) failed to demonstrate the beneficial effects of a high-dose of MP on the posttraumatic spinal cord blood flow and evoked potentials in a rat model of spinal cord injury in the acute stage. Koyanagi and Tator (9) found that none of the doses of MP ranging from 30 to 240 mg/kg improved post-traumatic spinal cord blood flow. In both treatment groups, the somatosensory evoked potentials, the cerebellar evoked potentials, and the posterior fossa evoked potentials completely disappeared after the injury and did not recover (at 3.5 hr). This indicates that a single huge dose of MP had no beneficial effects on the traumatized rat spinal cord in the acute stage.
Therefore, it is not clear whether MP has any beneficial effects on functional recovery after acute SCI. The present study was conducted to observe functional recovery from the behavioral deficits and electrophysiological abnormalities following MP treatment in the spinal cords of rats subjected to a traumatic contusion injury.
Male adult Sprague-Dawley rats (300-350 g) were used in a series of studies. The rats were housed two per cage, exposed to a 12 hr light/dark cycle, and had free access to food and water. Experiments were carried out in accordance with the NIH guidelines from the U.S.A. regarding the care and use of animals for experimental procedures. All of the procedures used in this experiment were approved by the Committee for Animal Experiments in the Yonsei University College of Medicine.
Spinal cord contusion surgeries were performed under pentobarbital sodium (Entovar®, 50-70 mg/kg, i.p.) anesthesia and prophylactic administration of gentamycin sulfate (1 mg/kg). The thoracic area was shaved and prodine and alcohol were applied to the skin. During surgery, the rat's body temperature was maintained at 37℃ using a homeothermic feedback controlled heating pad. An incision was extended from the mid to low thoracic regions. A laminectomy of T9 exposed the spinal cord. For the contusion injuries, spinal clamps were attached to the T7 and T12 spinous processes, a transducer was placed over the transverse process of T10, and the impact rod was centered above T9. The rod was slowly lowered until it contacted the dura, which was determined by the completion of the circuit that activated a tone. The cord was then contused with the NYU weight-drop device.
Immediately after surgery, all rats were maintained in an incubator until thermoregulation was reestablished. Postoperative nursing care included bladder expression two times per day.
After the injury, the rats were randomly assigned to two groups and received an intravenous bolus injection of either MP or physiological saline. The injection volume of the saline and MP was 0.3 mL and the bolus was administered by hand injection for over 1 min. MP (30 mg/kg) or saline was administered through the tail vein 15 min after the SCI.
This study followed the open field training and testing procedures previously described as the BBB locomotor rating scale (10). Fourteen rats were trained preoperatively to move in an open field which was a molded-plastic circular enclosure with a smooth nonslip floor (90 cm diameter; 21 cm wall height). The rats were gently adapted to the open field for a short period of time. Once a rat walked continuously in the open field, two examiners conducted a 5 min, preoperative testing session on each leg using the BBB locomotor rating scale. Postoperative (p.o.) open field testing occurred at least once a week from 1 day p.o. to 8 weeks p.o. for all animals.
Fifty-eight Sprague-Dawely rats were anesthetized with pentobarbital given by an intraperitoneal injection (50 mg/kg). Each animal was also given atropine sulfate (0.8 mg/kg) to reduce tracheal secretions. Using a surgical microscope, the right femoral artery and vein were catheterized and the trachea was intubated. Pancuronium bromide (0.4 mL) was then perfused through the femoral vein to induce muscle relaxation. Each rat was then artificially respired using a small animal respirator (Harvard Apparatus, South Natik, MA, U.S.A.) and expiratory CO2 was maintained within the physiological range using a capnometer (Model 2200, Traverse Medical Monitors, Saline, Michigan, U.S.A.). Each animal was then placed on the stereotaxic device (Narishige Scientific Instrument Laboratory, Setagaya-Ku, Tokyo, Japan) and the rectal temperature was maintained between 36.5-37.5℃ using a feedback controlled heating blanket (Harvard Apparatus, South Natik, MA, U.S.A.).
To stimulate motor cortex, a metal electrode (NE-120, Rhodes Medical Instruments, Inc., distributed by David Kopf Instruments, Tujunga, California, U.S.A.) which was originally designed for recording the sensory evoked potentials in the cerebral cortex was used. The pointed tip of the electrode, with a 0.5 mm long exposed area, was inserted into the cerebral cortex perpendicular to the cortical surface. This enabled gentle contact of the round flat surface with the cortical surface. During cortical stimulation, the round area of the electrode was the anode and the pointed tip was the cathode. This electrode was designed to keep the cathode and the anode close together in order to prevent current spread. In addition, there was also a large enough surface for the activation of a large number of pyramidal neurons with a low intensity electrical current. Furthermore, the direction of the current flow was designed to be the same as the projection of the pyramidal cell bodies. A single square pulse (0.1 msec duration) of electrical stimulus was delivered by a stimulus isolator (A365D or A365, World Precision Instruments, Inc., New Heaven, Connecticut, U.S.A.), which was driven by a pulse generator (Pulsemaster A300, World Precision Instruments, Inc., New Heaven, Connecticut, U.S.A.).
