Abstract
Apoptosis is a programmed, physiologic mode of cell death that plays an important role in tissue homeostasis. As for the central nervous system, ischemic insults can induce pathophysiologic cascade of apoptosis in neurophils. Impairment of astroctye functions during brain ischemia can critically influence neuron survival by neuronglia interactions. We aimed to elucidate the protective effect of ketamine on apoptosis by energy deprivation in astrocytes. Ischemic insults was induced with iodoacetate/carbonylcyanide m-chlorophenylhydrazone (IAA/CCCP) 1.5 mM/20 µM or 150 µM/2 µM for 1 hr in the HTB-15 and CRL-1690 astrocytoma cells. Then these cells were reperfused with normal media or ketamine (0.1 mM) containing media for 1 hr or 24 hr. FITC-annexin-V staining and propidium iodide binding were determined by using flow cytometry. Cell size and granularity were measured by forward and side light scattering properties of flow cytometry system, respectively. An addition of ketamine during reperfusion increased the proportion of viable cells. Ketamine alleviated cell shrinkage and increased granularity during the early period, and ameliorated cell swelling during the late reperfusion period. Ketamine may have a valuable effect on amelioration of early and late apoptosis in the astrocytoma cells, even though the exact mechanism remains to be verified.
Cellular injury can trigger a variety of responses, such as adaptation, repair, proliferation and cell death. Apoptosis is a programmed cell death that has been implicated in the regulation of tissue homeostasis, and the pathophysiologic process of neurologic disorders, such as Alzheimer's disease, Parkinson's disease, Huntington' disease, amyotrophic lateral sclerosis, and ischemic insults (1, 2).
It is generally believed that the mechanism of apoptotic cell death is different from necrosis. By electron microscopic analysis, apoptotic cell death demonstrates specific morphological changes including cell shrinkage, chromatin condensation, DNA fragmentation, membrane blebbing, apoptotic body formation, while necrosis is consistent with cell swelling and destruction of cell morphology with the release of intracellular organelles. During the early phase of apoptosis, phosphatidylserine (PS), which is normally almost totally confined to the inner layer of phospholipid plasma membrane is moved to the outer leaflet of the plasma membrane by the decreased ATP-dependent translocase (flippase) activity and the increased calcium-dependent scramblase activity while maintaining the plasma membrane integrity (2-4). Fluorescein isothiocyanate (FITC)-conjugated annexin-V, which is a Ca2+ dependent phospholipid binding protein, is used as a reliable marker for PS exposure (3, 5, 6). During the late phase of apoptosis, a shift from tightly to loosely packing of the plasma membrane phospholipids with PS externalization allows propidium iodide (PI) penetration into the cell and binding with DNA. Double staining of cells with FITC-annexin-V and PI in association with flow cytometry has been proven to be a valuable method to discriminate between early and late phase of apoptosis. Changes in morphology of the apoptotic cell can also be monitored by flow cytometry as a change in light-scattering properties, forward scattering (FSC) and side scattering (SSC) (5, 7).
Central nervous system (CNS) neurons are extremely sensitive to metabolic changes and physio-chemical injuries. Restoration of neuronal viability after apoptotic or necrotic cell death has been suggested to be impossible, therefore, it is hoped that more focused therapies to prevent apoptotic cell death could be developed. Many clinicians are reluctant to use ketamine in head injury patients in the clinical setting due to an increase in the cerebral metabolic rate, cerebral blood flow, and intracranial pressure. However, the protective effect of ketamine in the amelioration of ischemic insults of CNS has been reported in several animal and cellular experiments. Ketamine was reported to suppress excessive excitatory amino acid release, attenuate the development of focal brain edema, and ameliorate neurological dysfunction after neuronal injury in animal experiments (8-12).
