Abstract
While ica gene of Staphylococcus epidermidis is known to undergo phase variation by insertion of IS256, the phenomenon in Staphylococcus aureus has not been evaluated. Six biofilm-positive strains were tested for the presence of biofilm-negative phase-variant strains by Congo red agar test. For potential phase-variant strains, pulsed-field gel electrophoresis was done to exclude the possibility of contamination. To investigate the mechanism of the biofilm-negative phase variation, PCR for each ica genes were done. Changes of ica genes detected by PCR were confirmed by southern hybridization, and their nucleotides were analyzed by DNA sequencing. Influence of ica genes and biofilm formation on capacity for adherence to biomedical material was evaluated by comparing the ability of adhering to polyurethane surface among a biofilm-negative phase-variant strain and its parent strain. A biofilm-negative phase-variant S. aureus strain was detected from 6 strains tested. icaC gene of the phase-variant strain was found to be inactivated by insertion of additional gene segment, IS256. The biofilm-negative phase-variant strain showed lower adhering capacity to polyurethane than its parent strain. This study shows that phase variation of ica gene occurs in S. aureus by insertion of IS256 also, and this biofilm-negative phase variation reduces adhering capacity of the bacteria.
As biomaterials are used commonly in medical practice, the incidence of infections related to biomaterials is increasing. To prevent biomaterial-related infections, it is essential to understand the pathogenesis of the infection.
Biofilm is known to play a key role in the adherence of many pathogenic bacteria to biomaterials. In coagulase negative staphylococci (CNS), the most common pathogen of biomaterial-related infections, the later phase of biofilm formation is mediated by polysaccharide intercellular adhesin (1-5), a product of the intercellular adhesion (ica) operon (3, 6).
It is also known that ica gene of CNS is subject to phase variation, which is a process of ON↔OFF in the production of biofilm (7, 8). The phase variation of CNS in biofilm formation is believed to be the mechanism of its long persistence and relapse. Although the whole mechanism of this phase variation have not been understood, some of the phase variation in CNS were reported to occur due to the inactivation of ica operon by insertion of the insertion sequence element IS256 (8).
Staphylococcus aureus is another common pathogen of biomaterial-related infections, and biofilm formation is also known to be associated with its virulence. Recently, Cramton et al. reported that S. aureus also possessed ica genes (9). Deletion of ica genes in S. aureus resulted in the loss of ability to form biofilm.
The presence of phase variation in biofilm formation of S. aureus has been reported before (10). However, the mechanisms of the phenomenon, which might be associated with some changes in ica gene, have not been investigated yet.
Furthermore, although the influence of ica gene of S. aureus on the biofilm formation has been evaluated, studies about the impact of changes in ica gene and subsequent biofilm formation on the adhering capacity to biomaterials have not been performed yet.
Therefore, this study was carried out to investigate the presence and mechanism of phase variation in ica gene of S. aureus, and to evaluate the relationship between ica gene and the adhering capacity to biomaterials in S. aureus using phase-variant strains.
We screened 38 clinical strains and 44 nasal carriage strains of S. aureus for ica operon and biofilm formation. ica operon was detected by PCR. Primers used to amplify the ica operon are presented in Table 1. Biofilm formation was evaluated by biofilm assay using microtiter-plate (9) and Congo red agar method (11).
Six biofilm-positive strains, which had ica operon and showed consistent results in both of the biofilm formation tests, were tested for selection of biofilm-negative phase-variant strains. Screening for isolation of biofilm-negative variants was performed on Congo red agar. Biofilm-positive strains grow on this medium as dark-red colonies, whereas biofilm-negative strains grow on as light-red colonies. Agar plates were incubated overnight at 37℃ and an additional 24 hr at room temperature. The variation rate was determined by counting the different colony forms in relation to the total number of colony-forming unit (CFU).
A single colony of a biofilm-negative variant was isolated and incubated at 37℃ in tryptic soy broth in a tissue culture flask. After 24 hr the medium was replaced. This procedure was repeated until a biofilm of adhering bacteria became visible on the bottom of the tissue culture flask (maximum of 15 days). After washing with phosphate-buffered saline (PBS), the adhering bacteria cells were scratched from the bottom and streaked on Congo red agar. After incubation at 37℃ overnight and an additional 24 hr at room temperature, single, dark-red colony was isolated.
DNA of biofilm-negative phase-variants, biofilm-positive revertants and their parent strains were compared by pulsed-field gel electrophoresis (PFGE). PFGE was performed as described (12).
Genes of icaA, icaB, and icaC were amplified by PCR, and compared with those of the parent and revertant strains. Primers and the amplification conditions of the ica-specific PCR are summarized in Table 1.