A laminectomy was performed at L1 of the spinal cord of each rat for the placement of the epidural recording electrodes. The shape and specifications of the recording electrodes were the same as those of the stimulating elecrodes. Following the laminectomy, the electrode was inserted into the contralateral gray matter at L1 of the spinal cord. The pointed tip of the electrode was used for activation and the rounded part of the electrode was used as a reference electrode. The analog signals of the evoked potential were amplified (AC amplifier, Model RPS 107, Grass Instrument Co., W. Warwick, RI, U.S.A.), filtered (bandpass 300-1,000 Hz), and averaged on an IBM-compatible personal computer system equipped with Spike 2 software (CED, Cambridge, U.K.). Each MEP consisted of an average of 100-300 single sweep epochs. In order to minimize the effect of the anesthetic on the MEP, recording commenced at least 20 min after the injection of Entobar. The threshold of the electrical stimulation was first determined in each experiment. The effect of the stimulation intensity was analyzed on the wave form and latencies.
Data were expressed as mean±s.e.m. Differences between the MP- and saline-treated groups at each post-operative time point in the behavioral data were analyzed by Student's t-test. Differences between the MP- and saline-treated groups at 2, 4, and 6 weeks p.o. in the electrophysiological data were analyzed by the t-test. Statistical significance was inferred at p<0.05.
All of the animals showed a score of 21 before the SCI. After the SCI, the animals seldom moved their hindlimbs. Fig. 1 shows the changes in locomotor deficits after the SCI. Behavioral scores were measured by BBB methods. Data were expressed as mean±s.e.m. As shown in Fig. 1, BBB scores decreased after the injury but then they slowly and gradually increased. When MP was administered (n=6), locomotor behavior was enhanced when compared to the saline-treated control group (n=8). An asterisk indicates a significant difference between the MP- and saline-treated groups at each post-operative time point (p<0.05).
The MEP was recorded using a special disk electrode in L1 spinal cord after the hindlimb area of the motor cortex was stimulated. When the hindlimb area of the motor cortex was stimulated, a negative-positive-negative wave with a short latency was observed at L1 spinal cord (an upward deflection was designated as negative).
MEPs obtained from the rats after a weight drop injury consisted of only a few peaks above the detection level of 5 µV. On the other hand, MEPs obtained from uninjured normal rats consisted of distinctive peaks with large amplitudes (Fig. 2). After a spinal cord injury, the animals showed lengthened MEP latencies and reduced amplitudes when compared to the normal animals (Fig. 2). Fig. 2 shows representative MEPs recorded in saline-treated SCI rats (upper left), MP-treated SCI rats (lower left), and intact (right) rats. Different amplitude scales were noted. Intraperitoneally injected MP promoted an electrophysiological recovery following the SCI when compared to the saline-treated control animals.
Fig. 3 shows changes in the latencies and amplitudes of MEPs after a MP administration in the SCI animals. Data were expressed as mean±s.e.m. Within the MEPs, the initial latencies (the rising phase of MEP) in the MP-treated group [n=11 (2W); 9 (4W); 8 (6W)] were significantly shortened when compared to the saline-treated control group [n=11 (2W); 8 (4W); 11 (6W)] at 2, 4, and 6 weeks p.o. (p<0.05, Fig. 3A). The latencies of N1 (the first negative deflection) were not significantly different between the MP- and salinetreated groups at 2, 4, and 6 weeks p.o. (p>0.05, Fig. 3B). The latencies of P1 (the first positive deflection) were significantly different between the MP- (n=9) and saline-treated groups (n=8) at 4 weeks p.o. (p<0.05) but not at 2 and 6 weeks p.o. (p>0.05, Fig. 3C).
Fig. 4 shows the changes in the amplitudes of MEPs after the MP administration in the SCI animals. Data were expressed as mean±s.e.m. Within the MEPs, the N1 amplitudes in the MP-treated group (n=11) had significantly increased when compared to the saline-treated control group (n=11) at 2 weeks p.o. (p<0.05, Fig. 4A) but not at 4 and 6 weeks p.o. (p>0.05). Similarly, the P1 amplitudes in the MP-treated group (n=11) had significantly increased when compared to the saline-treated control group (n=11) at 2 weeks p.o. (p<0.05) but not at 4 and 6 weeks p.o. (p>0.05, Fig. 4B).
Although axons in the peripheral nervous system have the capacity to regrow after an injury (11, 12), injured central nervous system axons fail to regenerate (13, 14). Similarly, in the spinal cord, the final sequences of a SCI are related to both the initial physical impact and the numerous secondary injury factors occurring in and around the site of the primary damage over a period of time (days or months).
In the present study, behavioral motor deficits were observed after a SCI. When the MEPs were measured, the latencies of the MEPs were lengthened. These behavioral and electrophysiological deficits may have resulted from the primary physical insult as well as the secondary injury.
A common treatment for a SCI in human beings is the administration of MP (5, 6). Several studies have shown that MP recovers post-traumatic functional deficits in experimental SCIs using a weight drop in different species such as the rat (15), cat (16, 17), and monkey (18). However, some studies have reported that MP is not effective for improving functional recovery after different injury models such as the weight drop (19, 20), clip compression (8, 21, 22), and heat injury with a radiofrequency current (23), in different species such as the dog (19), cat (20) and rat (8, 19, 21-23). In the present study, we observed behavioral recovery after a MP injection using a weight drop model using the BBB test.