Recent studies have revealed that glial cells are regarded to play many important roles, such as regulating extracellular concentrations of ions, metabolites, and neurotransmitters, and participated in synaptic functions with neurons in CNS. Therefore, damage of the most abundant glial cells, astrocytes, can bring about crucial influence on the neuronal survival (13-15). It is now well known that signals between astroglial cells and neurons go back and forth to modulate synaptic activity (16-18).
Here, under in vitro conditions, we investigated the effect of ketamine and reperfusion on the apoptosis of the astrocytoma cells following energy depletion with glycolysis inhibitor iodoacetate (IAA) and blocker of oxidative phosphorylation carbonylcyanide m-chlorophenylhydrazone (CCCP), by flow cytometric analysis.
Human astrocytoma cell line HTB-15 and CRL-1690 cells were cultivated in T-tube flask. Dulbecco's modified minimal essential medium (DMEM) for HTB-15 cells and DMEM with 1.5 g/L sodium bicarbonate, 1.0 mM sodium pyruvate was used for CRL-1690 cells. The medium was supplemented with 10% fetal calf serum and 100 IU penicillin G and 50 µg/mL streptomycin. The cells were incubated in a 5% CO2 chamber at a humidified atmosphere. Once the cells were grown confluent to the flask, the cells were harvested for the experiment with 0.1% trypsin-EDTA in a phosphate buffer solution (PBS) and washed twice thereafter. The addition of DMEM blocked the trypsin activity, and then suspension cells were transferred to the other T-tube. For the flow cytometric analysis, 2-3 mL of cell suspension were again transferred to the cuvette. In order to control the metabolic rate of experimental cells carefully, harvested cells only in the 3-5 passages were used.
To mimic the ischemic damage in vitro, cells were treated with IAA/CCCP 1.5 mM/20 µM or 150 µM/2 µM for 1 hr. And then, to observe reperfusion-induced cellular damage or the effect of ketamine during the reperfusion, experimental protocols called for cells to be exposed to normal media or ketamine (0.1 mM or 0.5 mM)-containing media for 1 hr or 24 hr after wash out of agonists. Also, to investigate the effect of ketamine itself on the PI binding, ketamine 0.1 mM or 0.5 mM was added to the perfusion media without ischemic insult.
For the measurement of surface exposure of PS and plasma membrane leakage of experimental cells with flow cytometry system, 105 cells were incubated with FITC-annexin-V (1 µg/mL) for 15 min and PI (2 µg/mL) for 2 min in a dark area at room temperature, and then washed with calcium-containing phosphate buffered solution to remove excess fluorescent dyes. We used commercially available FITC-annexin-V and PI (Trevigen Inc, Gaithersburg, MD, U.S.A.), and those were diluted to 1:50, 1:10 with calcium buffer solution, respectively, in this experiment.
All experiments were performed using a FACStar Plus Flow Cytometer (Becton Dickinson, Sunnyvale, CA, U.S.A.) equipped with a single argon ion laser exciting at 488 nm. FITC-annexin-V fluorescence was collected through a 530 nm band pass filter while PI fluorescence was measured through 639 nm band pass filter (19). It was designed specifically to detect cellular changes of less than 1%. Prior to every experiment, the flow cytometry was calibrated electrically and mechanically by latex beads with already known diameter. Quantitative analysis of cell viability of 104 cells was performed in 20 sec. The mean value of cellular changes was obtained by the computer analysis of cytogram. The X, Y-axis indicated the fluorescence of PI and annexin-V, respectively. By the gate of the 4 areas, it was possible to detect and quantitatively compare normal cells (annexin-V-/PI-), early apoptosis (annexin-V+/PI-), late apoptosis or necrosis (annexin-V+/PI+) in the whole cell population.
Alterations of cellular characteristics were also analyzed by the degree and direction of light scattering through laser beam of flow cytometry. FSC determines cell volume, SSC for cellular granularity, density and folding. Decrease in FSC and increase in SSC indicative of cell shrinkage and increased cell granularity, respectively, indicate early phase of apoptosis. Increase in FSC and decrease in SSC are associated with late phase of apoptosis. Therefore, flow cytometry was also utilized to assess viable cells, early or late phase of apoptosis according to cell volume and degree of cellular granularity. In order to analyze better morphological changes with treatment, we expressed cell volume and granularity as % of those of the control cells without any treatment.