Changes in ica genes found by PCR were confirmed with Southern blot hybridization and DNA sequencing. Chromosomal DNA was isolated from S. aureus wild-type, biofilm-negative variants, and biofilm-positive revertant and digested with EcoRI. The digested DNA was separated on 0.8% agarose gel electrophoresis, and then transferred to a Hybond-N+ Nylon transfer membrane (Amersham Life Science, Buckinghamshire, U.K.). After the membrane was backed at 80℃ for 2 hr, prehybridization and hybridization were conducted at 42℃ for 1 hr and overnight, respectively. Southern blot DNA-DNA hybridization was carried out under high-stringency condition by using icaC-specific probe. The probe DNA was labeled by using the ECL direct nucleic acid labelling system (Amersham). Washing the membrane with 1×SSC containing 0.4% sodium dodecyl sulphate was reacted with detection reagent (Amersham) and then exposed to radiography film.
The nucleotide sequence of PCR products was determined by using dideoxy chain termination method. A template was mixed Terminator Ready Reaction Mix (Perkin Elmer Cetus, Norwalk, U.S.A.), which already contained primers, and incubated in thermal at 96℃ (30 sec), 50℃ (15 sec), and 60℃ (4 min). Typically, 25 cycles are required for extensive amplification (i.e., 106 fold) of a specific target. After being denatured by heating for 2 min at 90℃, the samples were set ABI377 automated DNA sequencer (PE Applied Biosystems, Foster city, CA, U.S.A.).
The assay for the quantitative determination of biofilm formation was performed with polyurethane (PU) films. 1.0×1.0 cm square form of PU films were sterilized with ethylene oxide gas. Bacterial cultures in Tryptic soy broth, containing 107CFU/mL of SA 204 wild-type strain and phase variant, respectively, incubated with 100 rpm agitation at 37℃ with 4 sheets of PU films. After 5 days, PU films were washed three times with 4 mL of PBS. Remaining bacteria on PU films were resuspended by vigorous vortexing and 0.1 mL of a diluted culture was seeded per blood agar plate. After incubation for 2 days at 37℃, the number of colonies was determined.
From a strain among the 6 strains tested, potential biofilm-negative subcolonies were grown at a frequency of 1×10-9-5×10-9. Two strains (SA204/v-5, SA204/v-6) from the subcolonies showed biofilm negative on biofilm assay. From one strain among them, a biofilm-positive revertant was obtained after 3-4 passages. Congo red agar test and biofilm assay of the strains are compared with those of their parent strain in Fig. 1 and Fig. 2, Biofilm forming strains, SA204 and SA204/r-6, grow on Congo red agar medium as dark-red color, and show red color on microtiter plate. Whereas biofilm-negative phase-variant strains, SA204/v-5 and SA204/v-6, grow on Congo red agar medium as light-red color.
SA204/v-5 and SA204/v-6 showed the same PFGE patterns with that of the parent strain and the revertant strain (Fig. 3). While PCR products of icaA and icaB genes of the variant strains were the same in size with those of the parent strain and the revertant strain, icaC-specific PCR products showed different sized bands (Fig. 4). Southern hybridization analysis with the icaC-specific DNA probe showed a larger restriction fragment also (Fig. 5). The increased size of the shifted icaC gene was about 1.3 kb, which was the same in size with that of the IS256. Nucleotide sequence of the enlarged PCR fragments perfectly matched the published sequence of the insertion sequence element IS256.
The adhesion capacity to PU film of SA204/v-5 and its parent strain showed a significant difference statistically (104.8 vs. 107, p<0.02).
In S. epidermidis, phase variation of biofilm formation is caused by alternating insertion and excision of the insertion sequence element IS256 into different sites of the ica gene cluster (7, 8). Specifically, 11 out of 13 insertion mutants carry the element in the icaC gene, one mutant was an icaA and another one an icaB insertional mutant (8). In this study, we obtained two phase-variant strains that are insertional mutants the inactivation of icaC gene by IS256. These investigations appear to suggest that the icaC nucleotide sequence seems to represent a preferred insertion site of IS256.
As far as we know, this is the first report about the role of IS256 in the mechanism of phase variation in biofilm formation of S. aureus. We did not evaluate the prevalence of phase variant strains, nor the proportion of IS256 contributing to the phase variation of ica gene in S. aureus. To figure out the frequency of phase variation in biofilm and insertion of IS256, studies with more strains are warranted.
Due to the fact that a biofilm-variant strain had a low capacity of adhering to PU films, we could conclude that ica gene and biofilm play a role in the adherence of S. aureus to biofilm materials. We used phase-variant strains to compare the adherence ability to PU films between biofilm-forming and biofilm-nonforming S. aureus strains, on the premise that the phase-variant S. aureus strains had changed only in ica genes. It was not feasible to evaluate all other virulence factors in phase-variant strains. To exclude the potential influence of other virulence factors, studies using mutant strains with inactivated ica genes specifically are warranted. Moreover, considering the influence of host components in adherence of S. aureus to biomaterials, in vivo studies using animal models are also warranted.