The effect of MP on evoked potentials after an experimental SCI has been examined by several studies. Two studies (24, 25) have reported significant recovery of the somatosensory evoked potentials (SSEPs) by MP, while others have shown no improvement (7-9). Koyanagi and Tator (9) observed that MP did not improve the SSEP, reflecting mainly the dorsal column pathways. Furthermore, Ross and Tator (7) monitored MEPs in clip compression-injured rats, but the MEPs had not improved following an MP treatment. In the present study, the latencies of MEPs were shortened and the amplitudes were increased after an MP injection. Contrast to N1 or P1 latencies which were variable from stimulation to stimulation, the initial latencies were measured constantly. The initial latencies of MEPs were shortened after an MP injection at 2, 4, and 6 weeks p.o. On the contrary, we could not observe any improvement in SSEPs (unpublished data). The reason why MP improved MEP but not SSEP is not clear at present. Most of ascending pathways including dorsal column might be injured too severely to be regenerated by contusion injury but descending pathways might be affected less to be regenerated. However, these electrophysiological findings correspond to a behavioral phenomenon. Therefore, MP was effective in both the behavioral and electrophysiological functional recovery after a SCI.
One mechanism that explains the action of MP, is the binding of the glucocorticoid receptor, which mediates its anti-inflammatory properties. MP inhibits the inflammatory processes induced after a SCI (26). MP also inhibits phospholipase A2 activity (27), which reduces production of the metabolites of the arachidonic acid cascade (28) thereby reducing the formation of free radicals (27, 28). MP also reduces the expression of the tumor necrosis factor by decreasing the activation of NF-κB, thereby diminishing the intensity and duration of the inflammatory response (29). Whereas these effects are achieved with the administration of MP at a dose of 0.03 mg/kg (30), to be clinically effective after a SCI, MP must be administered at the large dose of 30 mg/kg (31). At this dose, MP exhibits antioxidant effects, which allow it to scavenge free radicals, thus decreasing the oxygen radical-induced lipid peroxidation of cell membranes (32). According to Nash et al. (33), MP was found to promote axonal regeneration and improve functional recovery after a rat SCI when compared to untreated rats. According to a recent report (34), however, MP may depress the production of growth-promoting factors following acute SCI. Therefore, MP is regarded to have multiple mechanisms for improving functional recovery after a SCI.
At present, many therapies to promote neuronal regeneration after a SCI have been investigated, including the injection of chemicals like MP, surgery, cell therapy, etc. However, it does not appear that any single therapy will solve all of the problems associated with the lack of regeneration after a SCI. It would be helpful to treat a SCI with a combination of two or more effective therapies such as brain-derived neurotrophic factor and MP (35).
Figures and Tables
Fig. 1
Changes in locomotor deficits after a spinal cord injury. Behavioral scores were measured by the BBB methods. The BBB scores decrease after an injury and slowly and gradually increase. Intraperitoneally injected MP promoted a behavioral recovery following a SCI when compared to the saline-treated control animals. Data were expressed as mean±s.e.m. Asterisks (*) indicate a significant difference between the MP- and saline-treated groups at each post-operative time point (p<0.05).
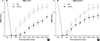
Fig. 2
Representative motor evoked potentials (MEPs) recorded in saline-treated SCI (upper left), MP-treated SCI (lower left), and intact (right) animals. Note the different amplitude scales.
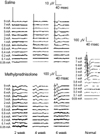
Fig. 3
Changes in latencies of MEPs after MP administration in the SCI animals. (A) Comparison of the initial latencies (the rising phase of MEPs) in the MP- and saline-treated groups at 2, 4, and 6 weeks p.o. (B) Comparison of the N1 (the first negative deflection) latencies in the MP- and saline-treated groups at 2, 4, and 6 weeks p.o. (C) Comparison of the P1 (the first positive deflection) latencies in the MP- and saline-treated groups at 2, 4, and 6 weeks p.o. Data were expressed as mean±s.e.m. Asterisks (*) indicate a significant difference between the MP- and saline-treated groups at each post-operative time point (p<0.05).
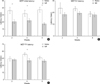
Fig. 4
Changes in amplitudes of MEPs after the MP administration in the SCI animals. (A) Comparison of the N1 (the first negative deflection) amplitudes in the MP- and saline-treated groups at 2, 4, and 6 weeks p.o. (B) Comparison of the P1 (the first positive deflection) amplitudes in the MP- and saline-treated groups at 2, 4, and 6 weeks p.o. Data were expressed as mean±s.e.m. Asterisks (*) indicate a significant difference between the MP- and saline-treated groups at each post-operative time point (p<0.05).
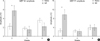
References
1. Collins WF, Piepmeir J, Ogle E. The spinal cord injury problem: A review. Central Nerv Syst Trauma. 1986. 3:317–331.
2. Anderson TE, Stokes BT. Experimental models for spinal cord injury research: Physical and Physiological considerations. J Neurotrauma. 1992. 9:S135–S142.
3. O'Brien MF, Lenke LG, Lou J, Bridwell KH, Joyce ME. Astrocyte response and transforming growth factor-β localization in acute spinal cord injury. Spine. 1994. 19:2321–2330.
4. Tator CH, Fehlings MG. Review of the secondary injury theory of acute spinal cord trauma with emphasis on vascular mechanisms. J Neurosurg. 1991. 75:15–26.