Experimental data were expressed as mean±SD. To compare between the two groups the unpaired t-test was performed. p value less than 0.05 was considered statistically significant. Jandel Sigma Stat (version 2.0, Jandel Corporation, Chicago, IL, U.S.A.) was used for statistical analysis.
Annexin-V binding and PI uptake according to the concentrations of IAA/CCCP were observed in CRL-1690 cells. The portion of annexin-V positive cells and PI positive cells increased from 4.9% and 0.8% in control cells to 8.8% and 1.9% in cells treated with IAA/CCCP 150 µM/2 µM for 1 hr, respectively (Fig. 1A). In a different condition, annexin-V positivity and PI positivity changed from 12.3% and 3.8% to 23.7% and 3.1%, respectively after treatment with IAA/CCCP 1.5 mM/20 µM (Fig. 1B). These results demonstrated the relationship between intracellular energy depletion and PS redistribution.
The administration of ketamine 0.1 mM during the reperfusion induced an increase in the portion of vital cells (annexin-V and PI negative cells) from 90.6% to 93.6% (Fig. 2A), and from 76.1% to 81.2%, respectively, after the insult with IAA/CCCP 150 µM/2 µM, and 1.5 mM/20 µM (Fig. 2B). These showed that ketamine plays a potential role in the inhibition of PS redistribution during intracellular energy depletion.
Only cellular debris was found following treatment with a higher concentration of IAA/CCCP for 1 hr and reperfusion for 24 hr. The experimental results after treatment with a low concentration of IAA/CCCP, 150 µM/2 µM, for 1 hr and reperfusion for 24 hr exhibited similar patterns of responses as with other experiments. The viable cells decreased from 91.7% to 81.5% after reperfusion, but the administration of ketamine 0.1 mM during the reperfusion caused an increase in the viable cells to 85.1% (Fig. 3A). In the other experiments with a viability of 85.5%, reperfusion left only 19.9% of cells viable. The addition of ketamine in this condition also saved cell viability of up to 33.7% (Fig. 3B). Based on these results, we found that the addition of ketamine during the reperfusion protected cells from the progression of apoptosis in any condition.
Fig. 4 clearly showed the effect of ketamine 0.1 mM on the change of cellular volume and granularity following IAA/CCCP (150 µM/2 µM) treatment in HTB-15 cells. The addition of ketamine during the reperfusion ameliorated the decrease in. Compared to the control condition, cell volume decreased to 71, 68, 68%, while cell granularity increased to 123, 131, 139%, respectively, during the reperfusion for 1 hr after energy depletion in HTB-15 cells. These cellular changes were indicative of apoptosis. An addition of ketamine 0.1 mM during the reperfusion reduced cell shrinkage to 72, 69, 79% of control cells and increased granularity to 113, 127, 132% of control cells, respectively. CRL-1690 cells also showed similar effect of ketamine on the cellular changes after energy depletion (Table 1).
During reperfusion for 24 hr after being exposed to IAA/CCCP 150 µM/2 µM for 1 hr, however, both HTB-15 and CRL-1690 cells showed cell swelling, rather than cell shrinkage. This indicated morphological changes from the early phase of apoptosis to late stage of apoptosis. The addition of ketamine during the reperfusion protected cells from swelling. However, the changes of cellular granularity was not consistent, thereby, the effect of ketamine on the cellular granularity after reperfusion for 24 hr was not observed (Table 2).
This experiment was performed to investigate the effect of ketamine on the PI binding during the reperfusion for 24 hr following IAA/CCCP treatment in CRL-1690 cells. PI positive cells increased 7.4% in control cells to 80.8% in cells treated with IAA/CCCP 150 µM/2 µM. The addition of ketamine at both concentrations of 0.1 mM and 0.5 mM during the reperfusion significantly decreased PI binding to 73.1% and 65.2%, respectively. PI positive cells with ketamine 0.1 mM or 0.5 mM were 6.6% and 8.4%, respectively, which showed no difference with the control data. Therefore, we found that ketamine protected cells from increased plasma membrane permeability due to energy depletion, rather than affect normal cell membrane integrity (Table 3).