Figures and Tables
Fig. 1
Congo red agar tests of 2 biofilm-negative phase variants (SA204/v-5, SA204/v-6), the revertant strain (SA204/r-6), and their parent strain (SA204). Biofilm forming strains show dark-red color, whereas biofilm-ngative strains show light-red color. Left upper quadrant, SA204; right upper quadrant, SA204/v-5; left lower quadrant, SA204/v-6; right lower quadrant, SA204/r-6.
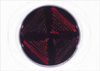
Fig. 2
Biofilm assay of 2 biofilm-negative phase variants (SA204/v-5, SA204/v-6), the revertant strain (SA204/r-6), and their parent strain (SA204). Biofilm forming strains show red color. From left; SA204, SA204/v-5, SA204/v-6, and SA204/r-6.
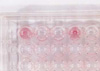
Fig. 3
Pulse field gel electrophoresis (PFGE) analysis of biofilm-negative phase-variant strains, compared to parent strain and the revertant strain. Chromosomal DNA fragments, generated by SmaI digestion, were analyzed by PFGE. Lane 1 refers to Lambda ladder PFGE marker. All strains show same PFGE pattern. WT, SA204; V5, SA204/v-5; V6, A204/v-6; R5, SA204/r-6.
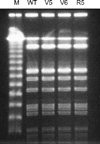
Fig. 4
PCR amplification of icaC gene in biofilm-negative phase-variant strains, compared to parent strain and the revertant strain. Lane 1 refers to Lambda/HindIII marker. Biofilm-negative phase-variant strains show shifted icaC-specific PCR product, the increased size of which is about 1.3 kb. WT, SA204; V5, SA204/v-5; V6, A204/v-6; R5, SA204/r-6.
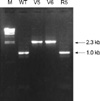
Fig. 5
Southern blot with icaC-specific DNA probe in EcoRI-digested chromosomal DNA of biofilm-negative phase-variant strains, compared to parent strain and the revertant strain. Biofilm-negative phase-variant strains show shifted icaC-specific PCR product of increased size. WT, SA204; V5, SA204/v-5; V6, A204/v-6; R5, SA204/r-6.
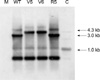
References
1. Heilmann C, Gerke C, Perdreau-Remington F, Götz F. Characterization of Tn917 insertion mutants of Staphylococcus epidermidis affected in biofilm formation. Infect Immun. 1996. 64:277–282.


2. Heilmann C, Hussain M, Peters G, Götz F. Evidence for autolysin-mediated primary attachment of Staphylococcus epidermidis to a polystyrene surface. Mol Microbiol. 1997. 24:1013–1024.
3. Heilmann C, Schweitzer O, Gerke C, Vanittanakom N, Mack D, Götz F. Molecular basis of intercellular adhesion in the biofilm-forming Staphylococcus epidermidis. Mol Microbiol. 1996. 20:1083–1091.


4. Mack D, Fischer W, Krokotsch A, Leopold K, Hartmann R, Egge H, Laufs R. The intercellular adhesin involved in biofilm accumulation of Staphylococcus epidermidis is a linear β-1,6-linked glucosaminoglycan: purification and structural analysis. J Bacteriol. 1996. 178:175–183.
5. Mack D, Nedelmann M, Krokotsch A, Schwarzkopf A, Heesemann J, Laufs R. Characterization of transposon mutants of biofilm-producing Staphylococcus epidermidis impaired in the accumulative phase polysaccharide intercellular adhesin. Infect Immun. 1994. 62:3244–3253.
6. Gerke C, Kraft A, Süssmuth R, Schweitzer O, Götz F. Characterization of the N-acetylglucosaminyl-transferase activity involved in the biosynthesis of the Staphylococcus epidermidis polysaccharide intercellular adhesin. J Biol Chem. 1998. 273:18586–18593.
7. Christensen GD, Baddour LM, Simpson WA. Phenotypic variation of Staphylococcus epidermidis slime production in vitro and in vivo. Infect Immun. 1987. 55:2870–2877.


8. Ziebuhr W, Krimmer V, Rachid S, Lötzssner I, Götz F, Hacker J. A novel mechanism of phase variation of virulence in Staphylococcus epidermidis : evidence for control of the polysaccharide intercellular adhesin synthesis by alternating insertion and excision of the insertion sequence element IS 256. Mol Microbiol. 1999. 32:345–356.
9. Cramton SE, Gerke C, Schnell NF, Nichols WW, Götz F. The intercellular adhesion (ica) locus is present in Staphylococcus aureus and is required for biofilm formation. Infect Immun. 1999. 67:5427–5433.


10. Baselga R, Albizu I, De La Cruz M, Del Cacho E, Barberan M, Amorena B. Phase variation of slime production in Staphylococcus aureus: implications in colonization and virulence. Infect Immun. 1993. 61:4857–4862.