5. Bracken MB, Shepard MJ, Collins WF, Holford TR, Young W, Baskin DS, Eisenberg HM, Flamm E, Leo-Summers L, Maroon J, Marshall LF, Perot PL Jr, Piepmeier J, Sonntag VK, Wagner FC, Wilberger JE, Winn HR. A randomized, controlled trial of methylprednisolone or naloxone in the treatment of acute spinal-cord injury: Results of the Second National Acute Spinal Cord Injury Study. N Engl J Med. 1990. 322:1405–1411.
7. Ross IB, Tator CH. Spinal cord blood flow and evoked potential responses after treatment with nimodipine or methylprednisolone in spinal cord injured rats. Neurosurgery. 1993. 33:470–477.
8. Ross IB, Tator CH, Theriault E. Effect of nimodipine or methylprednisolone on recovery from acute experimental spinal cord injury in rats. Surg Neurol. 1993. 40:461–470.


9. Koyanagi I, Tator CH. Effect of a single huge dose of methylprednisolone on blood flow, evoked potentials, and histology after acute spinal cord injury in the rat. Neurol Res. 1997. 19:289–299.


10. Basso DM, Beattie MS, Bresnahan JC. A sensitive and reliable locomotor rating scale for open field testing in rats. J Neurotrauma. 1995. 12:1–21.


11. Fawcett JW, Keynes RJ. Peripheral nerve regeneration. Annu Rev Neurosci. 1990. 13:43–60.
12. Raivich G, Kreutzberg GW. Peripheral nerve regeneration: role of growth factors and their receptors. Int J Dev Neurosci. 1993. 11:311–324.


13. Schwab ME, Bartholdi D. Degeneration and regeneration of axons in the lesioned spinal cord. Physiol Rev. 1996. 76:319–370.