Ischemia-induced pathologic processes in the CNS are one of the leading causes of primary mortality and morbidity. ATP is required for a number of vital neuronal processes and energy failure evokes numerous pathologic events including apoptosis. Many previous studies reported that astrocyte function could critically influence neuronal survival during ischemia. Therefore, we mimicked ischemic condition in vitro by using IAA/CCCP for depleting intracellular energy. In this study, we demonstrated the evidence of ketamine effect on the reduction of apoptosis in astrocytoma cells by using flow cytometry system.
In this experiment, we used Annexin-V and PI as indicators for apoptosis. Recently Yu et al. (20) demonstrated that the mechanism of astroglial cell death due to ischemic insults is apoptosis by using annexin-V staining. Annexin-V cannot penetrate the double layer of cell membrane and interact with PS. PS mostly faces the cytosol by outside-inside PS translocase, using ATP, in the normal cells. Having damages to the cells including hypoxia or ischemia, internally located PS moves over the outer leaflet of the plasma membrane; hence allowing the binding of annexin-V (21).
Annexin-V assay with flow cytometry for assessing apoptosis is simple and useful. The observation with electron microscopic analysis to discriminate between apoptosis and necrosis is elaborate, time consuming, and cumbersome for quantitative analysis (5, 6). Although terminal deoxynucleotidyl transferase (TdT)-mediated dUTP-biotin nick-end labeling (TUNEL) analysis shows a high level of specificity, experimental method is complicated and requires 3-4 hr to complete (22). Flow cytometry using annexin-V fluorescence allows rapid quantitative measurement in 5-10 min, and is relatively easy because it does not require enzyme or fixation (23). In addition, DNA fragmentation is not an early event in apoptosis, and not always associated with apoptosis. Apoptosis is a programmed, physiologic mode of cell death, which is characterized by structural nuclear changes, but also relatively by preserved plasma membrane and organelle components. Recently the externalization of PS has been demonstrated in the absence of nuclear damage (24). This indicates that a cytoplasmic apoptotic regulator may be responsible for apoptosis in the early phase. Castedo et al. (25) suggested that the redistribution of PS was not affected by increased intracellular calcium ion or reactive oxygen species (ROS), but disruption of mitochondrial membrane potential. When lymphocytes are exposed to CCCP, breakdown of mitochondrial potential was demonstrated in a few minutes, followed by PS exposure on the outer membrane layer in 60 min.
The addition of pharmacological agents which stabilize mitochondrial membrane potential or inhibit disruption of mitochondrial potential, protected PS exposure (26). Therefore, aberrant PS movement may be a secondary effect subsequent to the disruption of mitochondrial membrane potential. Moreover, the functional impact of mitochondrial respiratory chain may be significant during the reperfusion period than during the ischemic insult, by the rapid ionic movement through the damaged membrane (27). Changes of mitochondrial membrane potential are irreversible and appear in several cells in the early event of apoptosis, without exception. Less than 5% of annexin-V positive cells contain normal nuclear morphology. Loss of plasma membrane asymmetry by the cell surface exposure of PS precedes loss of membrane integrity, increased membrane permeability and nuclear condensation (3, 5, 24, 28).
Annexin-V is commonly used to analyze apoptotic cell death in suspending cells. But, approximately 1-5% of cells in culture medium show apoptosis (5). Trypsinization to detach adherent cells from the culture plate may cause membrane damage by handling and following annexin-V staining. In the human lung carcinoma cell line MR-65, approximately 25% of the suspension cells showed annexin-V binding and a lack of PI uptake after trypsin treatment (5). About 10% of control cells were positive for PI in PC-12 cell line (4). In this experiment, the portion of the HTB-15 and CRL-1690 cells showing both annexin-V and PI negativity after trypsinization ranged from 85 to 95%. Therefore these cells were appropriate for performing experiment on the apoptotic process.
Apoptosis has been described to occur under several stimulations. We found that in this experiment, the degree of redistribution of PS depended on the concentration of IAA/CCCP: higher concentration of IAA/CCCP is associated with increased annexin-V and PI uptake during the reperfusion (Fig. 1). The addition of ketamine 0.1 mM during the reperfusion increased viable cells with both annexin-V and PI negative staining, compared to reperfusion without ketamine (Fig. 2, 3). This finding strongly suggests that ketamine was able to restore intracellular energy, maintain membrane integrity by the inhibition of phospholipid membrane peroxidation, consequently protect cells from PS redistribution and PI entry intracellulary. Zhou et al. (29) suggested that the addition of ketamine 0.1-10 mM inhibited chemical- or ischemia-induced lipid peroxidation and ischemic glutathione depletion.
For mimicking energy depletion we used CCCP in this experiment. As an uncoupler of mitochondrial respiratory chain, CCCP decreases mitochondrial membrane potential, causes alterations of energy metabolism, produces membrane peroxidation and disrupt membrane integrity due to structural and functional alterations (25, 26). Table 1, 2 represents the results concerning the changes of cell volume and granularity due to intracellular energy depletion, by using forward and side light scattering on flow cytometry. Reperfusion for 1 hr causes cell shrinkage and increased granularity, but in the late apoptotic phase a progressive water influx causes cell swelling and increased the release of intracellular organelles, which can be related to decreased granularity. It is evident that the progression of early apoptosis to secondary necrotic cell death is dependent on the intensity of the stimulus and the energy status of the cell (30, 31). CsA-induced apoptosis in the renal proximal tubular cell line, LLC-PK1 showed increased FITC-annexin-V affinity and cell granularity, and decreased cell volume with a low dose, while decreased membrane integrity and cell granularity, and increased cell volume in higher concentration (19). In the apoptosis of HL-60 cells induced by UV exposure for 30 min, morphological change showing cellular swelling, the phenomenon of secondary cellular necrosis, was observed after 6 hr under the electronic microscopy (1). As time passes, the entry of PI was increased in fresh cells without stimuli, although cell morphology was well preserved under electronic microscopy (32).
We found that ketamine addition during the reperfusion for 1 hr ameliorated morphological changes of apoptosis, such as cellular shrinkage and increased cellular granularity (Table 1), and ketamine addition during the reperfusion for 24 hr also ameliorated cellular necrosis accompanying cellular swelling (Table 2). Chen and Simard (33) also demonstrated that hypoxia-ischemia and ATP depletion are associated with glial swelling and blebbing. Ketamine 0.1 mM itself was found not to induce apoptosis by the observation that annexin-V affinity or PI uptake was not increased. Thus, ketamine was effective in two types of cell death, both early stage of apoptosis and necrosis.
As a non-competitive NMDA receptor blocker (34), ketamine produced considerable neuroprotection after mechanical trauma or ischemic injury. Until very recently, NMDA receptor was demonstrated only in the neuronal cell membrane, however, Krebs et al. revealed the expression of functional NMDA receptor in astrocytes under the ischemic insults by immunohistochemistry (35). Ketamine may become a valuable agent in restoring vulnerable astrocytes (36). The potential neuroprotective effect of ketamine was proved at the cellular level (9, 32). Many studies revealed that ketamine stabilized neuronal and glial electrophysiological functions by regulating Ca2+, Na+, K+ conductance, thus maintaining intracellular ion homeostasis (32, 37-39). Administration of ketamine before anoxic-hypoxic insults preserved neuronal action potential in the hippocampus and protected cellular energy status, maintaining two-thirds of ATP production (9, 10). Related to experiments mimicking ischemic insult or head trauma in rats, ketamine improved neurological outcomes by inhibiting pathophysiologic NO production as a potential blocker for NMDA receptor (11, 32), decreasing catecholamine level (12) and ameliorating brain swelling (8).