14. Nicholls JG, Adams WB, Eugenin J, Geiser R, Lepre M, Luque JM, Wintzer M. Why does the central nervous system not regenerate after injury? Surv Ophthalmol. 1999. 43:S136–S141.


15. Holtz A, Nystrom B, Gerdin B. Effect of methylprednisolone of motor function and spinal cord blood flow after spinal cord compression in rats. Acta Neurol Scand. 1990. 82:68–73.
16. Campbell JB, DeCrescito V, Tomasula JJ, Demopoulos HB, Flamm ES, Ransohoff J. Experimental treatment of spinal cord contusion in the cat. Surg Neurol. 1973. 1:102–106.
17. Braughler JM, Hall EX, Means ED, Waters TR, Anderson DK. Evaluation of an intensive methylprednisolone sodium succinate dosing regimen in experimental spinal cord injury. J Neurogurg. 1987. 67:102–105.


18. Green BA, Kahn T, Klose KJ. A comparative study of steroid therapy in acute experimental spinal cord injury. Surg Neurol. 1980. 13:91–97.
19. Hedeman LS, Sil R. Studies in experimental spinal cord trauma. Part 2: Comparison of treatment with steroids, low molecular weight dextran, and catecholamine blockade. J Neurosurg. 1974. 40:44–51.
20. Faden AI, Jacobs TP, Patrick DH, Smith MT. Megadose corticosteroid therapy following experimental traumatic spinal injury. J Neurosurg. 1984. 60:712–717.


21. Iizuka H, Iwasaki Y, Yamamoto T, Kadoya S. Morphometric assessment of drug effects in experimental spinal cord injury. J Neurosurg. 1986. 65:92–98.


22. Kelly DF, Bernstein JJ, Laws ER. Methylprednisolone: Therapeutic time window in experimental spinal cord injury in rats. Surg Forum. 1991. 42:512–514.
23. Iwai A, Monafo WW, Elliasson SG. Methylprednisolone treatment of exerimental spinal cord injury. Paraplegia. 1993. 31:417–429.
24. Young W, Flamm ES. Effect of high-dose corticosteroid therapy on blood flow, evoked potentials, and extracellular calcium in experimental spinal injury. J Neurosurg. 1982. 57:667–673.


25. De Ley G, Leybaert L. Effect of flunarizine and methylprednisolone on functional recovery after experimental spinal injury. J Neurotrauma. 1993. 10:25–35.


26. Hsu CY, Dimitrijevic MR. Methylprednisolone in spinal cord injury: the possible mechanism of action. J Neurotrauma. 1990. 7:115–119.


27. Hirata F, Schiffmann E, Venkatasubramanian K, Salomon D, Axelrod J. A phospholipase A2 inhibitory protein in rabbit neutrophils induced by glucocorticoids. Proc Natl Acad Sci USA. 1980. 77:2533–2536.


28. Becker J, Grasso RJ. Suppression of phagocytosis by dexamethasone in macrophage cultures: inability of arachidonic acid, indomethacin, and nordihydroguaiaretic acid to reverse the inhibitory response mediated by a steroid-inducible factor. Int J Immunopharmacol. 1985. 7:839–847.


29. Xu J, Fan G, Chen S, Wu Y, Xu XM, Hsu CY. Methylprednisolone inhibition of TNF-alpha expression and NF-kB activation after spinal cord injury in rats. Mol Brain Res. 1998. 59:135–142.
30. Young W, DeCrescito V, Flamm ES, Blight AR, Gruner JA. Pharmacological therapy of acute spinal cord injury: studies of high dose methylprednisolone and naloxone. Clin Neurosurg. 1988. 34:675–697.
31. Hall ED, Wolf DL, Braughler JM. Effects of a single large dose of methylprednisolone sodium succinate on experimental posttraumatic spinal cord ischemia. Dose-response and time-action analysis. J Neurosurg. 1984. 61:124–130.
32. Demopoulos HB, Flamm ES, Pietronigro DD, Seligman ML. The free radical pathology and the microcirculation in the major central nervous system disorders. Acta Physiol Scand Suppl. 1980. 492:91–119.
33. Nash HH, Borke RC, Anders JJ. Ensheathing cells and methylprednisolone promote axonal regeneration and functional recovery in the lesioned adult rat spinal cord. J Neurosci. 2002. 22:7111–7120.