Using harvested cells, which have similar characteristics of primary cells, is relatively easy in vitro experiment. We used astroglioma cells instead of primary astrocytes in the current study. Recently, the capacity of the CNS has been revealed in many studies. As the most abundant cell type in the central nervous system, astrocytes play very important and diverse roles, interacting with neurons. Astrocytes provide structural, trophic, and metabolic support to neurons and coordinate with neurons in neurotransmission. A large body of studies has demonstrated on the relationship between neurons and astrocytes survival. The addition of astrocyte-conditioned medium in the serum free medium allows neurons to be attached to the bottom of the coverslip and neuritis outgrowth, whereas in the absence of astrocyte-conditioned medium impairment of neuronal survival was shown (17). In the condition of coculture of neurons and astrocytes, removal of astrocytes caused neuronal apoptosis (16). To prevent hypoxia-ischemia induced neuronal degeneration, astrocytes survival is crucial (37).
In conclusion, we have demonstrated the occurrence of early and late phase of apoptosis during the reperfusion after intracellular energy depletion in astrocytoma cells by using flow cytometry system. Ketamine addition during the reperfusion protected astrocytoma cells from annexin-V binding and PI uptake, and changes of cell volume and granularity. Considering the intimate functional and structural interaction of astrocyte with neuron in the CNS, the presence of astrocyte is very important for neuronal survival.
Figures and Tables
Fig. 1
Bivariate PI/annexin-V analysis of the CRL-1690 cells during the reperfusion for 1 hr after IAA/CCCP treatment. Quadrant % gated in this assay identify the different cell populations, i.e. region UL: PI-positive/ annexin-V-negative, UR: PI-positive/ annexin-V-positive, LL: PI-negative/annexin-V-negative, LR: PI-negative/ annexin-V-positive. (A) and (B) indicate the effect of different concentrations of IAA/CCCP (150 µM/2 µM vs. 1.5 mM/20 µM) on the cell apoptosis.
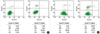
Fig. 2
The effect of ketamine on the apoptosis of the CRL-1690 cells during the reperfusion for 1 hr Ketamine 0.1 mM addition during the reperfusion increased cell viability after both low (A) and high concentration (B) IAA/CCCP treatment.
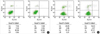
Fig. 3
Bivariate PI/annexin V analysis of the CRL-1690 cells during the reperfusion for 24 hr. A, B, C indicate cells in normal media for 24 hr, in reperfusion for 24 hr after IAA/CCCP (150 µM/2 µM) treatment for 1 hr and in reperfusion with ketamine 0.1 mM containing media after IAA/CCCP treatment, respectively. (A) and (B) are examples of many experiments.
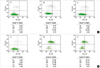
Fig. 4
The effect of ketamine on the changes of size and granularity of the HTB-15 cells. Forward light scattering properties measured the size of cells in the normal media for 1 hr (1), in reperfusion for 1 hr after IAA/CCCP (150 µM/2 µM) treatment for 1 hr (2), and in reperfusion with ketamine 0.1 mM containing media for 1 hr after IAA/CCCP treatment (3), respectively (A). Side light scattering properties measured the granularity of cells (B) in the normal media for 1 hr (1), in reperfusion for 1 hr after IAA/CCCP (150 µM/2 µM) treatment for 1 hr (2), and in reperfusion with ketamine 0.1 mM containing media for 1 hr after IAA/CCCP treatment (3).
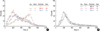
Table 1
The esffect of ketamine on the changes of FSC and SSC during reperfusion for 1 hr after IAA/CCCP treatment
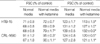
References
1. Reno F, Burattini S, Rossi S, Luchetti F, Columbaro M, Santi S, Papa S, Falcieri E. Phospholipid rearrangement of apoptotic membrane does not depend on nuclear activity. Histochem Cell Biol. 1998. 110:467–476.
2. Walton M, Sirimanne E, Reutelingsperger C, Williams C, Gluckman P, Dragunow M. Annexin V labels apoptotic neurons following hypoxia-ischemia. Neuroreport. 1997. 8:3871–3875.


3. Martin SJ, Reutelingsperger CP, McGahon AJ, Rader JA, van Schie RC, LaFace DM, Green DR. Early redistribution of plasma membrane phosphatidylserine is a general feature of apoptosis regardless of the initiating stimulus: inhibition by overexpression of Bcl-2 and Abl. J Exp Med. 1995. 182:1545–1556.


4. Rimon G, Bazenet CE, Philpott KL, Rubin LL. Increased surface phosphatidylserine is an early marker of neuronal apoptosis. J Neurosci Res. 1997. 48:563–570.


5. van Engeland M, Ramaekers FC, Schutte B, Reutelingsperger CP. A novel assay to measure loss of plasma membrane asymmetry during apoptosis of adherent cells in culture. Cytometry. 1996. 24:131–139.


6. Bose S, Tuunainen I, Parry M, Medina OP, Mancini G, Kinnunen PK. Binding of cationic liposomes to apoptotic cells. Anal Biochem. 2004. 331:385–394.


7. Telford WG, King LE, Fraker PJ. Rapid quantitation of apoptosis in pure and heterogeneous cell populations using flow cytometry. J Immunol Methods. 1994. 172:1–16.


8. Shapira Y, Lam AM, Eng CC, Laohapraist V, Michel M. Therapeutic time window and response of the beneficial effects of ketamine in experimental head injury. Stroke. 1994. 25:1637–1643.
9. Rothman SM, Thurston JH, Hauhart RE, Clark GD, Solomon JS. Ketamine protects hippocampal neurons from anoxia in vitro. Neuroscience. 1987. 21:673–678.


10. Pophprecki R, Becker GL, Reilly PJ, Landers DF. Ketamine, MK-801 or calmidazolium protects rat hippocampal energy status during in vitro ischemia. Ann NY Acad Sci. 1991. 625:818–820.


11. Lin SZ, Chiou AL, Wang Y. Ketamine antagonizes nitric oxide release from cerebral cortex after middle cerebral artery ligation in rats. Stroke. 1996. 27:747–752.


12. Hoffman WE, Peligrino D, Werner C, Kochs E, Albrecht RF, Schulte am Esch J. Ketamine decreases plasma catecholamines and improves outcome from incomplete cerebral ischemia in rats. Anesthesiology. 1992. 76:755–762.


14. Aschner M, Sonnewald U, Tan KH. Astrocyte modulation of neurotoxic injury. Brain Pathol. 2002. 12:475–481.


15. Tamatani M, Ogawa S, Niitsu Y, Tohyama M. Involvement of Bcl-2 family and caspase-3 like protease in NO-mediated neuronal apoptosis. J Neurochem. 1998. 71:1588–1596.
16. Ohgoh M, Kimura M, Ogura H, Katayama K, Nishizawa Y. Apoptotic cell death of cultured cerebral cortical neurons induced by withdrawal of astroglial trophic support. Exp Neurol. 1998. 149:51–63.


17. Wang XF, Cynader MS. Effects of astrocytes on neuronal attachment and survival shown in a serum-free co-culture system. Brain Res Protoc. 1999. 4:209–216.


18. Araque A, Carmignoto G, Haydon PG. Dynamic signaling between astrocytes and neurons. Annu Rev Physiol. 2001. 63:795–813.


19. Healy E, Dempsey M, Lally C, Ryan MP. Apoptosis and necrosis: mechanisms of cell death induced by cyclosporine A in a renal proximal tubular cell line. Kidney Int. 1998. 54:1955–1966.


20. Yu AC, Wong HK, Yung HW, Lau LT. Ischemia-induced apoptosis in primary cultures of astrocytes. Glia. 2001. 35:121–130.


21. Gummadi SN, Hrafnsdottir S, Walent J, Watkins WE, Menon AK. Reconstitution and assay of biogenic membrane-derived phospholipid flippase activity in proteoliposomes. Methods Mol Biol. 2003. 228:271–279.


22. Gavrieli Y, Sherman Y, Ben-Sasson SA. Identification of programmed cell death in-situ via specific labeling of nuclear DNA fragmentation. J Cell Biol. 1992. 119:493–501.
23. Zhang G, Gurtu V, Kain SR, Yan G. Early detection of apoptosis using a fluorescent conjugate of annexin-V. Biotechniques. 1997. 23:525–531.
24. Chan A, Reiter R, Wiese S, Fertig G, Gold R. Plasma membrane phospholipid asymmetry precedes DNA fragmentation in different apoptotic cell models. Histochem Cell Biol. 1998. 110:553–558.


25. Castedo M, Hirsch T, Susin SA, Zamzami N, Marchetti P, Macho A, Kroemer G. Sequential acquisition of mitochondrial and plasma membrane alterations during early lymphocyte apoptosis. J Immunol. 1996. 157:512–521.
26. van Engeland M, Nieland LJ, Ramaekers FC, Schutte B, Reutelingsperger CP. Annexin V-affinity assay: a review on an apoptosis detection system based on phosphatidylserine exposure. Cytometry. 1998. 31:1–9.


27. Smis NR, Anderson MF. Mitochondrial contributions to tissue damage in stroke. Neurochem Int. 2002. 40:511–526.
28. Wolvetang EJ, Johnson KL, Krauer K, Ralph SJ, Linnane AW. Mitochondrial respiratory chain inhibitors induce apoptosis. FEBS Lett. 1994. 339:40–44.


29. Zhou M, Ma T, Tseng MT. Effects of taurine and ketamine on bovine retinal membrane lipid peroxidation. Neuroscience. 1991. 45:461–465.


30. Bonfoco E, Kraine D, Ankarerona M, Nicotera P, Lipon SA. Apoptosis and necrosis: two distinct events induced, respectively, by mild and intense insults with N-methyl-D-aspartate or nitric oxide/superoxide in cortical cell cultures. Proc Natl Acad Sci USA. 1995. 92:7162–7166.


31. Leist M, Single B, Castoldi AF, Kuhnle S, Nicotera P. Intracellular adenosine triphosphate (ATP) concentration: A switch in the decision between apoptosis and necrosis. J Exp Med. 1997. 185:1481–1486.


32. Pfenninger E, Himmelseher S. Neuroprotection by ketamine at the cellular level. Anaesthesist. 1997. 46:S47–S54.
33. Chen M, Simard JM. Cell swelling and a nonselective cation channel regulated by internal Ca2+ and ATP in native reactive astrocytes from adult rat brain. J Neurosci. 2001. 21:6512–6521.
34. Kohrs R, Durieux ME. Ketamine: teaching an old drug new tricks. Anesth Analg. 1998. 87:1186–1193.
35. Krebs C, Fernandes HB, Sheldon C, Raymond LA, Baimbridge KG. Functional NMDA receptor subtype 2B is expressed in astrocytes after ischemia in vivo and anoxia in vitro. J Neurosci. 2003. 23:3364–3372.
36. Kim MH, Hahm TS, Ahn HJ. Regulation of astroglial volume by ketamine in glutamate induced cellular volume changes. Korean J Anesthesiol. 1997. 33:1005–1011.


37. Chan PH, Chu L. Ketamine protects cultured astrocytes from glutamate-induced swelling. Brain Res. 1989. 487:380–383.


38. Brau ME, Sander F, Vogel W, Hempelmann G. Blocking mechanisms of ketamine and its enantiomers in enzymatically demyelinated peripheral nerve as revealed by single-channel experiments. Anesthesiology. 1997. 86:394–404.


39. Silver IA, Erecinska M. Intracellular and extracellular changes of Ca2+ in hypoxia and ischemia in rat brain in vivo. J Gen Physiol. 1990. 95:837–866.